ABSTRACT
Current human papillomavirus (HPV) vaccines provide substantial protection against the most common HPV types responsible for oral and anogenital cancers, but many circulating cancer-causing types remain for which vaccine coverage is lacking. In addition, all current HPV vaccines rely on aluminum salt-based adjuvant formulations that function through unclear mechanisms with few substitutes available. In an effort to expand the toolbox of available adjuvants suitable for HPV vaccines, we compared the immunogenicity of the RG1-VLP (virus-like particle) vaccine in BALB/c mice when formulated with either the aluminum hydroxide adjuvant Alhydrogel or the novel polyphosphazene macromolecular adjuvant poly[di (carboxylatoethylphenoxy) phosphazene] (PCEP). PCEP-formulated RG1-VLPs routinely outperformed VLP/Alhydrogel in several measurements of VLP-specific humoral immunity, including consistent improvements in the magnitude of antibody (Ab) responses to both HPV16-L1 and the L2 RG1 epitope as well as neutralizing titers to HPV16 and cross-neutralization of pseudovirion (PsV) types HPV18 and HPV39. Dose-sparing studies indicated that RG1-VLPs could be reduced in dose by 75% and the presence of PCEP ensured activity comparable to a full VLP dose adjuvanted by Alhydrogel. In addition, levels of HPV16-L1 and -L2-specific Abs were achieved after two vaccinations with PCEP as adjuvant that were equivalent to or greater than levels achieved with three vaccinations with Alhydrogel alone, indicating that the presence of PCEP resulted in accelerated immune responses that could allow for a decreased dose schedule. Given the extensive clinical track record of polyphosphazenes, these data suggest that substitution of alum-based adjuvants with PCEP for the RG1-VLP vaccine could lead to rapid seropositivity requiring fewer boosts, the dose-sparing of commercial VLP-based vaccines, and the establishment of longer-lasting humoral responses to HPV.
Introduction
Broad and consistent HPV vaccination programs have achieved a great degree of success lowering rates of the most common HPV genotypes that cause oral and anogenital cancers. Current vaccines are based on noninfectious VLPs produced by expression of the major L1 capsid protein and containing no viral material. The FDA initially licensed bivalent and quadrivalent versions of these vaccines which succeeded in reducing infection by HPV16/18 types that are responsible for 70–80% of HPV-related cervical intraepithelial neoplasia (CIN). The most recently FDA-approved nonavalent vaccine, Gardasil-9, only extends protection to 7 of the 13 high risk HPV types that together cause 90% of HPV-positive cancers. However, in the absence of comprehensive coverage of high-risk HPV types, the need for continued cervical screening cannot be eliminated.Citation1 Further addition of new L1-VLPs derived from different HPV genotypes would continue to increase manufacturing cost-of-goods (COG) with only small incremental improvements in protection coverage over the general population. Here, we examine a single candidate vaccine antigen in which a conserved protective epitope is displayed on the surface of an L1 VLP as an approach to extend coverage to all high-risk HPV genotypes.
HPV infection occurs through viral engagement of heparin sulfate proteoglycans and laminin on the surface of basal keratinocytes, leading to conformational changes which expose the minor capsid protein L2 and permit its cleavage by the host-derived convertase furin. L2 demonstrates substantial sequence conservation among high-risk HPVs, and antisera specific to linear epitopes in the N-terminal HPV16-L2 region have demonstrated cross-neutralization activity directed against multiple HPV types,Citation2,Citation3 a phenomenon not achievable with the L1 capsid subunit.Citation4–6 In particular, the L2 amino acid 17–36 epitope has a high degree of conservancy between types and is named RG1 due to recognition by the RG1 mAb.Citation7 Passive transfer of sera from RG1-VLP-vaccinated mice confers immunity from experimental PsV challenge, suggesting a central role of neutralizing antibody in mediating protection.Citation7 Vaccine configurations aimed at enhancing the immunogenicity of the protective L2 epitopes have included linear L2 epitope repeats on a modified human IgG1 Fc scaffold,Citation8 a concatenated fusion protein adjuvanted with Montanide ISA51,Citation9 or as adenovirus- or AAV vector-expressed L2 epitopesCitation10,Citation11 (reviewed inCitation12,Citation13). These L2 vaccines have demonstrated cross-neutralizing Ab responses against HPV of diverse types including HPV5/6/11/16/18/31/33/45/52/58,Citation8,Citation11 underlining the cross-neutralization potential of L2. To take advantage of the naturally immunogenic nature of the closed-packed, repetitive surface L2 array while retaining the well-established protective potency of the L1 subunit, a VLP vaccine was engineered to display the RG1 HPV16-L2 epitope inserted within the DE loop of each of 360 HPV16-L1 subunits that spontaneously assemble into a VLP.Citation14 This ‘RG1-VLP’ vaccine, when adjuvanted with aluminum salts, has demonstrated robust immunity in mice and the induction of cross-neutralization to many mucosal high-risk HPV types, including HPV18 and HPV39, mediated by L2-specific Abs.Citation15 However, all currently licensed prophylactic HPV vaccines are adjuvanted with aluminum salts, in part because few other effective adjuvants with good safety profiles are available.
Polyphosphazenes (PP) comprise a family of synthetic organic-inorganic polymers which have demonstrated promise for use as adjuvant vehicles in both preclinical and clinical studies. The common synthetic precursor can give rise to a diverse group of macromolecules exhibiting advantageous properties such as biodegradability, water solubility, self-assembly, and the ability to increase vaccine antigen valency. Two macromolecules, poly[di(carboxylatophenoxy)phosphazene] (PCPP) and poly[di(carboxylatoethylphenoxy)phosphazene (PCEP), have demonstrated their immunoadjuvant potency with several viral and bacterial antigens in multiple animal models.Citation16–18 These water-soluble dual-mode adjuvants attain their biological activity via self-assembly with vaccine antigens as delivery vehicles as well as by intrinsic immunostimulatory activity. Research conducted with these polymers has been focused on soluble antigenic proteins and although PCPP has demonstrated potency when combined with rotavirus VLPs,Citation19 this earlier study did not include PCEP and lacked characterization of formulation in context of antigen–polymer interactions, which usually play an important role in defining immunological performance of these adjuvants.Citation20–23The goal of the current study was to test the immunogenicity of RG1-VLPs formulated with PCPP or PCEP compounds in BALB/c mice, in an attempt to broaden the choice of adjuvant that could be paired with a VLP-based HPV vaccine and demonstrate equivalent or superior efficacy compared to the standard FDA-approved adjuvant, aluminum salts.
Materials and methods
Polyphosphazenes
PCPP and PCEP, both with weight-average molecular weight of 800 kDa, were synthesized as described previously.Citation24,Citation25
Biophysical characterization of formulations
Analysis of PCPP or PCEP-VLP formulations was conducted by dynamic light scattering (DLS) using Malvern ZetaSizer Nano series (Malvern Instruments Ltd.) instrument and Asymmetric Flow Field Flow Fractionation (AF4) with Postnova AF2000 MT instrument (Postnova Analytics GmbH). pH-dependent membrane disruptive activity of polyphosphazene (PP)/VLP vaccine formulations was evaluated using a hemolysis test with fresh porcine RBCs as described previously.Citation20 Porcine red blood cells (RBC) – 10% suspension in PBS (Innovative Technology Inc., Novi, MI) were used as received.
Vaccine preparation
RG1-VLPs (lot PB16076) were manufactured by Paragon Bioservices (under contract with MRIGlobal, Repository Contractor for the National Cancer Institute, Division of Cancer Prevention) and were combined with Alhydrogel at 40 µg/ml RG1-VLPs and 1 mg/ml Alhydrogel and incubated on a rocking platform for 1 h at 4°C to yield a vaccine of 2 µg RG1-VLPs and 50 µg Alhydrogel per 50 µl per mouse. PCEP and PCPP at 2 mg/ml solutions were first depleted of aggregates and sterilized by syringe filtration through a 0.2 µm filter (Millex) before combining with RG1-VLPs and vortexed to yield 2 µg RG1-VLPs and 7.5–50.0 µg PP in 50 µl per injection. Gardasil-9™ (Merck, recombinant 9-valent human papillomavirus vaccine) was used as a positive control comparator and was dose-normalized to 2 µg HPV16-L1 VLPs per injection. After equilibration to room temperature (r.t.), vaccines were immediately administered to mice.
In vivo mouse vaccination studies
Eight to ten-week-old female BALB/c mice (Charles River or Jackson Laboratories) were randomized into groups of 8–10 animals and immunized on days 0, 14, and 28 (2-week intervals) or 0, 21, 42 (3-week intervals). Mice were anesthetized with isoflurane before intramuscular (i.m.) injection of RG1-VLPs combined with Alhydrogel, PCEP, or PCPP into the quadriceps muscle with 50 µl dose volumes. In some cases, submandibular bleeds were performed on days 13 or 14 and 27 or 28, and terminal bleeds were conducted via cardiac puncture on isoflurane-anesthetized mice at day 42 or day 56. In order to measure longer-term Ab responses, some mice were not terminated on day 42 but were monitored with submandibular bleeds at days 42, 70, 98, and 125, followed by termination. No systemic adverse events or injection site reactions were observed. Bleeds were collected in serum separator tubes (Fisher Scientific) at r.t. and centrifuged at 6000 g for 1.5 min. Cell-free sera were collected and stored at −80°C.
