ABSTRACT
Respiratory syncytial virus (RSV) infection caused severe acute respiratory disease in children and the elderly. There is no licensed vaccine. It has been a challenging problem to avoid vaccine enhanced respiratory disease in developing a safe and effective RSV vaccine. Here, we investigated the impact of MF59-like oil-in-water emulsion adjuvant Addavax on the vaccine efficacy of inactivated split RSV (sRSV) and the roles of natural killer (NK) cells in enhanced respiratory disease in sRSV vaccinated mice after RSV infection. Addavax-adjuvanted sRSV vaccination induced higher levels of IgG1 isotype antibodies and more effective lung viral clearance upon RSV infection but promoted enhanced respiratory disease of weight loss, pulmonary inflammation, and NK and NK T (NKT) cell infiltrations in the lungs. Antibody treatment depleting NK cells prior to RSV infection resulted in preventing severe weight loss and histopathology, as well as attenuating infiltration of dendritic cell subsets and TNF-α+ T cells in the lungs. This study demonstrated the impacts of oil-in-water emulsion adjuvant on sRSV vaccination and the potential roles of NK and NKT cells in protection and respiratory disease after adjuvanted RSV vaccination and infection in a mouse model.
Introduction
Respiratory syncytial virus (RSV) causes upper and lower respiratory disease in infants, children and the elderly. However, no vaccine against RSV has been licensed yet. One of the challenges in developing a safe and effective RSV vaccine is to avoid vaccine enhanced RSV disease as observed after formalin-inactivated RSV (FI-RSV) vaccination. FI-RSV vaccination induced T helper (Th) 2 type immune responses, severe lung inflammation and eosinophil infiltration in the lungs upon RSV infection. In the 1960s, alum-adjuvanted FI-RSV–immunized individuals experienced severe RSV diseases during RSV epidemic season and two children died of enhanced respiratory disease. Therefore, safety concerns of enhanced respiratory diseases should be addressed in developing a new RSV vaccine.Citation1–3
A split form of viral vaccines has been widely used in commercial vaccines against seasonal influenza.Citation4,Citation5 The virus is inactivated and then disrupted by detergent to retain viral proteins but no replication capacity. In a previous study, split RSV (sRSV) vaccine has been tested in a BALB/c mice model to determine its immunogenicity and safety. Vaccination with sRSV elicited both Th1 and Th2 immune responses and less histopathological changes in lungs than FI-RSV vaccination in BALB/c mice after RSV challenge, suggesting that sRSV could be an RSV vaccine candidate.Citation6
An adjuvant in the subunit or inactivated virus vaccine formulation is common to induce stronger vaccine antigen-specific immune responses and to modulate T cell responses. Aluminum hydroxide (Alum) is the most widely used vaccine adjuvant in human and animal vaccines for more than 80 y but has been known to induce Th2-biased immune responses. MF59 is a squalene oil-in-water emulsion type commercial vaccine adjuvant developed by Novartis and has been licensed for use in seasonal influenza vaccines for the elderly and the 2009 pandemic influenza vaccines.Citation7,Citation8 It is known that MF59 adjuvant can elicit both Th1 and Th2 immune responses and strong vaccine antigen-specific antibody production in a CD4 T cell-dependent and independent manner.Citation9 Addavax is an MF59-like adjuvant and has been tested with various vaccine candidates including hepatitis C virus, human immunodeficiency virus, beta–coronaviruses, and bacterial vaccines.Citation10–13 However, Addavax has not been evaluated with a new RSV vaccine candidate, sRSV to determine its adjuvant efficacy, protection against RSV, and possibility of causing RSV vaccine enhanced diseases.
Th2-biased alum adjuvant in FI-RSV vaccination is likely a factor contributing to enhanced respiratory disease after RSV infection and has been used as a control to mimic a clinical outcome of enhanced respiratory disease in preclinical studies.Citation14–16 Many other licensed adjuvants, such as QS21, Addavax, liposomes, monophosphotyl lipid A (MPL), and oligonucleotide CpG, also evaluated their adjuvant effects in inducing immune responses specific to RSV antigens.Citation17,Citation18 However, the cellular components responsible for RSV vaccine enhanced disease remain to be further defined. In addition, it is less well understood whether the more potent licensed adjuvants (MF59 or MF59-like Addavax) would exhibit effects on enhancing protection or lung inflammatory disease after RSV vaccination and challenge. In this study, we have investigated what effects MF59-like Addavax adjuvant would exert on sRSV vaccination and cross-talks between natural killer (NK) cells and other cellular infiltrates contributing to enhanced disease after RSV infection. We found that Addavax-adjuvanted sRSV vaccination increased IgG antibody responses and lung viral clearance upon RSV infection but elicited severe respiratory disease. Results in this study support that NK cells play a major role in causing Addavax-adjuvanted sRSV vaccine enhanced disease and lung inflammation after RSV infection.
Material and methods
Cells, virus and antigens
HEp-2 cells were purchased from the American Type Culture Collection (ATCC, Rockville, MD, USA) and maintained in Dulbecco’s modified Eagle’s medium (DMEM; GIBCO-BRL, Grand Island, NY) with 10% fetal bovine serum (FBS, GIBCO-BRL), 2 mM glutamine, penicillin and streptomycin (GIBCO-BRL) at 37℃ with 5% CO2. RSV A2 strain was kindly provided by Dr Martin Moore (Emory University, GA) and propagated in HEp-2 cells. RSV-infected HEp-2 cells were cultured for 3 d, harvested and centrifuged for 10 min at 2000 rpm in a table-top centrifuge at 4℃. Collected RSV within supernatants was inactivated by incubating with 10% formalin (1:4000 vol/vol) for 3 d at 37℃.Citation19 Then, the formalin-inactivated RSV (FI-RSV) was purified by ultracentrifugation for 60 min at 30,000 rpm. Splitting of FI-RSV to prepare Split RSV (sRSV) was carried out by treating with detergent 1% Triton-x 100 (Sigma Aldrich) in Phosphate buffered saline (PBS) for 2 h at 20℃.Citation20 The detergent was removed by dialysis cassette (10,000 MWCO, Thermo scientific) floating in PBS.
