ABSTRACT
In addition to the classical immunological functions such as neutralization, antibody-dependent cellular cytotoxicity, and complement activation, IgG antibodies possess a little-recognized and under-utilized effector function at mucosal surfaces: trapping pathogens in mucus. IgG can potently immobilize pathogens that otherwise readily diffuse or actively swim through mucus by forming multiple low-affinity bonds between the array of pathogen-bound antibodies and the mucin mesh. Trapping in mucus can exclude pathogens from contacting target cells, and facilitate their rapid elimination by natural mucus clearance mechanisms. Despite the fact that most infections are transmitted at mucosal surfaces, this muco-trapping effector function has only been revealed within the past decade, with the evidence to date suggesting that it is a universal effector function of IgG-Fc capable of immobilizing both viral and highly motile bacterial pathogens in all major mucosal secretions. This review provides an overview of the current evidence for Fc-mucin crosslinking as an effector function for antibodies in mucus, the mechanism by which the accumulation of weak Fc-mucin bonds by IgG bound to the surface of a pathogen can result in immobilization of antibody-pathogen complexes, and how trapping in mucus can contribute to protection against foreign pathogens.
KEYWORDS:
Introduction
Antibodies (Abs) are defense proteins produced by the host immune system that specifically binds bacteria, viruses and other entities to protect against infections and toxicities via an intricate array of immunological functions.Citation1–3 The Fab arms of an Ab molecule can bind to critical epitopes on a pathogen with high specificity, directly inhibiting the pathogen from binding and infecting target cells in a process known as neutralization.Citation4–6 The Fc domain on pathogen-bound Ab also enables other immunological effector functions, including triggering the phagocytosis of pathogens (opsonization), activation of natural killer cells to ingest infected cells via antibody dependent cellular cytotoxicity (ADCC), as well as initiation of the classical complement pathway, a cascade of enzymes that lyses pathogen membranes.Citation7–9
While these effector functions have been well characterized, Abs including IgG also perform a major but little-recognized effector function in mucus gels secreted at mucosal surfaces: Abs form transient Fc-mucin bonds that trap antibody-coated pathogens in mucus. This effector function provides a powerful means by which the immune system can fortify the barrier properties of mucus to exclude pathogens from contacting their target cells to establish initial infections. It also provides a mean of blocking the local spread of infections, and physically eliminating progeny viruses quickly from the mucosa via natural mucus clearance mechanism(s).
Evidence of Ab-mucin interactions was first observed over 40 years ago: Kremer and Jager observed that anti-sperm Abs could trap individual vigorously motile sperm in cervical mucus, resulting in sperm shaking in place for hours until they die;Citation10–12 Kremer and Jager referred to this as the “shaking phenomenon”. Their observations contrast with the ability for multivalent secreted antibodies such as sIgA and IgM to agglutinate pathogens that arrive at mucosal surfaces in high concentrations into clusters too large to permeate mucus. As will be discussed below, Ab-mediated trapping of individual pathogens can occur before pathogens become agglutinated, and more importantly, can be mediated by IgG in mucus. Unfortunately, in the subsequent decades, owing in part to the difficulty in performing studies directly with fresh mucus gels, very few investigators have investigated this Ab-mucin crosslinking mechanism as a potential effector function for Abs in mucus, and particularly overlooked the potential role for IgG-mucin interactions. As a result, while there have been thousands of clinical trials using IgGs for systemic applications, there have been very few attempts to harness IgGs for mucosal therapy and protection in clinical settings.
In this review, we will overview the evidence to date that supports Fc-mucin crosslinking as a major effector function for Abs in mucus, the principle of multiple transient and weak Fc-mucin bonds that avidly trap Ab-coated pathogens in mucus, and how trapping in mucus can contribute to and potentially synergistically enhance the overall immunological defense at mucosal surfaces. The results to date underscore Fc-mucin crosslinking as a universal effector function in all major mucus secretions.
Biophysical properties of mucus, and the need for adaptive immune response to reinforce the mucus barrier
Mucus refers to the viscoelastic secretions that coat exposed epithelial surfaces such as the respiratory, gastrointestinal and reproductive tracts. On a macroscopic level, mucus can serve as a lubricant that facilitates transport of food and movement (blinking, copulation, etc.) without damaging the epithelium. The macroscopic viscoelasticity of mucus is highly regulated in order to perform these functions.Citation13 On a microscopic level, mucus is a hydrogel composed of heavily glycosylated mucin fibers that are crosslinked and entangled to form a porous network.Citation14–16 The mesh structure can impede diffusion either by steric obstruction via the pore size of its mucin network, or by direct adhesion to the mucin fibers itself (viscidity). The characteristic mesh spacings in mucus vary depending on the mucosal surface. For example, gastrointestinal mucus has a relatively large pore structure as it allows the diffusional transport of beads up to 2 µm in size.Citation17 In comparison, airway mucus (AM) only permits rapid diffusion of particles up to 0.2 µm16, while cervicovaginal mucus (CVM), with an estimated average pore size of ~0.34 µm,Citation14 allows rapid diffusion of beads as large as 0.5 µm.