HPV16-L1 VLP and HPV16-L2 RG1 peptide ELISAs
Mouse sera were subjected to quantitation by enzyme-linked immunosorbent assay (ELISA) of Abs specific to HPV16-L1 VLPs and to the HPV16-L2 RG1 epitope. For HPV16-L1 Ab quantitation, Maxisorp 96-well plates (Thomas Scientific) were coated with lab-produced HPV16-L1 VLPs at 2.7 µg/ml in coating buffer (1X PBS + 0.2% Proclin 300 (Sigma)) and used within 3–5 days after incubation at 4°C. For HPV16-L2 RG1 epitope-specific Ab quantitation, NUNC streptavidin-coated 96-well plates (Thermo-Fisher) were coated with 250 ng/ml N-terminal-biotinylated L2 peptide (L2 a.a. 17–36 QLYKTCKQAGTCPPDIIPKV) (JPT) in coating buffer (0.1 M Tris buffer, 0.15 M NaCl, 0.1% Tween 20) at 100 µl/well. L1 or L2 ELISA plates were incubated with blocking buffer (4% skim milk, 0.2% Tween 20 in 1X PBS) for 1.5 h then washed 4 times with wash buffer (0.25% Tween 20 in saline buffer) using a BioTek EL405 plate washer. Sera samples were diluted in blocking buffer at 1:2500 (L1) or 1:5000 (L2) dilution and then serially diluted 1:2 on the plate for 7 more wells for a final volume of 100 µl/well. Sera used for standards and positive controls were generated from mice vaccinated with RG1-VLPs + Alhydrogel, and BALB/c naïve mouse sera (Innovative Research) was used for negative controls. Sera samples were incubated for 1 h at r.t., gently shaking (300 rpm), followed by plate washing. The secondary Ab conjugate goat anti-mouse IgG-horseradish peroxidase (HRP) (Sigma) or goat-anti-mouse IgG2a-HRP (Thermo-Fisher) were added to the plates at a dilution of 1:20,000 or 1:1,000, respectively, and plates were incubated again for 1 h at r.t., gently shaking. After washing, freshly prepared TMB solution (KPL), according to manufacturer’s instructions, was added at 100 µl/well and plates were incubated 25 min at r.t. protected from light. Reactions were stopped by the addition of 100 µl/well of 0.36 N H2SO4. Plate optical density (OD) values were measured at 450/620 nm with a SpectraMax M5 (Molecular Devices) instrument and data processed by SoftMax Pro 6.3 (Molecular Devices). Ab levels, expressed as ELISA units (EU/ml), were then calculated by interpolation of OD values from the standard curve by averaging the calculated concentrations from all dilutions which fell within the range of the standard curve.
VLP and PsV production
As described in,Citation26 293-TTF cells (293 T cells expressing a second large T antigen and furin) were plated at approximately 60–80% confluence in flasks and incubated at 37°C for 24 h. Cells were co-transfected with codon-optimized HPV6-L1/L2 p6shell, HPV16-L1/L2 p16shell, HPV18-L1/L2 p18shell plasmids (kindly provided by J. Schiller, NCI, NIH), HPV39-L1/L2 pVitro plasmid (R. Roden), reporter plasmid pYSEAP (J. Schiller), or reporter plasmid Luciferase (Luc, AddGene) using Lipofectamine 2000 (Thermo Fisher) prepared in serum-free Opti-MEM media (Thermo Fisher). This transfection mixture was added to the cells which were incubated for 48 h and followed by trypsin-mediated cell harvest. Both cells and media were centrifuged, media was decanted, and cells resuspended in buffer 1 (DPBS with 1 g/L D-glucose and 36 mg/L sodium pyruvate) before transfer to 1.5 mL siliconized tubes. Cells were pelleted and resuspended in lysis buffer (DPBS + 10 mM MgCl2, 0.5% Brij58 (Sigma), and RNase A (500 U/ml)/T1 (20 U/ml) cocktail (Ambion)), then incubated for 48 h. Lysates were clarified by centrifugation at 10,000 g for 10 min at 4°C, then layered on top of an Optiprep (STEMCELL) gradient, underlaying 27%, 33%, and 39% Optiprep in a 5 mL thin-wall polyallomer tube (Beckman Coulter). Gradients were ultracentrifuged using a SW55 Ti rotor (Beckman Coulter) at 50,000 rpm for 3.5 h at 16°C with an acceleration of 5 and deceleration of 7. Nine fractions were collected by puncturing tube bottoms with a 18 G needle. 500 µl were collected in tube 1 followed by 250 µl in 8 more tubes. Fraction volumes of 20 µl were electrophoresed on a Coomassie gel to assure purity, and fractions with substantial bands at the predetermined sizes were titrated on LoVo-T cells beginning at 1:125 dilution to assess alkaline phosphatase activity. All fractions that yielded a highly purified band by Coomassie and demonstrated high activity were pooled before use in furin-cleaved pseudovirion-based neutralization assay (fc-PBNA). The dilution factor for PsVs used in the neutralization assay was chosen by identifying the dilution that provided a signal 100–200-fold higher than background signal (no-virus negative control). All fractions that yielded pure PsV bands by Coomassie and could demonstrate a signal 100–200 above background were then combined to make a final PsV pool for each HPV type.
Furin-cleaved PsV-based neutralization assay (fc-PBNA)
As described in,Citation27 LoVo-T cells (ATCC CCL-229, human colorectal adenocarcinoma line expressing SV40 Large T antigen), grown to 70–90% confluency, were removed by Trypsin/EDTA treatment and seeded at 7500 cells/well in a 96-well flat-bottom plate and incubated for 24 h at 37°C, 5% CO2. Pre-diluted (1:25) mouse sera samples were serially diluted 4-fold in DMEM + 10% FBS media in another 96-well plate, including positive and negative control samples derived from RG1-VLP/Alhydrogel-vaccinated mice and naïve mice, respectively. Furin-cleaved PsV (fc-PsV) particles (from HPV types 16, 18, 39, 6) were diluted to pre-determined concentrations (1:1500 for HPV16/39, 1:500 for HPV6, 1:125 for HPV18 based on titration assays), then added to 96-well round-bottom plates followed by equal volumes of serially diluted serum samples. Finally, the plates were incubated for 2 h at 37°C. After incubation, the serum/fc-PsV particle mixtures were added to the 96-well flat-bottom plates previously seeded with LoVo T cells, and the plates were then incubated at 37°C for 72 h, after which cell supernatants were transferred to 96-well Optiplates (Perkin-Elmer) and incubated at 70°C for 45 min. Optiplates were then incubated on ice for 5 min and centrifuged briefly, before SEAP (secreted alkaline phosphatase) substrate (Caymen Chemical) was added, followed by 30 min incubation at r.t., protected from light. Plates were read on a SpectraMax M5 microplate reader. The PBNA titers are reported as the reciprocal of the dilution that caused a 50% reduction in SEAP activity in comparison to the fc-PsV-infected cells without added sera.
In vivo PsV challenge and bioluminescent imaging
On day 35, 7 days after the third vaccination, some mice were injected subcutaneously (s.c.) with 3 mg Depo-Provera (Pfizer), and 4 days later, the vaginal vault of anesthetized recipient mice was instilled with VCF gel (Apothecus Pharmaceutical, containing 4% Nonoxynol-9) using a positive-displacement pipette. The vulva was gently grasped on either side of the pipette tip with smooth dissecting forceps to force retention of the VCF, and then mice were allowed to recover and rest for 6 h. PsV16-Luc and PsV39-Luc inoculate were prepared at 1.5% carboxymethyl cellulose (CMC, Sigma-Aldrich) and administered via positive-displacement pipette to the vaginal vault of anesthetized mice. Dose volumes of PsV16-Luc and PsV39-Luc were determined in previous pilot studies to be sufficient for obtaining a luminescent signal of 5e5-1e6 flux (photons/sec) before background subtraction. Three days after PsV inoculation (day 42) mice were anesthetized and then transferred and positioned ventral side up in the imaging chamber of a Xenogen IVIS Spectrum scanner (PerkinElmer). D-Luciferin (Gold Biotechnology) was deposited by positive-displacement pipette into the vaginal vault. 2D bioluminescent images were acquired within 1 min of luciferin application with an exposure time of 3 min. Bioluminescence signal (total flux in photons/sec) was measured by placing a region of interest (ROI) in the groin area (covering both the vaginal and surrounding area). Additionally, a background ROI was placed over the thoracic area for purposes of measuring constitutive background signal. Total flux values from both inoculated target regions and non-targeted background regions were first normalized for area of the ROI, and then the respective normalized background signal was subtracted from the normalized signal of the target regions for each animal. To obtain % reduction values, the formula (X-Y)/X (100) was used where X is the mean normalized targeted value for untreated mice and Y is the mean normalized target value for any of the vaccinated groups.
Statistical analysis
Statistical analyses were conducted with GraphPad Prism 7 software using one-way ANOVA nonparametric analysis with the Kruskal–Wallis multiple comparisons test. p < .05 was considered significant.