Animals, immunization, RSV infection
BALB/c mice were purchased from Charles River Laboratories and maintained in the animal facility at Georgia State University (GSU). All mouse experiments in this study were performed under the guidelines of a GSU-approved institutional animal care and use committee (IACUC) protocol (protocol A18001). BALB/c mice at adult age (6–8 weeks old) were one-time immunized intramuscularly with sRSV (5 μg) alone or with Addavax (50%) or PBS (naïve control). To determine serum IgG levels, blood samples were collected 2 weeks later after prime immunization. Naïve control and immunized mice were intranasally challenged with RSV A2 (4 × 105 PFU in 50 μl of PBS per mouse) under isoflurane anesthesia at 4 weeks after prime immunization and body weight changes monitored daily. At 5 d after challenge, we collected individual lung samples to examine lung inflammation and cell recruitments.
In vivo NK cell depletion
For NK cell depletion, mice were given intraperitoneal injections two times with 50 µl of anti-asialo GM1 or control rabbit IgG in 200 µl PBS at 2 d and 1 h prior to RSV infection.Citation21,Citation22
ELISA assay
RSV specific IgG isotype antibodies (IgG, IgG1, and IgG2a) were measured in serum samples by enzyme-linked immunosorbent assay (ELISA) as previously described.Citation14 The 96-well ELISA plates were coated with inactivated RSV (4 μg/ml). Isotype antibodies were detected using horseradish peroxidase (HRP)-conjugated anti-goat IgG, IgG1 and IgG2a secondary antibodies (Southern Biotechnology) after blocking and sample treatment. Cytokine levels in lung homogenates were measured by commercially available ELISA kits (R&D systems). The substrate (TMB, 3,3′,5,5′-tetramethylbenzidine, Sigma Aldrich) was treated and then stopped with 1M H3PO4. Optical densities (O.D.) were read at 450 nm.
RSV immuno-plaque assay
RSV titer was measured in individual lung samples day 5 postchallenge. The serially diluted lung homogenates were added into the monolayer HEp-2 cells and adsorbed for 2 h, then overlaid with growth medium containing 0.8% low-temperature gelling agarose prior to incubation for 3 d. After fixation in formalin, the plaques were detected using the primary anti-RSV F monoclonal (131-2a) antibody,Citation23 secondary anti-mouse IgG antibody- horse radish peroxidase (HRP) conjugate. As for HRP substrate, 3,3ʹdiaminobenzidine (0.5 mg/ml DAB with 0.01% H2O2) was used. The viral titer detection limit was approximately 40 PFU from lung samples in this assay.
Flow cytometry and intracellular cytokine staining
The lung cells were prepared by homogenization and Percoll gradients (44% and 67%) centrifugation. The contaminated red blood cells were lysed by using ammonium chloride potassium buffer. The lung cells were divided to two, one for surface marker staining and another for intracellular cytokine staining. For the surface marker staining, the cells were stained with CD45 (clone 30-F11), CD11b (clone M1/70), CD11c (clone N418), F4/80 (clone BM8), Ly6c (clone HK1.4), MHC class II (clone M5/114.15.2), Siglec F (clone E50-2440), B220 (clone RA3-6B2), CD103 (clone 2E7) antibodies. For the intracellular cytokine staining, the cells stimulated with RSV F85-93 (KYKNAVTEL) specific for CD8 T cells and G183-195 (WAICKRIPNKKPG) peptides specific for CD4 T cells,Citation24,Citation25 and BD GolgiStop at 37℃ for 5 h, stained with the surface marker antibodies, CD3 (clone 17A2), CD4 (clone GK1.5), and CD8 (clone 53–6.7), and then fixed and permeabilized according to the manufacturer’s instructions (Fixation/Permeabilization Solution Kit, BD Biosciences). Intracellular cytokine antibodies were added to the permeabilized cells. For analysis, the Becton–Dickinson LSR-II/Fortessa flow cytometer (BD, San Diego, CA) was used to collect cell populations. Acquired flow cytometry data were analyzed by using Flowjo software (Tree Star Inc.).
Histopathology
Lung tissues collected from mice at 5 d after RSV challenge were fixed with 10% neutral buffered-formalin. Lung tissue histology was performed by staining with hematoxylin and eosin (H&E), periodic acid–Schiff (PAS), and hematoxylin and Congo red (H&CR) and analyzed under light microscopy. The tissue slides were examined for lymphocytes and eosinophils in peribronchiolar, perivascular, interstitial, and alveolar spaces. At least 10 sections per lung tissue from individual mouse were obtained and blind scoring was performed for histopathology analysis as detailed in our previous studies.Citation26,Citation27
Statistical analysis
Statistical differences were performed using GraphPad statistical software (GraphPad software Inc., San Diego, CA). Data were analyzed for significance using one-way or two-way ANOVA with Tukey’s multiple comparison test for multiple comparisons. The difference was considered statistically significant when the p value was less than 0.05.
Results
Addavax-adjuvanted sRSV vaccination induced pulmonary inflammation upon RSV infection despite high antibody levels and effective viral clearance
To determine the adjuvant effects of Addavax on RSV vaccination, BALB/c mice were intramuscularly immunized with sRSV in the presence or absence of Addavax. The immune sera were collected week 2 postimmunization to measure RSV-specific antibody levels. The sRSV+Addavax group elicited significantly higher RSV-specific IgG and IgG1 antibody levels in sera compared to those in the sRSV group ( and B). However, the RSV-specific IgG2a levels were comparable between sRSV only and sRSV+Addavax groups (). At 4 weeks after prime immunization, the mice were challenged with RSV to investigate the protective efficacy of sRSV+Addavax vaccination. The virus replication was fully inhibited in the lungs of the sRSV+Addavax immunized mice day 5 post-RSV infection (). However, despite higher antibody levels in sera and effective lung viral clearance, the sRSV+Addavax immunized group showed up substantial weight loss (15%), which is higher than 5% in the sRSV-only group and 10% in the naïve-infected group after RSV infection. A peak in weight loss was observed day 2 postinfection in sRSV only and sRSV+Addavax groups, but naïve infected group lost weight until day 3 postinfection. The sRSV+Addavax group could not recover original body weight until day 5 postinfection, when they were sacrificed. The other groups were better at recovering their body weights (). In addition, compared to the sRSV-immunized and the naïve-infected groups, more severe lung inflammation and mucus production were observed in the sRSV+Addavax immunized group (). These data suggest that the presence of Addavax in sRSV immunization-enhanced RSV-specific serum antibody production and lung viral clearance but elicited severe lung inflammation and body weight loss upon RSV infection.