Results
Biophysical characterization of polyphosphazene-adjuvanted VLP vaccine formulations
Formulations of PCPP and PCEP with RG1-VLPs were prepared by simple mixing of aqueous solutions and were characterized using DLS and AF4 methods. DLS studies revealed that the dimensions of VLPs and both PP adjuvants were in the same range (60–70 nm). Although their binary formulations were also characterized with a unimodal distribution, the resulting assemblies had a somewhat larger hydrodynamic diameter (), which clearly indicates interactions in the system. AF4 analysis independently confirmed spontaneous self-assembly in the VLPs-PP system. This physicochemical method is capable of analyzing supramolecular assemblies with up to 1 µm sizes by detecting molecules or particles that were subjected to a cross-flow of mobile phase which press analytes against a semi-permeable membrane, thereby slowing them in a size-dependent manner.Citation25 Although AF4 analysis of pure VLPs did not allow for the detection of particles, which is probably due to their nonspecific adsorption to the membrane, analysis of polymer-treated VLPs resulted in a signal larger than that of the polymer itself (). The size of VLP-PP assemblies was largely dependent on the ratio of the components. Severe aggregation was detected by DLS at a low polymer content (0–2 polymer-to-VLP w/w ratio) for both polymers (). However, further increases in polymer concentration prevented aggregation and only manifested in a slight (20–50%) increase in hydrodynamic diameters compared to individual components
Figure 1. Characterization of particle size and aggregation potential of VLP/PP formulations. (A, B) DLS profiles of VLPs, PPs, and formulations of VLPs with PCEP (A) and PCPP (B) at 1:2.5 (w/w) ratio (PBS, pH 7.4); (C) z-average hydrodynamic diameter of VLPs-PCPP and VLPs-PCEP versus ratio of formulation components. (D) Schematics of suggested mechanisms of interactions between VLPs and polymers is also shown (0.05 mg/mL VLPs, PBS, pH 7.4)
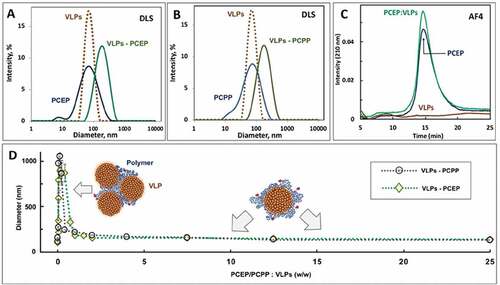
We also compared the short-term stability of VLP formulations adjuvanted with PCPP or PCEP. Formulations were kept at 4°C for three days and changes in z-average hydrodynamic diameters were monitored using DLS. A significant increase (50–60%) in the dimensions of supramolecular assemblies over time was detected for PCPP (). In contrast, PCEP-adjuvanted VLPs displayed only a minor increase (5–15%) in diameter. Further comparative analysis of the two formulations was conducted via evaluation of their pH-dependent membrane disruptive activity using the hemolysis test, which generally can be correlated to the ability of the delivery vehicle to facilitate endosomal escape and cytosolic delivery of pharmaceutical cargo.Citation28–30 Whereas the PCEP-VLP formulation showed strong hemolytic activity below pH 6.8, no hemolysis was detected for PCPP formulations regardless of the pH of the media ()
Figure 2. Physicochemical analysis of VLP-PP formulations. (A) Change in z-average hydrodynamic diameter of PCPP-VLP and PCEP-VLP formulations versus time (D0 – diameter at 0 h; PBS, pH 7.4, 4°C, 0.5 mg/mL PCPP or PCEP, 0.02 mg/mL VLPs); (B) endosomolytic activity of PCEP-VLP and PCEP-VLP formulations as assessed by their hemolytic activity at various pH (red blood cells, 0.025 mg/mL polymer concentration, 10 mM phosphate buffer, 0.9% sodium chloride)
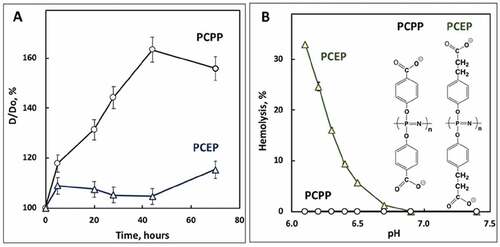
PP adjuvants induce VLP-specific humoral immunity to levels equivalent or superior to Alhydrogel
To investigate the in vivo adjuvant properties of PECP in a VLP-based vaccine, we utilized RG1-VLPs, the novel chimeric HPV vaccine candidate comprised 72 HPV16-L1 pentameric capsomers, with each L1 engineered to express a 20 amino acid sequence from the HPV16-L2 capsid protein termed RG1Citation15 for a display total of 360 RG1 epitopes/particle. Unlike L1 capsid sequences, the RG1 epitope of the L2 sequence is well-conserved among disparate HPV strains and promotes the generation of cross-neutralization Abs in vivo in mice. When adjuvanted with aluminum salt formulations such as Alhydrogel,Citation15 this vaccine design can provide protection to a broad repertoire of HPV strains. We immunized BALB/c mice three times i.m. with 3-week intervals between injections with RG1-VLPs and either Alhydrogel (alum), the PP compounds PCPP or PCEP, or the nonavalent FDA-approved HPV VLP vaccine Gardasil-9. Sera were analyzed for Abs specific to HPV16-L1 and L2 via ELISA and were additionally tested for neutralizing Ab titers against HPV16/18/39/6 by fc-PBNA.
While alum augmented the geometric mean of L1 Ab levels compared to VLPs alone, this increase did not reach statistical relevance. Adjuvanting with either PCPP or PCEP, however, resulted in substantially higher L1 Ab levels that did achieve statistical superiority over VLPs alone and furthermore exhibited significantly higher increases than the alum or Gardasil-9 groups (), with the highest degree of difference demonstrated by PCEP. RG1-VLPs express the RG1 epitope in a highly repetitive and immunogenic manner, and detectable L2-specific Ab levels are achieved by immunization with RG1-VLPs without adjuvant. Combining VLPs with alum, PCPP, or PCEP all induced L2 Ab levels significantly higher than VLPs alone (). As expected, Gardasil-9 vaccination resulted in no detectable L2-specific responses
Figure 3. Enhancement of RG1-VLP-specific humoral immunity in the presence of PP adjuvant formulations. Mice were immunized i.m. with 2 µg RG1-VLP alone or adjuvanted with 50 µg alum, 25 or 50 µg PCPP, 25 or 50 µg PCEP or Gardasil-9 on days 0, 21, 42 and peripheral blood sera samples derived on day 56. (A-B) Sera samples were tested for HPV16-L1- and HPV16-L2 RG1-specific IgG via ELISA. (C-F) Sera samples were analyzed for neutralizing titers via fc-PBNA specific for PsV16-, PsV18-, PsV39-, PsV6-SEAP. Data are reported as geometric means ± 95% confidence interval (CI). Statistical comparisons are between VLPs alone and all groups or between alum and all groups (upper tier for A and C) and were generated using one-way ANOVA nonparametric analysis with the Kruskal-Wallis multiple comparisons test. ns, not significant (p > .05); *, p < .05; **, p < .01; ***, p < .001; ****, p < .0001
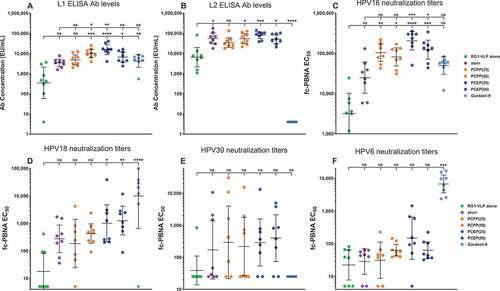
The RG1 epitope of L2 is buried below the capsid surface of native virions and is only transiently accessible to neutralizing antibodies during the infectious process upon a conformation change and specific cleavage of L2 by extracellular furin that is triggered by virion binding to the extracellular matrix or basement membrane. An in vitro furin-dependent cell-based neutralization assay was developed to more closely resemble in vivo infectivity.Citation26 Titers of neutralization Abs that prevent the in vitro infection of LoVo-T cells by SEAP-expressing HPV16 PsVs were substantially higher when the RG1-VLP vaccine had been adjuvanted by PP compounds, particularly by PCEP which achieved a geometric mean 2X higher than PCPP at the 25 µg dose (). Cross-neutralization Abs elicited by the RG1-VLP vaccine were demonstrated by the presence of titers specific to HPV18 and HPV39, since the RG1-VLP is comprised of HPV16-L1 capsid scaffold only. PCEP adjuvant induced higher geomean cross-neutralizing titers compared to alum, although statistical difference was only achieved in the case of HPV18 (). Since Gardasil-9 includes the HPV18 but not HPV39 L1 VLP, it achieved high neutralizing titers for HPV18 but did not demonstrate any capacity to induce a cross-neutralizing response to HPV39. Adjuvanting RG1-VLPs with alum appears to be least effective for inducing elevated levels of cross-neutralizing Abs to HPV6, likely reflecting its more divergent sequence within the RG1 epitope (). Only PCEP elevated levels of HPV6-neutralizing titers in some mice, although significance to VLPs alone was not achieved. Since PCEP routinely outperformed PCPP, we focused further studies on the adjuvant capabilities of PCEP.
VLP dose-sparing achieved by PCEP adjuvanting of the RG1-VLP vaccine
We investigated the antigen dose-sparing capabilities that utilization of PCEP might allow. Due to the superior responses of 25 µg over 50 µg PCEP in several humoral immune assays (), we investigated the activity of lower doses of PCEP to facilitate vaccine responses to decreasing amounts of RG1-VLPs. Even at a 50–75% VLP dose reduction (from 2 to 1 or 0.5 µg), PCEP promoted responses equivalent or superior to 2 µg VLPs + alum or Gardasil-9 in all assays, including L1- and L2-specific Ab levels and neutralization titers to HPV16/18/39/6 (). PCEP adjuvantation of 0.5 µg VLPs even achieved statistical significance over 2 ug VLPs alone in the cases of L1 Ab levels and HPV-16 neutralizing titers (), which Alhydrogel adjuvantation of 2 µg VLPs was unable to accomplish, underlining the VLP dose-sparing capacity that PCEP confers
Figure 4. PCEP adjuvant formulations allow for dose-sparing of VLPs and PCEP. Mice were immunized i.m. with 0.5–2.0 µg RG1-VLP alone or adjuvanted with 50 µg alum, 7.5 or 15 µg PCEP, or Gardasil-9 on days 0, 14, 28 and peripheral blood sera samples derived on day 42. (A-B) Sera samples were tested for HPV16-L1- and HPV16-L2 RG1-specific IgG via ELISA. (C-F) Sera samples were analyzed for neutralizing titers via fc-PBNA specific for PsV16-, PsV18-, PsV39-, PsV6-SEAP. (F) Numbers of responding mice with detectable titers of PsV6-neutralizing activity are indicated. Data are reported as geometric means ± 95% CI. Statistical comparisons are between VLPs alone and all groups or between alum and all groups (upper tier for C) and were generated using one-way ANOVA nonparametric analysis with the Kruskal-Wallis multiple comparisons test. ns, not significant (p > .05); *, p < .05; **, p < .01; ***, p < .001; ****, p < .0001
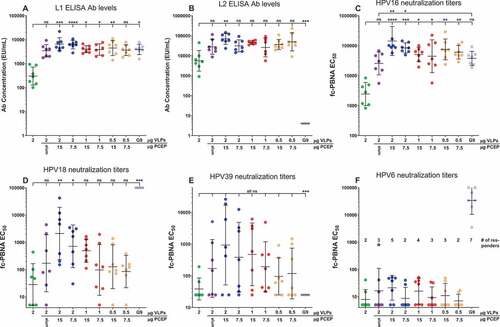
PCEP accelerates the vaccine schedule compared to Alhydrogel and provides for long-lasting Ab responses
The RG1-VLP vaccine requires two additional boosts after the initial prime vaccination to yield substantial levels of L1 and L2-specific Abs when adjuvanted with Alhydrogel alone.Citation14 To determine whether the addition of PCEP could accelerate the kinetics of the humoral response to RG1-VLP vaccine, we measured Ab levels to both L1 and L2 at earlier timepoints, 2 weeks after the first immunization (day 14) and 2 weeks after the second immunization (day 28), as well as 2 weeks after the third immunization (day 42). PCEP substantially elevated L1 and L2 ELISA Ab levels compared to alum alone after only 2 immunizations when the VLP dose was 2 µg ()). At lower amounts of VLPs (1 or 0.5 µg), PCEP still induced levels of L1/L2 Abs by day 28 (after 2 immunizations) that were statistically higher than levels achieved by VLPs alone, while alum-induced L1/L2 levels failed to achieve statistical significance over VLPs alone at day 28. Gardasil-9 did achieve significance over VLPs alone at day 28, indicative of a potentially faster-acting mechanism for L1 Ab induction compared to RG1-VLPs + alum. Furthermore, day 28 L2-specific Ab levels induced by PCEP at all 3 VLP doses were equivalent to or higher than day 42 L2-specific Ab levels induced by the alum-formulated vaccine after 3 vaccinations (), indicative of the accelerated kinetics of L2 Ab response when PCEP is utilized. We also analyzed day 28 sera samples for neutralizing Ab titers to HPV16 and HPV18 and found that PCEP increased the appearance of both types after only 2 immunizations, achieving statistical relevance compared to VLPs alone, which RG1-VLPs + alum was not able to accomplish (). These data indicate that adjuvanting the RG1-VLP vaccine with PCEP could lead to significant COG savings by decreasing the schedule to fewer immunizations as well as allowing for less VLPs per injection
Figure 5. PCEP accelerates the appearance of L1/L2 Ab levels as well as HPV16/18-neutralization titers. Mice were immunized i.m. with 0.5–2.0 µg RG1-VLP alone or adjuvanted with 50 µg alum, 7.5 or 15 µg PCEP, or Gardasil-9 on days 0, 14, 28 and peripheral blood sera samples derived on days 28, 42. (A-B) Sera samples were tested for HPV16-L1- and HPV16-L2 RG1-specific IgG via ELISA. Statistical comparisons are between VLPs alone and adjuvanted groups for each time point; absence of designation indications no statistical difference. (C-D) Day 28 sera samples were analyzed for neutralizing titers via fc-PBNA specific for PsV16-, PsV18-SEAP. Data are reported as geometric means ± 95% CI. Statistical comparisons are between VLPs alone and adjuvanted groups, or between alum and PCEP groups (upper tier in C) and were generated using one-way ANOVA nonparametric analysis with the Kruskal-Wallis multiple comparisons test. ns, not significant (p > .05); *, p < .05; **, p < .01; ***, p < .001
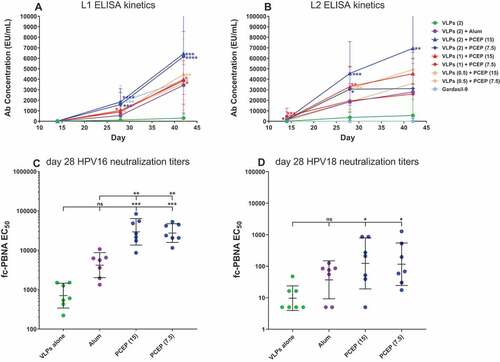
To measure the durability of circulating L1/L2 Abs observed in PCEP-adjuvanted responses compared to alum, we continued to monitor vaccinated mice after the third immunization at day 42 for 3 more months, harvesting sera at monthly time points. RG1-VLPs at the 2 µg dose were combined with high (50 µg) and low dose (15 µg) PCEP. L1 Ab levels were higher with either dose of PCEP compared to alum or Gardasil-9 at day 42 and although some slight waning of all responses was observed, at least one of the two PCEP groups maintained significantly higher L1 Ab levels even after 3 months of monitoring (). PCEP-adjuvanted L2 Ab levels also maintained elevated levels over 3 months after the second boost with only minor waning and continued to maintain consistent elevation over VLPs alone (), suggesting the higher magnitude responses induced with the PCEP adjuvant are long-lasting and remain elevated for months
Figure 6. PCEP promotes a sustained elevation of the L1 and L2 Ab response over several months. Mice were immunized i.m. with 2 µg RG1-VLP alone or adjuvanted with 50 µg alum, or 15 or 50 µg PCEP, or Gardasil-9 on days 0, 14, 28 and peripheral blood sera samples derived on days 42, 70, 98, 125. (A-B) Sera samples were tested for HPV16-L1- and HPV16-L2-specific IgG via ELISA. Data are reported as geometric means ± 95% CI. Statistical comparisons were generated using nonparametric Mann-Whitney t-test. p > .05; *, p < .05; **, p < 001
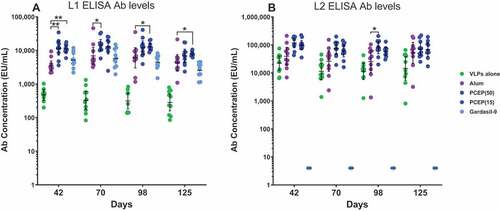
PCEP-provided protection against PsV39 challenge in vivo
Although the fc-PBNA assay measures the ability of vaccine-generated sera to block infection of LoVo-T cells in vitro by HPV PsVs expressing SEAP, we investigated whether antisera induced by RG1-VLPs + PCEP could provide protection against PsVs expressing Luciferase in vivo. Mice were vaccinated 3X with biweekly intervals with Gardasil-9 or with RG1-VLPs ± Alhydrogel or PCEP and 11 days post third immunization, the vaginal epithelia of vaccinated mice were disrupted with Nonoxynol-9 followed by intravaginal inoculation with PsV16 or PsV39 expressing Luc. After 3 days of incubation, recipient mice were treated intravaginally with luciferin and the resulting luminescent signal imaged 1–5 minutes later. Immunizations with unadjuvanted RG1-VLPs alone conferred robust protection against challenge with PsV16-Luc (), presumably because the backbone of RG1-VLP is composed of a repeating array of 360 HPV16-L1 capsid proteins and therefore the generation of high levels HPV16-neutralizing Abs is accomplished by the inherent adjuvant activity conferred by the nature of the VLP particle. Indeed, neither the presence of alum nor PCEP were able to suppress the luminescent signal any further. Vaccination with RG1-VLPs alone was also able to confer a substantial, albeit not complete, level of protection against inoculation with PsV39-Luc (). In this case, adjuvanting with Alhydrogel or PCEP further decreased the luminescent signal and achieved stronger statistical significance compared to no treatment than did VLPs alone, accentuating the benefit that the presence of either adjuvant could bring to increasing the level of cross-neutralizing protection against HPV39. Gardasil-9 was unable to demonstrate any protective effect versus HPV39 as this HPV type is not one of the nine VLP species comprising that vaccine.