Figure 1. Pulmonary inflammation caused by Addavax-adjuvanted sRSV vaccination upon RSV infection. Balb/c mice (n = 5) were immunized intramuscularly with sRSV with or without Addavax and 3 weeks after immunization infected with 4 × 105 PFU of RSV A2 strain. (A–C) RSV-specific antibody in the immunized mice sera. The immune sera were collected at week 2 postimmunization. RSV-specific IgG, IgG1, IgG2a were measured by ELISA. (D) RSV titers from the infected mice. The lung samples were harvested at day 5 post-RSV infection. One-way ANOVA and Tukey’s multiple comparison tests were performed. ***; p < .0002, and ****; p < .0001. (E) Daily body weight monitoring after RSV infection. Two-way ANOVA and Tukey’s multiple comparison tests were performed. *; p < .0332 between sRSV and sRSV+Addavax. (F) Lung histopathology and mucus production of the immunized mice. The lung tissues were collected at day 5 post-RSV infection, processed, and stained with hematoxylin&eosin (H&E) or periodic acid-Schiff (PAS). Scale bars represent 100 μm (×100 magnification). Arrows indicate mucus infiltrated regions
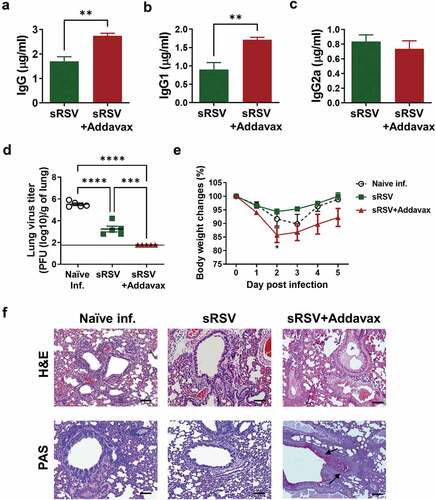
NK cell depletion from the sRSV+Addavax immunized mice mitigated weight-loss disease after RSV infection
RSV infection induced recruitment of NK cells into the lung in the naïve group and Addavax-adjuvanted sRSV vaccine group (). Addavax-adjuvanted sRSV group also significantly enhanced NKT cell infiltration to the lungs upon RSV challenge (). This observation provided rational data supporting the focus on the role of NK cells in vaccine-enhanced RSV diseases in this study. To determine the role of NK cells in Addavax-enhanced RSV disease, we depleted NK cells by injecting anti-asialo GM1 monoclonal antibodies intraperitoneally to the sRSV+Addavax immunized mice prior to RSV infection ().Citation21 Anti-asialo GM1 antibodies depleted NK cells mainly, but NKT cells were also partially affected in vivo by this antibody treatment, consistent with previous studies.Citation22,Citation28,Citation29 NK cell depletion was maintained at least until 5 d post-RSV infection, but a fraction of NKT cells remained in the lungs of the GM1-treated mice ( and C). Lung RSV titers were determined from the lung samples harvested day 5 postinfection, indicating that this NK cell depletion did not result in the RSV titers (). Body weight loss was minimized by NK depletion from the sRSV+Addavax immunized mice. The sRSV+Addavax group showed up to 17% of body weight loss until day 3 postinfection, whereas the NK-depleted sRSV+Addavax group lost only 9% of body weight until day 3 postinfection. Both groups showed weight recovery after day 3 postinfection (). These data suggest that NK cells played a role in inducing vaccine-enhanced weight loss by sRSV+Addavax immunization in a mouse model.
Figure 2. NK depletion from sRSV+Addavax immunized mice. (A) A brief schedule of immunization and NK depletion. A group of the sRSV+Addavax immunized mice (n = 5) was injected with anti-asialo GM1 monoclonal antibody intraperitoneally at −2 d and −1 h before RSV infection to deplete NK cells. (B and C) NK and NKT cells in lung at day 5 post-RSV infection. NK cells; CD3−CD49b+, NKT cells; CD3+CD49b+. One-way ANOVA and Tukey’s multiple comparison tests were performed. *; p < .0332, and ***; p < .0002. (D) Lung RSV titers at day 5 post-RSV infection. (E) Body weight changes of the RSV-infected mice. Two-way ANOVA and Tukey’s multiple comparison tests were performed. *; p < .0332 between sRSV+Addavax and sRSV+Addavax+GM1
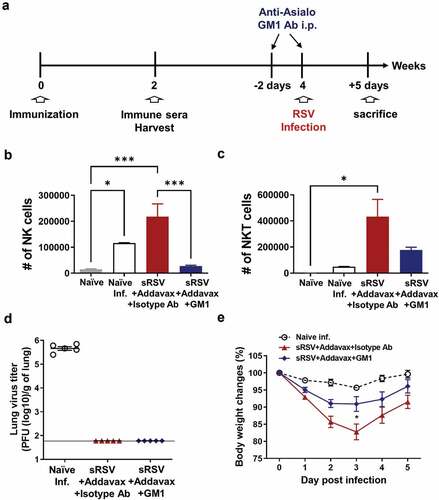
NK cell depletion reduced lung inflammation in sRSV+Addavax immunized mice
To further investigate the protective effects of NK cell depletion in sRSV+Addavax immunized mice, lung samples were harvested at day 5 post-RSV infection. The lungs were fixed, processed and stained with H&E for inflammation scoring and PAS for mucus staining. As previously observed (), severe lung inflammation was observed in the naïve RSV-infected group and sRSV+Addavax group. The NK-depleted sRSV+Addavax group showed lower levels in lung inflammation (). Inflammation scores in the airways, blood vessels, and interstitial spaces of the lungs from the sRSV+Addavax group were significantly higher than those in the NK-depleted sRSV+Addavax and naïve infected groups (–D). Mucus production was highly elevated in the sRSV+Addavax group, whereas NK cell depletion resulted in a significantly lower level of mucus production (). Taken together, NK cells appeared to have played a significant role in enhancing lung inflammation and mucus production resulting from sRSV+Addavax immunization.
Figure 3. Less pulmonary inflammation in NK depleted sRSV+Addavax immunized mice after RSV infection. (A) Representative photographic pictures for histopathology and mucus production in lung. The lung tissues were collected from mice (n = 5) day 5 post-RSV infection and stained with H&E or PAS. Scale bars represent 100 μm (×100 magnification). (B–D) Inflammation scores around the airways, blood vessels, and interstitial spaces, respectively. (E) Mucus production in the RSV-infected mice (n = 5). PAS positive percentages were calculated. One-way ANOVA and Tukey’s multiple comparison tests were performed. *; p < .0332, **; p < .0021, ***; p < .0002
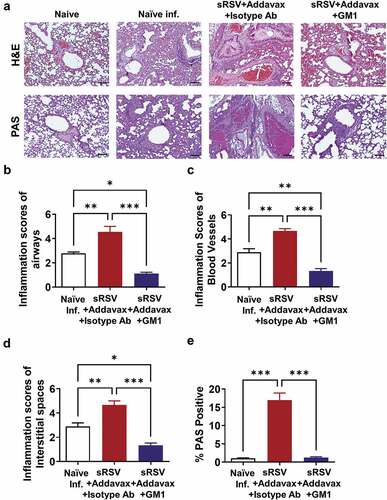
Severe eosinophil infiltration in the lungs is one of the main contributors to vaccine-enhanced RSV disease.Citation14,Citation26 The recruited eosinophils aggravate lung inflammation due to RSV infection.Citation26,Citation27 The sRSV+Addavax immunization enhanced eosinophil recruitment in the lung after RSV infection, but NK cell depletion prevented eosinophil infiltration (). Not only was the number of eosinophils decreased by NK cell depletion but also the total infiltrating cell numbers of lungs were significantly reduced in NK-depleted sRSV+Addavax mice. This result suggests that NK cell depletion reduced inflammatory infiltrates in lung ( and C).
Figure 4. Lower eosinophil infiltration in NK depleted sRSV+Addavax immunized mice. (A) Eosinophils stained in the lung tissues. The lung tissues were collected from mice (n = 5) at day 5 post-RSV infection and stained with hematoxylin and Congo red (H&CR). Scale bars represent 100 μm (×100 magnification). The insets indicate infiltrated eosinophils (x400 magnification). (B) Total cell numbers of the RSV-infected lungs were counted and calculated by trypan blue staining under a microscope. (C) The number of eosinophil in lung. Eosinophil population (CD11b+SiglecF+) was determined by flow cytometry and the number was calculated by multiplying the eosinophil percentages with the total cell numbers. One-way ANOVA and Tukey’s multiple comparison tests were performed. *; p < .0332
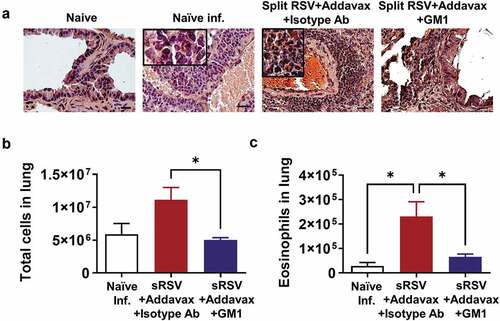
Dendritic cell recruitments were affected by NK cell depletion from sRSV+Addavax immunized mice
To determine the effects of NK cell depletion from the sRSV+Addavax immunized mice on recruiting other infiltrates in the lungs, the cellular phenotypes and cellularity were analyzed by flow cytometry of lung cells. Alveolar macrophages were decreased in both sRSV+Addavax and NK-depleted sRSV+Addavax groups after RSV infection (). MHC-IIhigh activated macrophages, monocytes, and neutrophils were increased in lungs from the sRSV+Addavax immunized mice. Although not statistically significant, NK depletion resulted in reduced numbers of DC cell populations (–D). CD11c+ pan DCs, pDCs, CD11blow and CD11bhigh DCs in the sRSV+Addavax group were significantly increased by RSV infection, but NK cell depletion resulted in statistically significant reduction in these cell populations (–H). These data suggest that NK cells may play a role in recruiting different DC subset after sRSV+Addavax vaccination and RSV challenge.