Figure 7. Vaccination with PCEP-adjuvanted VLPs provides protection against HPV39 inoculation. Mice were immunized i.m. with 2 µg RG1-VLPs alone or adjuvanted with 50 µg alum or 50 µg PCEP, or with Gardasil-9, or with no treatment (no Tx) on days 0, 14, 28. On day 39, mice were intravaginally inoculated with PsV16-Luc or PsV39-Luc, and 3 days later, luciferin was applied topically to the site of inoculation. 2D bioluminescent images were captured and signal quantitated. (A) Bioluminescent values were plotted individually with geomeans ± 95% CI. Statistical comparisons are between no Tx and vaccinated groups and were generated using one-way ANOVA nonparametric analysis with the Kruskal-Wallis multiple comparisons test. *, p < .05; **, p < .01; ***, p < .001, ****, p < .0001. (B) Bioluminescent signal is imaged for all mice with ROIs drawn over the ventral vaginal area and over the ventral thoracic area for background signal
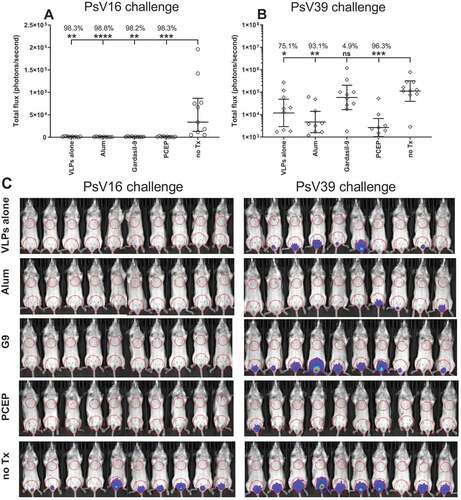
Promotion of IgG2a isotype switch by PCEP
Alum-based adjuvants have been well described as preferentially directing IgG subtype responses in mice to the Th2 switch factor-dependent IgG1 subtype with much less contribution by subtypes IgG2a, IgG2b, and IgG3, which usually align with Th1 responses.Citation31 To determine whether the superior total IgG responses achieved by adjuvanting with PCEP compared to alum also reflected a difference in subtypes, sera samples were analyzed for IgG2a content. PCEP-adjuvanted RG1-VLPs strongly promoted high levels of IgG2a specific to both L1 and L2 and substantially more than observed in the presence of alum (, B))
Figure 8. Elevated IgG2a titers induced by adjuvanting with PCEP. Mice were immunized i.m. with 2.0 µg RG1-VLPs alone or adjuvanted with 50 µg alum (Alhydrogel), 50 or 15 µg PCEP, or Gardasil-9 on days 0, 14, 28 and peripheral blood sera samples were derived on day 42. (A-B) Sera samples were tested for HPV16-L1- and HPV16-L2 RG1-specific IgG2a via ELISA. Data are reported as geometric means ± 95% CI. Statistical comparisons are between VLPs alone and adjuvanted groups (lower tier), or between alum and PCEP groups (upper tier) and were generated using one-way ANOVA nonparametric analysis with the Kruskal-Wallis multiple comparisons test. ns, not significant (p > .05); **, p < .01; ***, p < .001; ****, p < .0001
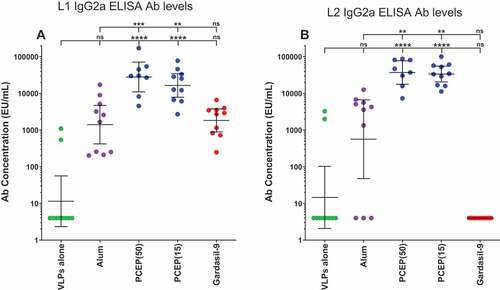
Discussion
Although the FDA-approved vaccines Gardasil-9 and Cervarix are effective at providing protection against HPV infection restricted to their respective nonavalent and quadrivalent HPV type repertoires, as well as preventing the onset of HPV-related cervical and oropharyngeal cancers, they could still further benefit from optimization of formulation and schedule. Currently, recommendations of the CDC’s Advisory Committee on Immunization Practices (ACIP) are for a 3-injection regimen at 1, 2, and 6 months with the standard clinical dose of VLPs ranging from 20 to 40 μg per VLP species, representing a substantial COG investment. The novel RG1-VLP vaccine shows significant potential for achieving broad immunity against high-risk HPV genotypes using only a single VLP species; however, the current Alhydrogel adjuvant (alum) may not optimize the response to the L2 component. Our investigation of substitution of Alhydrogel with the novel PP compound PCEP demonstrates that none of the humoral readouts in vaccinated mice showed any underperformance with PCEP, suggesting the compound could be suitable as a substitute for Alhydrogel. In fact, adjuvanting with PCEP resulted in dramatically improved levels of L1 Abs, HPV16-, and HPV18-neutralizing titers, and modestly improved levels of L2 Abs and HPV39-neutralizing titers. Additionally, the selection of PCEP also resulted in dose-sparing of VLPs, an accelerated attainment of L2 Ab titers after two immunizations equivalent to three immunizations with alum, and a longer-lasting circulating Ab response. These data emphasize the advantage that adjuvanting with PP compounds could convey in terms of simplifying the vaccine schedule as well as improving the cost:benefit ratio by allowing for reduction of VLP species number and dose.
PP compounds convey adjuvant effects primarily through two mechanisms: acting as a carrier vehicle for soluble antigen and through direct triggering of certain immunostimulatory pathways. The complex formation process by which nanoparticles are generated via spontaneous assembly of the PP polymers and soluble antigen allows for recognition by cell surface receptors such as the Mannose ReceptorCitation20 and potentially the Scavenger Receptor on the surface of APCs. This process greatly increases the amount of antigen received per APC, improves activity for receptor cross-linking, and additionally activates antigen-presenting machinery and APC maturation.Citation32 PPs are also known to facilitate endosomal escape, which may correspond with facilitation of the release of antigenic cargo into antigen-processing pathways.Citation33 Mounting evidence suggests that PP compounds can directly trigger several pathogen recognition receptors (PRRs), the primary functions of which are activation and augmentation of immune responses. Much of this evidence is indirect, in that PP compounds have been observed to induce expression of several inflammatory chemokines and TNF-α in miceCitation34 and recruitment of neutrophils, macrophages, T cells, and DCs to draining lymph nodes in mice,Citation35 responses often attributed to PRR activation by pathogens. Candidate PRR targets include TLR3, TLR4, and TLR9, as molecular interactions between those PRRs and PP compounds have been described via AF4 methods.Citation20 Like alum-based adjuvants, PP compounds have also been combined with TLR agonists such as poly(I:C) (TLR3L), R848 (TLR7/8 L), or CpG (TLR9L)Citation36–38 which underlines the potential for PPs to be incorporated into more complex adjuvant formulations.
Physicochemical characterization of PP-adjuvanted formulations using DLS and AF4 methods revealed the occurrence of intermolecular interactions and spontaneous self-assembly (). Although both components of the formulation were of similar dimensions, VLPs presented a system with a well-defined rigid surface, whereas PP adjuvants can be described as highly flexible macromolecules with a random coil conformation in solution.Citation20,Citation39–41 Once they encounter a solid surface, these polymers are known to easily adapt their conformation to create nanocoatings.Citation42,Citation43 The detectable but relatively minor increase in the hydrodynamic diameter of the assemblies compared to the formulation components suggests that flexible PPs form a polymer crown around VLPs similarly to the spatial arrangements well documented in other systems, where synthetic polymers were attached to VLPs via covalent bonding.Citation44,Citation45 These suggested non-covalently assembled shell-core constructs are illustrated in ) (right side). Biophysical characterization data also reveal that aggregation of the VLPs can take place when the polymer is used in deficiency and multiple VLPs are associated with the same polymer (), left side). Therefore, for proper vaccine formulation, care must be taken to avoid aggregating conditions and to perform physicochemical characterization of the formulation before in vivo experiments.
The superior immunoadjuvant potency of PCEP compared to PCPP has been documented in several studies.Citation23,Citation25,Citation46 However, no meaningful explanation to this phenomenon in the framework of structure–activity relationship has been suggested to date as structural differences between these two members of a homologous family are minor ()). Nevertheless, two noteworthy distinctions were observed in the behavior of these two formulations in vitro. PCEP-formulated VLPs demonstrated superior resistance to aggregation compared to PCPP-VLPs ()). This may be a result of a previously reported unusual sensitivity of PCPP to sodium ions, manifesting in a significantly lower phase separation threshold compared to PCEP.Citation24,Citation25,Citation47 It is possible that interactions with antigenic cargo like VLPs, which are usually realized via ionic bonds, can reduce the overall negative charge of the polymer and further facilitate destabilization of the system.Citation20 Yet another distinct difference between PCPP and PCEP is in the ability of PCEP to display membrane-disrupting activity at the pH of early endosomes, the result of the higher hydrophobicity of PCEP.Citation20 This feature was previously correlated with the ability of some vaccine carriers to facilitate the endosomal escape of antigenic cargo, thereby directing it into the major histocompatibility complex (MHC) class I antigen presentation pathway.Citation48–50 It is noteworthy that this mechanism of cross-presentation has been shown to play an important role in the immune defense against many viruses, including HPV.Citation51 VLP formulations modified with PCEP exhibit potent endosomolytic activity, which was assessed in the hemolysis test, a property that PCPP-adjuvanted VLPs evidently lack ()). These physicochemical differences in formulations of VLPs with either PCPP or PCEP may support an explanation of the superior potency of PCEP.
Further differences in activity between PCPP and PCEP may track back to preference of IgG subclass induction. Aluminum-based adjuvants exhibit a well-described ability to induce vaccine antigen-specific Th2 responses in mice. This has often been documented in terms of IgG1:IgG2a ratios, with alum responsible for the induction of high ratios, as IgG1 responses commonly accompany Th2 differentiation in mice and utilize the Th2 cytokine IL-4 as the switch factor from IgM to IgG1.Citation52 Elevated production of IL-4, IL-5, and IL-13, a Th2 cytokine profile, by T cells in mice immunized with alum-adjuvanted vaccines has also been described.Citation52 Alum-activated Th2 responses in humans have been more difficult to measure, partially due to a looser association between IgG subtypes and cytokine profiles. IgG1 and IgG3 are generally thought to correspond to Th1 responses while Th2 responses are accompanied by elevated IgE and IgG4 levels,Citation53 and high levels of the latter have commonly been observed in allergen-specific Th2 responses in atopic individuals.Citation54 In addition, Th2 responses to vaccination of human subjects with KLH + aluminum hydroxide are characterized by T cell-derived IL-5 and IL-13 as well as elevated IgG4 levels.Citation55 Induction of Th2-type responses by vaccines adjuvanted with aluminum-based compounds may be conducive for high levels of total IgG, but such responses could also pose a risk factor in subjects predisposed for atopic reactions.Citation56 PCEP demonstrated superiority to Alhydrogel in its ability to induce high titers of the IgG2a subtype specific to both L1- and L2-specific responses. Previous investigations of the use of PCEP as a vaccine adjuvant with the X:31 influenza antigen in mice have reported elevated IgG2a Ab responsesCitation46,Citation57 as well as T cell responses characterized by both IFN-ɣ and IL-4 secretion, in comparison to alum and PCPP formulations, which promoted low IgG2a and IL-4-dominant T cell secretion profiles.Citation35,Citation37,Citation46 The mechanism by which PCEP promotes Th1 differentiation is unclear but may involve activation of the NLRP3 inflammasome pathway, which leads to caspase-1-dependent conversion of pro-IL-1β and pro-IL-18 into bioactive forms.Citation35 As activation of this pathway has also been observed during the use of aluminum-based adjuvants,Citation58 there may be additional innate immune response mechanisms triggered by PCEP that are triggered less efficiently by either alum or PCPP and are responsible for its high performance. Substitute adjuvants like PCEP that demonstrate no predisposition for the development of Th2 differentiation, and in fact promote IgG2a switching in mice, may be a less risky choice for atopic vaccinees.
Neutralization of PsV16-SEAP in the fc-PBNA assay is mediated through L1-specific Abs generated through recognition of the HPV16-L1 subunit scaffold of RG1-VLP. However, since no sequence of HPV18, HPV39, or HPV6, L1, or L2, are present in the RG1-VLP, neutralization effects determined by the fc-PBNA assay against those three HPV types are therefore cross-neutralizing and can only be conducted through the L2-specific Ab response. Our study confirmed that the PCEP-augmented RG1-VLP vaccine demonstrated strong potency for the induction of neutralizing Abs to HPV16 and substantial capacity for induction of cross-neutralizing Abs to HPV18 and HPV39. However, only a few mice were induced to substantially increase HPV6-neutralizing titers after vaccination with PCEP compared to Alhydrogel, and the rise in the geomean was not statistically relevant ()). This difficulty in provoking an HPV6-cross-neutralizing response likely stems from the more significant disparity in RG1 epitope sequences between HPV16 and HPV6 compared to HPV16 and either HPV18 or HPV39. Alanine-scanning peptide ELISA has been used to map several L2 cross-neutralizing Abs to the amino acid sequence 20–31 within the HPV16-L2 RG1 epitope.Citation59 Disruption of either of the cysteines within this sequence at positions 22 and 28, critical for disulfide-bonding, resulted in loss of mAb binding. When L2 20–31 a.a. sequence homologies amongst the four HPV types analyzed in this study are compared using the BLOSUM62 comparison matrix, HPV6-L2 revealed the lowest homology to HPV16-L2, which is more conserved with HPV18-L2 and HPV39-L2 (). ClustalW analysis has suggested that RG1 mAb requires K20 and P29 for optimal neutralizing Ab activity and that A or S at L2 residue 25 are tolerated but not T.Citation60 The HPV6-L2 RG1 epitope has a Q at residue 20 and a T at residue 25 which may hinder optimal association with HPV16-L2-generated neutralizing Abs. Furthermore, the HPV6-L2 sequence stands out for a Q24L variation not shared by HPV18/39, a nonconservative polar to nonpolar change that may impact binding of cross-neutralizing Abs generated against the HPV16-L2 sequence in RG1-VLPs. Indeed, a consensus sequence for the longer HPV-L2 17–36 a.a. segment reveals a closer alignment of the RG1 sequence of HPV16, HPV18, and HPV39, compared to HPV6.Citation13 Our results suggest that HPV16-L2-generated Abs may poorly bind the HPV6-L2 RG1 epitope and underline the advantage that could be accrued by optimizing HPV-L2-containing VLP vaccines to express >1 RG1 epitopes in order to maximize type coverage, including low-risk types HPV6/11.Citation61
Table 1. L2 RG1 epitope sequences compared between HPV types. The amino acid sequences at positions 20–31 of L2 capsid for HPV16/18/39/6 were analyzed by BLOSUM62 comparison matrix for alignment
Although the neutralization of PsV18- and PsV39-SEAP describes the ability of the RG1-VLP vaccine to cross-neutralize infection by non-HPV16 types, further analyses are warranted to measure the extent of capacity for cross-neutralization, e.g. the neutralization of additional vaccine HPV types 31/33/45/52/58. Development of neutralizing assays specific to these HPV types is underway. Additional cross-neutralization data were derived from the in vivo PsV challenge model which demonstrated that the RG1-VLP vaccine was successful in greatly decreasing the efficiency of PsV39-SEAP infection of mouse vaginal epithelia. However, adjuvanting the vaccine with either alum or PCEP resulted in the equivalent and near-complete reduction of PsV39-Luc expression ()), indicating that the sensitivity of this assay to distinguish between two highly active vaccine formulations could be heightened. Further refinement of the in vivo PsV challenge model by increasing the PsV inoculation titer, reducing the number of vaccinations, or expanding the total viral burden by introducing oral inoculation techniques are in process.
While current HPV vaccines have enjoyed remarkable success at reducing rates of HPV infection and subsequent incidence of HPV-related cancers, there remains a need for improved vaccines that will lower COG, reduce the vaccine schedule, and amplify the humoral response. Our data indicate that substitution of Alhydrogel with PCEP can optimize the potency of the novel RG1-VLP vaccine by enhancing the magnitude of the Ab response, by allowing for VLP dose-sparing, by accelerating the appearance of protective levels of neutralizing Abs and thus allowing for a shorter vaccine schedule, and by the promotion of longer-lasting Ab responses. This novel formulation could take the form of a lyophilized or freeze-dried vaccine, as both VLPs and PP compounds have been demonstrated to be lyophilizable and retain activity upon reconstitution.Citation62,Citation63 This formulation could also be handled at room temperature without the requirement for refrigeration like most alum-based vaccines. Given the uncertainty behind alum’s mechanism of action and propensity for induction of Th2 responses, which could be problematic for atopic individuals, further investigation of adjuvanting RG1-VLPs with optimized PP compounds may lead to the development of a low-cost, high efficacy next-generation HPV vaccine and also provide another candidate for the clinical adjuvant toolbox.
Abbreviations
Ab | = | Antibody |
AF4 | = | Asymmetric flow field flow fractionation |
CI | = | Confidence interval |
CMC | = | Carboxymethyl cellulose |
COG | = | Cost of goods |
DLS | = | Dynamic light scattering |
ELISA | = | Enzyme-linked immunosorbent assay |
fc-PBNA | = | Furin-cleaved pseudovirion-based neutralization assay |
HPV | = | Human papilloma virus |
i.m. | = | Intramuscular |
Luc | = | Luciferase |
OD | = | Optical density |
PCEP | = | Poly[di(carboxylatoethylphenoxy)phosphazene] |
PCPP | = | Poly[di(carboxylatophenoxy)phosphazene] |
PP | = | Polyphosphazene |
PRR | = | Pathogen recognition receptor |
PsV | = | Pseudovirion |
RBC | = | Red blood cell |
ROI | = | Region of interest |
r.t. | = | Room temperature |
s.c. | = | Subcutaneous |
SEAP | = | Secreted alkaline phosphatase |
VLP | = | Virus-like particle |
Disclosure of potential conflicts of interest
No potential conflicts of interest were disclosed.
Acknowledgments
We gratefully acknowledge the invaluable assistance of Dr. Joseph Kalen, director of the Small Animal Imaging Program of the Laboratory of Animal Sciences Program at NCI-Frederick and support staff Nimit Patel for the performance of bioluminescent imaging mouse studies. This project has been funded in whole with Federal funds from the National Cancer Institute, National Institutes of Health, under Contract No. HHSN261200800001E. The content of this publication does not necessarily reflect the views or policies of the Department of Health and Human Services, nor does mention of trade names, commercial products, or organizations imply endorsement by the U.S. Government. RBR was supported by the National Cancer Institute of the National Institutes of Health under awards P30CA06973, P50CA098252, R01CA233486, R01CA237067. RBR and RK are members of PathoVax LLC and its Scientific Advisory Board. Under a licensing agreement between Pathovax Biotech, Inc., the National Institutes of Health and the Johns Hopkins University, the University and RBR are entitled to royalties on an invention described in this article. Under a licensing agreement between PathoVax LLC, the Johns Hopkins University, and University of Vienna Medical School, the University and RK are entitled to royalties associated with an invention described in this publication. These arrangements have been reviewed and approved by the Johns Hopkins University and Medical University of Vienna, respectively, in accordance with their conflict of interest policies. NCI-Frederick is accredited by AAALAC International and follows the Public Health Service Policy for the Care and Use of Laboratory Animals. Animal care was provided in accordance with the procedures outlined in the “Guide for Care and Use of Laboratory Animals” (National Research Council; 2011; National Academy Press; Washington, D.C.).
Additional information
Funding
References
- Serrano B, Alemany L, Tous S, Bruni L, Clifford GM, Weiss T, Bosch F, de Sanjosé S. Potential impact of a nine-valent vaccine in human papillomavirus related cervical disease. Infect Agent Cancer. 2012;7(1):38. doi:10.1186/1750-9378-7-38.