Figure 5. Immune cell recruitment after RSV infection in the lungs of sRSV+Addavax immunized mice. The lung cells from mice (n = 5) were harvested day 5 post-RSV infection and their phenotypes and cellularity were determined by flow cytometry. Alveolar macrophages (AM); CD11b− CD11c+ F4/80+, MHC-IIhigh macrophages; CD11b− CD11c+ F4/80 + MHC-IIhigh, monocytes; CD11b + Ly6chigh F4/80+, Neutrophils; CD11b+ Ly6cint F4/80−, total dendritic cells (DCs); CD11c+ F4/80− MHC-II+, pDCs; B220+ in total DCs, CD11bhigh DCs; CD11bhigh in total DCs, CD11blow DCs; CD11blow in total DCs. One-way ANOVA and Tukey’s multiple comparison tests were performed. *; p < .0332, **; p < .0021, ***; p < .0002, ****; p < .0001
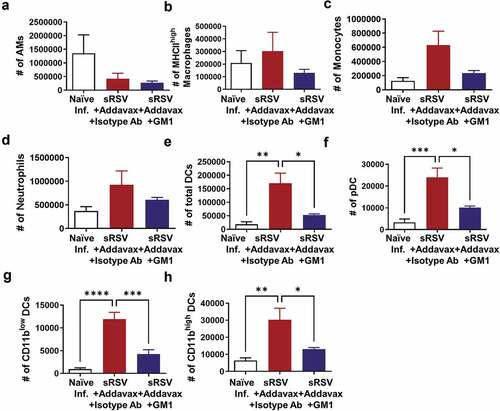
Antigen-specific CD4 and CD8 T cell recruitment into the lung was reduced by NK cell depletion in sRSV+Addavax immunized mice
NK cells also play a role in activating antigen-specific T cell responses by producing cytokines during virus infection.Citation30,Citation31 The antigen-specific T cell responses were determined by cytokine-producing CD4 and CD8 T cells after RSV peptide stimulation. The percentages of CD4 T cells producing cytokines (IL-4, IFN-γ, IL-13, and TNF-α) did not differ significantly between the groups with and without GM1 treatment (data not shown). Also, CD8 T cells producing TNF-α were similarly observed in both groups with (0.058%) and without GM1 treatment (0.032%). The percentage of CD8 T cells producing IFN- γ was observed to be approximately fivefold higher in the sRSV+Addavax group (0.062%) than that in the sRSV+Addavax+GM1 group (0.013%), suggesting the significant impact of NK cell depletion on CD8 T cells producing IFN-γ. Since the total effector cell numbers are more relevant in vivo than the relative proportions of the cells, the cytokine-producing cell numbers were presented in . TNF-α producing CD4 and CD8 T cells were significantly increased in the sRSV+Addavax immunized group after RSV infection, correlating with lung inflammation ( and E). The NK depleted sRSV+Addavax group showed statistically significant decreased CD4 T cells secreting TNF-α and IFN-γ cytokines (, D). TNF-α+ CD8 T and IFN-γ+ CD8 T cells were reduced by NK depletion, although statistical significance was observed only for TNF-α+ CD8 T cells ().
Figure 6. Antigen-specific T cell responses. The lung cells were harvested from mice (n = 5) day 5 post-RSV infection, stimulated with RSV F85-93 and G183-195 peptides, and stained with surface marker antibodies and intracellular cytokine antibodies. Cytokine-positive populations were determined by flow cytometry and the number was calculated by multiplying the percentages with the total cell numbers. One-way ANOVA and Tukey’s multiple comparison tests were performed. *; p < .0332, **; p < .0021, ***; p < .0002
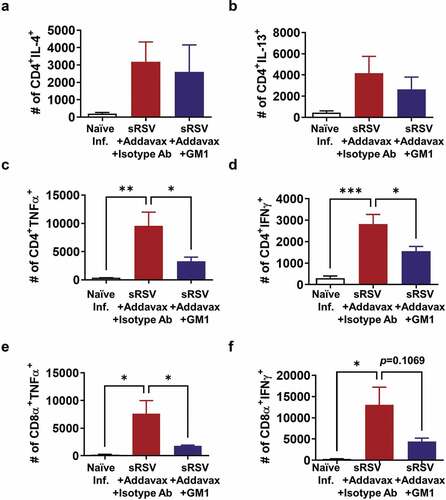
NK cell depletion causes decreased cytokine profiles in lung after RSV infection
sRSV+Addavax immunization caused significantly increased IL-13, TNF-α, and IFN-γ production in lung extract after RSV infection (). The anti-asialo GM1 antibody treatment reduced IL-13 cytokine significantly () and resulted in a pattern of reducing the TNF-α and IFN-γ cytokines although significance was not observed in TNF-α and IFN-γ levels. These data supported the role of NK cells in inducing inflammatory cytokines, particularly IL-13, correlating with eosinophils and enhanced RSV diseases by sRSV+Addavax immunization.
Discussion
Alum-adjuvanted FI-RSV vaccine-enhanced disease was shown to be mainly caused by Th2-biased immune responses, including IgG1 antibody production, IL-4, IL-5 and IL-13 cytokine production, and severe eosinophil infiltration in the lung.Citation14,Citation26,Citation27 Other adjuvants such as squalene oil-in-water emulsion, polysaccharides-based delta-inulin,Citation32 and NKT cell agonist α-GalCer also failed to attenuate RSV vaccine-associated inflammatory histopathology.Citation33,Citation34 Subunit protein RSV vaccines also have been reported to cause enhanced RSV disease, raising safety concerns.Citation35,Citation36 Therefore, different adjuvants and RSV vaccine platforms have the potential to induce enhanced respiratory disease after RSV infection, but mechanistic understanding is limited. MF59-like Addavax-adjuvanted sRSV vaccination induced higher levels of IgG1 isotype antibodies, suggesting the induction of Th2 immune responses and effective lung viral clearance after RSV infection. RSV titers in the nasal cavity and lungs were detected in a proportional correlative manner in previous studies,Citation37,Citation38 although viral titers in the nasal cavity were not determined in this study. Despite the effective viral clearance after RSV infection, enhanced respiratory disease was observed with symptoms including severe weight loss, pulmonary inflammation, and eosinophil infiltration in the lungs. In particular, we found significantly enhanced levels of NK and NKT cells infiltrated into the lungs from Addavax-adjuvanted sRSV-vaccinated mice after RSV infection. In this study, we wanted to test a hypothesis whether NK and NKT cells would play a critical role in enhanced respiratory disease in Addavax sRSV-vaccinated mice after RSV infection.
Ineffective viral clearance in the absence of RSV-specific IgG antibodies and moderate weight loss were observed in naïve mice, providing evidence for the role of these antibodies in viral clearance. During this RSV infection of naïve mice, a moderate level of NK cells was recruited into the lungs. NK cells can also elicit severe lung injury by inducing excessive cytokine production and killing the virus-infected epithelial cells. To date, the role of NK and NKT cells in RSV-induced disease and in adjuvanted RSV-vaccination disease enhancement has not been well studied. Compared to naïve and sRSV-vaccinated mice, the sRSV+Addavax-vaccinated mice induced the highest levels of NK and NKT cells in the lungs upon subsequent RSV infection, suggesting that infiltrated NK and NKT cells might have contributed to weight loss and lung inflammation.
Eosinophil infiltration is one of the hallmarks in vaccine-enhanced RSV disease. In this study, GM1 treatment depleted NK cells and partially NKT cells, which is correlating with lower levels of eosinophil infiltration after RSV infection in sRSV+Addavax immunized mice. There was no known direct relationship between NK cells and eosinophils. Increased infiltration levels of NK cells, NKT cells, and eosinophils were observed in the lung from the sRSV+Addavax immunized mice whereas eosinophil infiltration and enhanced RSV disease were significantly reduced in the sRSV+Addavax group with GM1 treatment. In addition, in , NK depletion significantly reduced the level of IL-13 correlating with reduced infiltration of eosinophils and DCs. Therefore, it is possible that NK cells and partially NKT cells together with producing cytokines might have modulated the infiltration of eosinophils, killing the infected cells and affecting DC recruitment and T cell responses.
The roles of NK cells in protection versus vaccine-enhanced diseases might be differentially dependent on the immune competency of the host. NK innate immune cells might play a significant role in protection by killing virus-infected cells via mechanisms of recognizing virus-infected cells and producing cytotoxic granules and cytokines in the absence of preexisting adaptive neutralizing antibodies.Citation39 A CD4-deficient condition induced lower levels of RSV-specific IgG antibodies, where NK cells played an important role in controlling lung viral loads and attenuating weight loss in FI-RSV+Alum immune mice after RSV infection.Citation40 Innate NKT cells contribute to early antiviral IFN-γ production and CD8 T cell expansion.Citation41 NKT cell agonist α-GalCer as a vaccine adjuvant was reported to aggravate RSV vaccine-associated inflammatory histopathology.Citation34 The findings in this study and the prior studies of viral clearance models suggest that, when adaptive humoral IgG responses were induced to control viral loads, excess recruitment and activation of innate NK and NKT cells likely contributed to cytokine-producing T cells and immunopathology of RSV vaccine-enhanced respiratory disease.
NK cells cross-talk with other immune cells, such as DC populations during viral infection. DCs secret IL-12 and IL-18 to activate NK cells and enhance NK cell cytolytic activity.Citation42,Citation43 Plasmacytoid DCs release type 1 IFN which can boost NK cell cytotoxicity.Citation42,Citation44 Moreover, NK cells also stimulate DC maturation by producing cytokines such as TNF-α and IFN-γ and direct engagement of NKp30, an NK cell activating receptor.Citation45 In this study, the numbers of CD11c+ pan DC and plasmacytoid and CD11blow/high DC cells were significantly decreased in the lungs of the NK-depleted sRSV+Addavax group after RSV infection (), indicating a decrease in DC maturation.
Taken together, this study demonstrated the impacts of squalene oil-in-water emulsion Addavax adjuvant on sRSV vaccination and the potential roles of NK and NKT cells in protection and vaccine-enhanced respiratory disease in a BALB/c mouse model. The results in this study support further evidence of cross-talks among NK and NKT cells and DC populations contributing to recruiting TNF-α+ CD4 and CD8 T cells and INF-γ+ CD4 T cells. Excess innate NK and DC cells and adaptive T cell immune responses even under a control of viral loads by humoral IgG responses might be contributing to immunopathology post-RSV infection.
Despite new findings in this study, treatment of NK cell depleting GM1 antibody resulted in off-target effects on reducing NKT cells, complicating the interpretation of the outcomes. Alum-adjuvanted FI-RSV vaccination enhanced respiratory disease was observed in naïve young children upon exposure to natural RSV infection, which is less likely in older children or adults who experienced with prior RSV infection. Humans are the only natural host for RSV infection. The weight loss is not represented as a symptom of vaccine-enhanced RSV disease in humans after RSV vaccination and infection. These differences between humans and mice limit the significance and need cautiousness in interpreting the experimental results from murine models. Nonetheless, MF59 oil-in-water adjuvant is safe and effective for use in licensed influenza vaccination. Further studies should focus on identifying adjuvants modulating immune responses avoiding away enhanced respiratory disease after RSV vaccination and infection.
Disclosure of potential conflicts of interest
No potential conflicts of interest were disclosed.
Additional information
Funding
References
- Kim HW, Canchola JG, Brandt CD, Pyles G, Chanock RM, Jensen K, Parrott RH. Respiratory syncytial virus disease in infants despite prior administration of antigenic inactivated vaccine. Am J Epidemiol. 1969;89(4):422–34. doi:10.1093/oxfordjournals.aje.a120955.
- Castilow EM, Olson MR, Varga SM. Understanding respiratory syncytial virus (RSV) vaccine-enhanced disease. Immunol Res. 2007;39:225–39. doi:10.1007/s12026-007-0071-6.
- Knudson CJ, Hartwig SM, Meyerholz DK, Varga SM. RSV vaccine-enhanced disease is orchestrated by the combined actions of distinct CD4 T cell subsets. PLoS Pathog. 2015;11:e1004757. doi:10.1371/journal.ppat.1004757.
- Beyer WE, Palache AM, Osterhaus AD. Comparison of serology and reactogenicity between influenza subunit vaccines and whole virus or split vaccines: a review and meta-analysis of the literature. Clin Drug Investig. 1998;15:1–12. doi:10.2165/00044011-199815010-00001.
- Soema PC, Kompier R, Amorij JP, Kersten GF. Current and next generation influenza vaccines: formulation and production strategies. Eur J Pharm Biopharm. 2015;94:251–63. doi:10.1016/j.ejpb.2015.05.023.
- Lee Y, Ko EJ, Kim KH, Lee YT, Hwang HS, Jung YJ, Jeeva S, Kwon Y-M, Seong BL, Kang SM, et al. The efficacy of inactivated split respiratory syncytial virus as a vaccine candidate and the effects of novel combination adjuvants. Antiviral Res. 2019;168:100–08. doi:10.1016/j.antiviral.2019.05.011.
- Del Giudice G, Rappuoli R, Didierlaurent AM. Correlates of adjuvanticity: a review on adjuvants in licensed vaccines. Semin Immunol. 2018;39:14–21. doi:10.1016/j.smim.2018.05.001.