- Jagu S, Kwak K, Schiller JT, Lowy DR, Kleanthous H, Kalnin K, Wang C, Wang H-K, Chow LT, Huh WK, et al. Phylogenetic considerations in designing a broadly protective multimeric L2 vaccine. J Virol. 2013;87(11):6127–36. doi:10.1128/JVI.03218-12.
- Roden RB, Yutzy WH, Fallon R, Inglis S, Lowy DR, Schiller JT. Minor capsid protein of human genital papillomaviruses contains subdominant, cross-neutralizing epitopes. Virology. 2000;270(2):254–57. doi:10.1006/viro.2000.0272.
- Roden RB, Greenstone HL, Kirnbauer R, Booy FP, Jessie J, Lowy DR, Schiller JT. In vitro generation and type-specific neutralization of a human papillomavirus type 16 virion pseudotype. J Virol. 1996;70(9):5875–83. doi:10.1128/JVI.70.9.5875-5883.1996.
- Chen XS, Garcea RL, Goldberg I, Casini G, Harrison SC. Structure of small virus-like particles assembled from the L1 protein of human papillomavirus 16. Mol Cell. 2000;5(3):557–67. doi:10.1016/S1097-2765(00)80449-9.
- El Aliani A, El Abid H, Kassal Y, Khyatti M, Attaleb M, Ennaji MM, El Mzibri M. HPV16 L1 diversity and its potential impact on the vaccination-induced immunity. Gene. 2020;747:144682. doi:10.1016/j.gene.2020.144682.
- Gambhira R, Karanam B, Jagu S, Roberts JN, Buck CB, Bossis I, Alphs H, Culp T, Christensen ND, Roden RBS, et al. A protective and broadly cross-neutralizing epitope of human papillomavirus L2. J Virol. 2007;81(24):13927–31. doi:10.1128/JVI.00936-07.
- Chen X, Liu H, Zhang T, Liu Y, Xie X, Wang Z, Xu X. A vaccine of L2 epitope repeats fused with a modified IgG1 Fc induced cross-neutralizing antibodies and protective immunity against divergent human papillomavirus types. PLoS One. 2014;9(5):e95448. doi:10.1371/journal.pone.0095448.
- Motavalli Khiavi F, Arashkia A, Golkar M, Nasimi M, Roohvand F, Azadmanesh K. A dual-type L2 11-88 peptide from HPV types 16/18 formulated in montanide ISA 720 induced strong and balanced Th1/Th2 immune responses, associated with high titers of broad spectrum cross-reactive antibodies in vaccinated mice. J Immunol Res. 2018;2018:9464186. doi:10.1155/2018/9464186.
- Vujadinovic M, Khan S, Oosterhuis K, Uil TG, Wunderlich K, Damman S, Boedhoe S, Verwilligen A, Knibbe J, Serroyen J, et al. Adenovirus based HPV L2 vaccine induces broad cross-reactive humoral immune responses. Vaccine. 2018;36(30):4462–70. doi:10.1016/j.vaccine.2018.06.024.
- Nieto K, Weghofer M, Sehr P, Ritter M, Sedlmeier S, Karanam B, Seitz H, Müller M, Kellner M, Hörer M, et al. Development of AAVLP(HPV16/31L2) particles as broadly protective HPV vaccine candidate. PLoS One. 2012;7(6):e39741. doi:10.1371/journal.pone.0039741.
- Jiang RT, Schellenbacher C, Chackerian B, Roden RBS. Progress and prospects for L2-based human papillomavirus vaccines. Expert Rev Vaccines. 2016;15(7):853–62. doi:10.1586/14760584.2016.1157479.
- Olczak P, Roden RBS. Progress in L2-based prophylactic vaccine development for protection against diverse human papillomavirus genotypes and associated diseases. Vaccines (Basel). 2020;8(4). doi:10.3390/vaccines8040568.
- Schellenbacher C, Roden R, Kirnbauer R. Chimeric L1-L2 virus-like particles as potential broad-spectrum human papillomavirus vaccines. J Virol. 2009;83(19):10085–95. doi:10.1128/JVI.01088-09.
- Schellenbacher C, Kwak K, Fink D, Shafti-Keramat S, Huber B, Jindra C, Faust H, Dillner J, Roden RBS, Kirnbauer R, et al. Efficacy of RG1-VLP vaccination against infections with genital and cutaneous human papillomaviruses. J Invest Dermatol. 2013;133(12):2706–13. doi:10.1038/jid.2013.253.
- Powell BS, Andrianov AK, Fusco PC. Polyionic vaccine adjuvants: another look at aluminum salts and polyelectrolytes. Clin Exp Vaccine Res. 2015;4(1):23–45. doi:10.7774/cevr.2015.4.1.23.
- Andrianov AK. Polyphosphazenes as vaccine adjuvants. In: Singh M, editor. Vaccine adjuvants and delivery systems. Hoboken (New Jersey): John Wiley & Sons; 2007. p. 355–78.
- Magiri R, Mutwiri G, Wilson HL. Recent advances in experimental polyphosphazene adjuvants and their mechanisms of action. Cell Tissue Res. 2018;374(3):465–71. doi:10.1007/s00441-018-2929-4.
- Istrate C, Hinkula J, Charpilienne A, Poncet D, Cohen J, Svensson L, Johansen K. Parenteral administration of RF 8-2/6/7 rotavirus-like particles in a one-dose regimen induce protective immunity in mice. Vaccine. 2008;26(35):4594–601. doi:10.1016/j.vaccine.2008.05.089.
- Andrianov AK, Marin A, Fuerst TR. Molecular-level interactions of polyphosphazene immunoadjuvants and their potential role in antigen presentation and cell stimulation. Biomacromolecules. 2016;17(11):3732–42. doi:10.1021/acs.biomac.6b01251.
- Andrianov AK, Marin A, Roberts BE. Polyphosphazene polyelectrolytes: a link between the formation of noncovalent complexes with antigenic proteins and immunostimulating activity. Biomacromolecules. 2005;6(3):1375–79. doi:10.1021/bm049329t.
- Cayatte C, Marin A, Rajani GM, Schneider-Ohrum K, Snell Bennett A, Marshall JD, Andrianov AK. PCPP-adjuvanted Respiratory Syncytial Virus (RSV) sF subunit vaccine: self-assembled supramolecular complexes enable enhanced immunogenicity and protection. Mol Pharm. 2017;14(7):2285–93. doi:10.1021/acs.molpharmaceut.7b00118.
- Andrianov AK, Marin A, Wang R, Chowdhury A, Agnihotri P, Yunus AS, Pierce BG, Mariuzza RA, Fuerst TR. In vivo and in vitro potency of polyphosphazene immunoadjuvants with hepatitis C virus antigen and the role of their supramolecular assembly. Mol Pharm. 2020. doi:10.1021/acs.molpharmaceut.0c00487.
- Andrianov AK, Svirkin YY, LeGolvan MP. Synthesis and biologically relevant properties of polyphosphazene polyacids. Biomacromolecules. 2004;5(5):1999–2006. doi:10.1021/bm049745d.
- Andrianov AK, Marin A, Chen J. Synthesis, properties, and biological activity of poly[di(sodium carboxylatoethylphenoxy)phosphazene]. Biomacromolecules. 2006;7(1):394–99. doi:10.1021/bm050790a.
- Wang JW, Matsui K, Pan Y, Kwak K, Peng S, Kemp T, Pinto L, Roden RB. Production of furin-cleaved papillomavirus pseudovirions and their use for in vitro neutralization assays of L1- or L2-specific antibodies. Curr Protoc Microbiol. 2015;38:14B 5 1–26.
- Wang JW, Jagu S, Kwak K, Wang C, Peng S, Kirnbauer R, Roden RBS. Preparation and properties of a papillomavirus infectious intermediate and its utility for neutralization studies. Virology. 2014;449:304–16. doi:10.1016/j.virol.2013.10.038.
- Yessine MA, Leroux JC. Membrane-destabilizing polyanions: interaction with lipid bilayers and endosomal escape of biomacromolecules. Adv Drug Deliv Rev. 2004;56(7):999–1021. doi:10.1016/j.addr.2003.10.039.
- Lackey CA, Murthy N, Press OW, Tirrell DA, Hoffman AS, Stayton PS. Hemolytic activity of pH-responsive polymer-streptavidin bioconjugates †. Bioconjug Chem. 1999;10(3):401–05. doi:10.1021/bc980109k.
- Rozema DB, Ekena K, Lewis DL, Loomis AG, Wolff JA. Endosomolysis by masking of a membrane-active agent (EMMA) for cytoplasmic release of macromolecules. Bioconjug Chem. 2003;14(1):51–57. doi:10.1021/bc0255945.
- Knudsen NP, Olsen A, Buonsanti C, Follmann F, Zhang Y, Coler RN, Fox CB, Meinke A, D´Oro U, Casini D, et al. Different human vaccine adjuvants promote distinct antigen-independent immunological signatures tailored to different pathogens. Sci Rep. 2016;6:19570. doi:10.1038/srep19570.
- Palmer CD, Ninković J, Prokopowicz ZM, Mancuso CJ, Marin A, Andrianov AK, Dowling DJ, Levy O. The effect of stable macromolecular complexes of ionic polyphosphazene on HIV Gag antigen and on activation of human dendritic cells and presentation to T-cells. Biomaterials. 2014;35(31):8876–86. doi:10.1016/j.biomaterials.2014.06.043.
- Andrianov AK, Marin A, Fuerst TR. Self-assembly of polyphosphazene immunoadjuvant with poly(ethylene oxide) enables advanced nanoscale delivery modalities and regulated pH-dependent cellular membrane activity. Heliyon. 2016;2(4):e00102. doi:10.1016/j.heliyon.2016.e00102.
- Awate S, Wilson HL, Lai K, Babiuk LA, Mutwiri G. Activation of adjuvant core response genes by the novel adjuvant PCEP. Mol Immunol. 2012;51(3–4):292–303. doi:10.1016/j.molimm.2012.03.026.
- Awate S, Wilson HL, Singh B, Babiuk LA, Mutwiri G. The adjuvant PCEP induces recruitment of myeloid and lymphoid cells at the injection site and draining lymph node. Vaccine. 2014;32(21):2420–27. doi:10.1016/j.vaccine.2014.03.014.
- Mutwiri G, Benjamin P, Soita H, Babiuk LA. Co-administration of polyphosphazenes with CpG oligodeoxynucleotides strongly enhances immune responses in mice immunized with Hepatitis B virus surface antigen. Vaccine. 2008;26(22):2680–88. doi:10.1016/j.vaccine.2008.03.031.
- Garlapati S, Eng NF, Kiros TG, Kindrachuk J, Mutwiri GK, Hancock REW, Halperin SA, Potter AA, Babiuk LA, Gerdts V, et al. Immunization with PCEP microparticles containing pertussis toxoid, CpG ODN and a synthetic innate defense regulator peptide induces protective immunity against pertussis. Vaccine. 2011;29(38):6540–48. doi:10.1016/j.vaccine.2011.07.009.
- Andrianov AK, Marin A, Wang R, Karauzum H, Chowdhury A, Agnihotri P, Yunus AS, Mariuzza RA, Fuerst TR. Supramolecular assembly of toll-like receptor 7/8 agonist into multimeric water-soluble constructs enables superior immune stimulation in vitro and in vivo. ACS Appl Bio Mater. 2020;3(5):3187–95. doi:10.1021/acsabm.0c00189.
- Andrianov AK, Le Golvan MP. Characterization of poly [di (carboxylatophenoxy)‐phosphazene] by an aqueous gel permeation chromatography. J Appl Polym Sci. 1996;60(12):2289–95. doi:10.1002/(SICI)1097-4628(19960620)60:12<2289::AID-APP28>3.0.CO;2-0.
- Andrianov AK. Self-assembling ionic polyphosphazenes and their biomedical applications. In: Andrianov AK, Allcock HR, editors. Polyphosphazenes in biomedicine, engineering, and pioneering synthesis. ACS Symposium Series. Vol. 1298.Washington, DC: American Chemical Society; 2018. p. 27–49. doi:10.1021/bk-2018-1298.ch002
- Andrianov AK, Marin A, Deng J, Fuerst TR. Protein-loaded soluble and nanoparticulate formulations of ionic polyphosphazenes and their interactions on molecular and cellular levels. Mater Sci Eng C Mater Biol Appl. 2020;106:110179. doi:10.1016/j.msec.2019.110179.
- Selin V, Albright V, Ankner JF, Marin A, Andrianov AK, Sukhishvili SA. Biocompatible nanocoatings of fluorinated polyphosphazenes through aqueous assembly. ACS Appl Mater Interfaces. 2018;10(11):9756–64. doi:10.1021/acsami.8b02072.
- Albright V, Marin A, Kaner P, Sukhishvili SA, Andrianov AK. New family of water-soluble sulfo–fluoro polyphosphazenes and their assembly within hemocompatible nanocoatings. ACS Appl Bio Mater. 2019;2(9):3897–906. doi:10.1021/acsabm.9b00485.
- Manzenrieder F, Luxenhofer R, Retzlaff M, Jordan R, Finn MG. Stabilization of virus‐like particles with poly (2‐oxazoline) s. Angewandte Chem. 2011;123(11):2649–53. doi:10.1002/ange.201006134.
- Lee PW, Isarov SA, Wallat JD, Molugu SK, Shukla S, Sun JEP, Zhang J, Zheng Y, Lucius Dougherty M, Konkolewicz D, et al. Polymer structure and conformation alter the antigenicity of virus-like particle-polymer conjugates. J Am Chem Soc. 2017;139(9):3312–15. doi:10.1021/jacs.6b11643.
- Mutwiri G, Benjamin P, Soita H, Townsend H, Yost R, Roberts B, Andrianov AK, Babiuk LA. Poly[di(sodium carboxylatoethylphenoxy)phosphazene] (PCEP) is a potent enhancer of mixed Th1/Th2 immune responses in mice immunized with influenza virus antigens. Vaccine. 2007;25(7):1204–13. doi:10.1016/j.vaccine.2006.10.011.
- Andrianov AK, Chen J, Payne LG. Preparation of hydrogel microspheres by coacervation of aqueous polyphosphazene solutions. Biomaterials. 1998;19(1–3):109–15. doi:10.1016/S0142-9612(97)00227-5.
- Shen H, Ackerman AL, Cody V, Giodini A, Hinson ER, Cresswell P, Edelson RL, Saltzman WM, Hanlon DJ. Enhanced and prolonged cross-presentation following endosomal escape of exogenous antigens encapsulated in biodegradable nanoparticles. Immunology. 2006;117(1):78–88. doi:10.1111/j.1365-2567.2005.02268.x.
- Stier EM, Mandal M, Lee KD. Differential cytosolic delivery and presentation of antigen by listeriolysin O-liposomes to macrophages and dendritic cells. Mol Pharm. 2005;2(1):74–82. doi:10.1021/mp049896v.
- Lin ML, Zhan Y, Villadangos JA, Lew AM. The cell biology of cross-presentation and the role of dendritic cell subsets. Immunol Cell Biol. 2008;86(4):353–62. doi:10.1038/icb.2008.3.
- Fausch SC, Da Silva DM, Kast WM. Differential uptake and cross-presentation of human papillomavirus virus-like particles by dendritic cells and Langerhans cells. Cancer Res. 2003;63:3478–82.
- Hogenesch H. Mechanism of immunopotentiation and safety of aluminum adjuvants. Front Immunol. 2012;3:406. doi:10.3389/fimmu.2012.00406.
- Hjelholt A, Christiansen G, Sørensen US, Birkelund S. IgG subclass profiles in normal human sera of antibodies specific to five kinds of microbial antigens. Pathog Dis. 2013;67(3):206–13. doi:10.1111/2049-632X.12034.
- Aalberse RC, Platts-Mills TA, Rispens T. The developmental history of IgE and IgG4 antibodies in relation to atopy, eosinophilic esophagitis, and the modified TH2 response. Curr Allergy Asthma Rep. 2016;16(6):45. doi:10.1007/s11882-016-0621-x.
- Spazierer D, Skvara H, Dawid M, Fallahi N, Gruber K, Rose K, Lloyd P, Heuerding S, Stingl G, Jung T, et al. T helper 2 biased de novo immune response to keyhole limpet hemocyanin in humans. Clin Exp Allergy. 2009;39(7):999–1008. doi:10.1111/j.1365-2222.2008.03177.x.
- Terhune TD, Deth RC. Aluminum adjuvant-containing vaccines in the context of the hygiene hypothesis: a risk factor for eosinophilia and allergy in a genetically susceptible subpopulation? Int J Environ Res Public Health. 2018;15(5):901. doi:10.3390/ijerph15050901.
- Eng NF, Garlapati S, Gerdts V, Babiuk LA, Mutwiri GK. PCEP enhances IgA mucosal immune responses in mice following different immunization routes with influenza virus antigens. J Immune Based Ther Vaccines. 2010;8:4. doi:10.1186/1476-8518-8-4.
- Awate S, Babiuk LA, Mutwiri G. Mechanisms of action of adjuvants. Front Immunol. 2013;4:114. doi:10.3389/fimmu.2013.00114.
- Rubio I, Seitz H, Canali E, Sehr P, Bolchi A, Tommasino M, Ottonello S, Müller M. The N-terminal region of the human papillomavirus L2 protein contains overlapping binding sites for neutralizing, cross-neutralizing and non-neutralizing antibodies. Virology. 2011;409(2):348–59. doi:10.1016/j.virol.2010.10.017.
- Wang JW, Wu WH, Huang T-C, Wong M, Kwak K, Ozato K, Hung C-F, Roden RBS. Roles of Fc domain and exudation in L2 antibody-mediated protection against human papillomavirus. J Virol. 2018;92(15). doi:10.1128/JVI.00572-18.
- Boxus M, Fochesato M, Miseur A, Mertens E, Dendouga N, Brendle S, Balogh KK, Christensen ND, Giannini SL, et al. Broad cross-protection is induced in preclinical models by a human papillomavirus vaccine composed of L1/L2 chimeric virus-like particles. J Virol. 2016;90(14):6314–25. doi:10.1128/JVI.00449-16.
- Andrianov AK, Decollibus DP, Marin A, Webb A, Griffin Y, Webby RJ. PCPP-formulated H5N1 influenza vaccine displays improved stability and dose-sparing effect in lethal challenge studies. J Pharm Sci. 2011;100(4):1436–43. doi:10.1002/jps.22367.
- Hassett KJ, Meinerz NM, Semmelmann F, Cousins MC, Garcea RL, Randolph TW. Development of a highly thermostable, adjuvanted human papillomavirus vaccine. Eur J Pharm Biopharm. 2015;94:220–28. doi:10.1016/j.ejpb.2015.05.009.