- Reed SG, Orr MT, Fox CB. Key roles of adjuvants in modern vaccines. Nat Med. 2013;19:1597–608. doi:10.1038/nm.3409.
- Ko EJ, Lee YT, Kim KH, Jung YJ, Lee Y, Denning TL, Kang SM. Effects of MF59 adjuvant on induction of isotype-switched IgG antibodies and protection after immunization with T-dependent influenza virus vaccine in the absence of CD4+ T cells. J Virol. 2016;90:6976–88. doi:10.1128/JVI.00339-16.
- Akache B, Deschatelets L, Harrison BA, Dudani R, Stark FC, Jia Y, Landi A, Law JLM, Logan M, Hockman D, et al. Effect of different adjuvants on the longevity and strength of humoral and cellular immune responses to the HCV envelope glycoproteins. Vaccines (Basel). 2019;7. doi:10.3390/vaccines7040204.
- Jones DI, Pollara JJ, Johnson-Weaver BT, LaBranche CC, Montefiori DC, Pickup DJ, Permar SR, Abraham SN, Maddaloni M, Pascual DW, et al. Optimized mucosal modified vaccinia virus ankara prime/soluble gp120 boost HIV vaccination regimen induces antibody responses similar to those of an intramuscular regimen. J Virol. 2019;93:e00475–19.
- Dai L, Zheng T, Xu K, Han Y, Xu L, Huang E, An Y, Cheng Y, Li S, Liu M, et al. A universal design of betacoronavirus vaccines against COVID-19, MERS, and SARS. Cell. 2020;182(3):722–33 e11. doi:10.1016/j.cell.2020.06.035.
- Choubini E, Habibi M, Khorshidi A, Ghasemi A, Asadi Karam MR, Bouzari S. A novel multi-peptide subunit vaccine admixed with AddaVax adjuvant produces significant immunogenicity and protection against Proteus mirabilis urinary tract infection in mice model. Mol Immunol. 2018;96:88–97. doi:10.1016/j.molimm.2018.03.001.
- Ko EJ, Kwon YM, Lee JS, Hwang HS, Yoo SE, Lee YN, Lee Y-T, Kim M-C, Cho MK, Lee YR, et al. Virus-like nanoparticle and DNA vaccination confers protection against respiratory syncytial virus by modulating innate and adaptive immune cells. Nanomedicine. 2015;11:99–108. doi:10.1016/j.nano.2014.07.013.
- Kim KH, Lee YT, Hwang HS, Kwon YM, Jung YJ, Lee Y, Lee JS, Lee Y-N, Park S, Kang S-M, et al. Alum adjuvant enhances protection against respiratory syncytial virus but exacerbates pulmonary inflammation by modulating multiple innate and adaptive immune cells. PLoS ONE. 2015;10:e0139916. doi:10.1371/journal.pone.0139916.
- Hwang HS, Kim KH, Lee Y, Lee YT, Ko EJ, Park S, Lee JS, Lee B-C, Kwon Y-M, Moore ML, et al. Virus-like particle vaccines containing F or F and G proteins confer protection against respiratory syncytial virus without pulmonary inflammation in cotton rats. Hum Vaccin Immunother. 2017;13:1031–39. doi:10.1080/21645515.2016.1272743.
- Lee Y, Ko EJ, Kim KH, Lee YT, Hwang HS, Kwon YM, Graham BS, Kang SM. A unique combination adjuvant modulates immune responses preventing vaccine-enhanced pulmonary histopathology after a single dose vaccination with fusion protein and challenge with respiratory syncytial virus. Virology. 2019;534:1–13. doi:10.1016/j.virol.2019.05.010.
- Isaacs A, Li Z, Cheung STM, Wijesundara DK, McMillan CLD, Modhiran N, Young PR, Ranasinghe C, Watterson D, Chappell KJ, et al. Adjuvant selection for influenza and RSV prefusion subunit vaccines. Vaccines (Basel). 2021;9. doi:10.3390/vaccines9020071.
- Lee Y, Lee YT, Ko EJ, Kim KH, Hwang HS, Park S, Kwon Y-M, Kang SM. Soluble F proteins exacerbate pulmonary histopathology after vaccination upon respiratory syncytial virus challenge but not when presented on virus-like particles. Hum Vaccin Immunother. 2017;13:2594–605. doi:10.1080/21645515.2017.1362514.
- Kon TC, Onu A, Berbecila L, Lupulescu E, Ghiorgisor A, Kersten GF, Cui Y-Q, Amorij J-P, Van Der Pol L. Influenza vaccine manufacturing: effect of inactivation, splitting and site of manufacturing. comparison of influenza vaccine production processes. PLoS ONE. 2016;11(3):e0150700. doi:10.1371/journal.pone.0150700.
- Khater M, Macai J, Genyea C, Kaplan J. Natural killer cell regulation of age-related and type-specific variations in antibody responses to pneumococcal polysaccharides. J Exp Med. 1986;164:1505–15. doi:10.1084/jem.164.5.1505.
- Nishikado H, Mukai K, Kawano Y, Minegishi Y, Karasuyama H. NK cell-depleting anti-asialo GM1 antibody exhibits a lethal off-target effect on basophils in vivo. J Immunol. 2011;186:5766–71. doi:10.4049/jimmunol.1100370.
- Widjaja I, Wicht O, Luytjes W, Leenhouts K, Rottier PJM, Van Kuppeveld FJM, Haijema BJ, De Haan CAM. Characterization of epitope-specific anti-respiratory syncytial virus (Anti-RSV) antibody responses after natural infection and after vaccination with formalin-inactivated RSV. J Virol. 2016;90:5965–77. doi:10.1128/JVI.00235-16.
- Chang J, Srikiatkhachorn A, Braciale TJ. Visualization and characterization of respiratory syncytial virus F-specific CD8(+) T cells during experimental virus infection. J Immunol. 2001;167:4254–60. doi:10.4049/jimmunol.167.8.4254.
- Varga SM, Wissinger EL, Braciale TJ. The attachment (G) glycoprotein of respiratory syncytial virus contains a single immunodominant epitope that elicits both Th1 and Th2 CD4+ T cell responses. J Immunol. 2000;165:6487–95. doi:10.4049/jimmunol.165.11.6487.
- Hwang HS, Kwon YM, Lee JS, Yoo SE, Lee YN, Ko EJ, Kim M-C, Cho M-K, Lee Y-T, Jung Y-J, et al. Co-immunization with virus-like particle and DNA vaccines induces protection against respiratory syncytial virus infection and bronchiolitis. Antiviral Res. 2014;110:115–23. doi:10.1016/j.antiviral.2014.07.016.
- Hwang HS, Lee YT, Kim KH, Ko EJ, Lee Y, Kwon YM, Kang S-M. Virus-like particle vaccine primes immune responses preventing inactivated-virus vaccine-enhanced disease against respiratory syncytial virus. Virology. 2017;511:142–51. doi:10.1016/j.virol.2017.08.022.
- Monnier J, Zabel BA. Anti-asialo GM1 NK cell depleting antibody does not alter the development of bleomycin induced pulmonary fibrosis. PLoS ONE. 2014;9:e99350. doi:10.1371/journal.pone.0099350.
- Kasai M, Iwamori M, Nagai Y, Okumura K, Tada T. A glycolipid on the surface of mouse natural killer cells. Eur J Immunol. 1980;10:175–80. doi:10.1002/eji.1830100304.
- Crouse J, Xu HC, Lang PA, Oxenius A. NK cells regulating T cell responses: mechanisms and outcome. Trends Immunol. 2015;36:49–58. doi:10.1016/j.it.2014.11.001.
- Pallmer K, Oxenius A. Recognition and regulation of T cells by NK cells. Front Immunol. 2016;7:251. doi:10.3389/fimmu.2016.00251.
- Wong TM, Petrovsky N, Bissel SJ, Wiley CA, Ross TM. Delta inulin-derived adjuvants that elicit Th1 phenotype following vaccination reduces respiratory syncytial virus lung titers without a reduction in lung immunopathology. Hum Vaccin Immunother. 2016;12:2096–105. doi:10.1080/21645515.2016.1162931.
- Lambert SL, Aslam S, Stillman E, MacPhail M, Nelson C, Ro B, Sweetwood R, Lei YM, Woo JC, Tang RS, et al. A novel respiratory syncytial virus (RSV) F subunit vaccine adjuvanted with GLA-SE elicits robust protective TH1-type humoral and cellular immunity in rodent models. PLoS ONE. 2015;10:e0119509. doi:10.1371/journal.pone.0119509.
- Johnson TR, Hong S, Van Kaer L, Koezuka Y, Graham BS. NK T cells contribute to expansion of CD8(+) T cells and amplification of antiviral immune responses to respiratory syncytial virus. J Virol. 2002;76:4294–303. doi:10.1128/JVI.76.9.4294-4303.2002.
- Schneider-Ohrum K, Cayatte C, Bennett AS, Rajani GM, McTamney P, Nacel K, Hostetler L, Cheng L, Ren K, O’Day T, et al. Immunization with low doses of recombinant postfusion or prefusion respiratory syncytial virus F primes for vaccine-enhanced disease in the cotton rat model independently of the presence of a Th1-biasing (GLA-SE) or Th2-biasing (Alum) adjuvant. J Virol. pp.91. 2017. doi:10.1128/JVI.02180-16.
- Clark CM, Guerrero-Plata A. Respiratory syncytial virus vaccine approaches: a current overview. Curr Clin Microbiol Rep. 2017;4:202–07. doi:10.1007/s40588-017-0074-6.
- Walsh EE, Peterson DR, Kalkanoglu AE, Lee FE, Falsey AR. Viral shedding and immune responses to respiratory syncytial virus infection in older adults. J Infect Dis. 2013;207:1424–32. doi:10.1093/infdis/jit038.
- Cullen LM, Blanco JC, Morrison TG. Cotton rat immune responses to virus-like particles containing the pre-fusion form of respiratory syncytial virus fusion protein. J Transl Med. 2015;13:350. doi:10.1186/s12967-015-0705-8.
- Topham NJ, Hewitt EW. Natural killer cell cytotoxicity: how do they pull the trigger? Immunology. 2009;128:7–15. doi:10.1111/j.1365-2567.2009.03123.x.
- Ko E-J, Lee Y, Lee Y-T, Hwang HS, Park Y, Kim K-H, Kang S-M. Natural killer and CD8 T cells contribute to protection by formalin inactivated respiratory syncytial virus vaccination under a CD4-deficient condition. Immune Netw. 2020;20. doi:10.4110/in.2020.20.e51.
- Tessmer MS, Fatima A, Paget C, Trottein F, Brossay L. NKT cell immune responses to viral infection. Expert Opin Ther Targets. 2009;13:153–62. doi:10.1517/14712590802653601.
- Ferlazzo G, Morandi B. Cross-talks between natural killer cells and distinct subsets of dendritic cells. Front Immunol. 2014;5:159. doi:10.3389/fimmu.2014.00159.
- Walzer T, Dalod M, Robbins SH, Zitvogel L, Vivier E. Natural-killer cells and dendritic cells: “l’union fait la force”. Blood. 2005;106:2252–58. doi:10.1182/blood-2005-03-1154.
- Andoniou CE, van Dommelen SL, Voigt V, Andrews DM, Brizard G, Asselin-Paturel C, Delale T, Stacey KJ, Trinchieri G, Degli-Esposti MA, et al. Interaction between conventional dendritic cells and natural killer cells is integral to the activation of effective antiviral immunity. Nat Immunol. 2005;6:1011–19. doi:10.1038/ni1244.
- Vitale M, Della Chiesa M, Carlomagno S, Pende D, Arico M, Moretta L, Moretta A. NK-dependent DC maturation is mediated by TNFalpha and IFNgamma released upon engagement of the NKp30 triggering receptor. Blood. 2005;106:566–71. doi:10.1182/blood-2004-10-4035.