To reach and infect target cells in mucosal tissues, viruses and intracellular obligate bacteria must first permeate through mucus gel overlaying the epithelial layer. Not surprisingly, most pathogens can readily permeate through mucus, including viruses (e.g., HIV,Citation18,Citation19 HSV,Citation20,Citation21 HPV,Citation18 Norwalk,Citation18 etc.) and bacteria possessing active motility (e.g., H. pylori,Citation22 Salmonella,Citation23,Citation24 etc.). Importantly, pathogens have evolved surfaces that do not adhere to mucin fibers, typically by having minimal exposed hydrophobic surfaces and with hydrophilic surfaces that are anionic, or are highly charged but neutral. Most mammalian viruses are also significantly smaller than pore size of mucus, and thus can permeate mucus by Brownian diffusion alone with little steric hindrance.
The hydrogel nature of mucus implies it can impede the permeation of pathogens either by steric obstruction by the mucins mesh, or by adhesive interactions with the mucins mesh. With any method that seeks to limit pathogen permeation across mucus, it is essential to only impede transport of specific pathogens, rather than limit transport nonspecifically that may in turn impede the absorption of nutrients, cells and immune molecules. Broad changes to the mucus mesh structure would also likely adversely impact the macroscopic viscoelasticity of the mucus gel that in turn could compromise other essential functions. As a result, to reinforce the barrier properties of mucus and limit pathogens from contacting target cells without altering the other functions of the mucus gel, the only approach is to harness third party crosslinker molecules that could transform the barrier properties of mucus against specific pathogens. As the key molecules in the adaptive immune system that can recognize foreign pathogens with high specificity, Ab represent the ideal platform for reinforcing the mucosal defense against specific pathogens.
Abundant quantities of Abs are present in mucus, including IgG
Abs are produced and secreted in abundant quantities by populations of plasmablasts and plasma cells in mucosal epithelial, a subset of B-lymphocytes that is often collectively referred to as Ab-secreting cells (ASC). Each ASC is specialized to produce large quantities of a single isotype with specific Fab moieties, and the Abs are produced in close vicinity with cells that transport them into mucus gel. In systemic circulation, Abs are secreted by plasma cells in blood and lymph. Only a small fraction of Ab in mucus is derived from the systemic circulation; instead, the majority of Abs in mucus is produced and secreted by local ASCs within mucosal epithelium. As a result, the clonal repertoire of mucosal Ab, as well as their concentrations, are distinct not only from those in the systemic circulation, but also across different mucosal surfaces.
The conventional view in immunology is that secretory IgA is the predominant isotype in mucus and thus the primary Ab responsible for mucosal immune defense. This longstanding dogma is owed in part to the fact that IgG is the dominant immunoglobulin in the blood, the limited quantities of IgA in the blood, and the abundant quantities of sIgA in GI mucus. Nevertheless, large quantities of IgG are actually present in mucus lining the lung airways and the female reproductive tract (), including at least 10-fold more IgG is present in CVM than sIgA.Citation21,Citation32–34
Table 1. Measurements of immunoglobulin concentration in lavages of various mucosal secretions. Though IgA comprises the majority of antibody at most surfaces, substantial concentrations of IgG are found at many mucosal interfaces, and predominates in cervicovaginal secretions. The concentrations have been rounded to two significant figures
IgM and IgA, particularly sIgA, are difficult to manufacture and purify at scale, and are notoriously unstable and prone to aggregation. In contrast, IgG is the easiest Ab isotype to manufacture and store, reflected by the fact that virally all monoclonal Ab on the market or in clinical development are IgG serotypes.Citation36 Given that there are abundant quantities of IgG in mucus and the ease in producing IgG, we will focus the bulk of the review on IgG effector functions in mucus.
Ab-mucin interactions are low affinity
Given the abundance of endogenous Ab in different mucus secretions, it was logical to assume that evolution would have resulted in Ab working in tandem with mucus or mucus constituents to enhance protection. Gel-forming mucins are the most abundant constituent in mucus,Citation37,Citation38 and thus represent the most obvious target for Ab to bind within the mucus gel. This led early investigations of muco-trapping Ab functions to probe for interactions between Ab and mucins, with the expectation of detecting high-affinity Ab-mucin bonds based on the seemingly intuitive assumption that high-affinity Ab-mucin bonds would facilitate the most potent trapping of pathogens in mucus. Indeed, much of the early observations, including those by Kremer and Jager, assumed that Ab were tightly bound to mucins.Citation39–41
However, high-affinity interactions between endogenous Ab and mucins were never found. Instead, much of the work that probed for Ab-mucin interactions found that Ab form only low-affinity and transient bonds with mucins.Citation18,Citation42,Citation43 The affinities between individual Ab and mucins are so weak that they cannot be readily revealed by most conventional methods for characterizing Ab-antigen bonds, such as surface plasmon resonance or biolayer interferometry. The detection of very weak affinity between Ab and mucins was only done using microscopy techniques such as Fluorescence Recovery After Photobleaching (FRAP), which measures the rate of diffusion of fluorescently tagged molecules in mucus gel vs. water. Early work, initially measured by Saltzman et al. and later confirmed and extended by Olmsted et al. found that IgG and sIgA diffuse rapidly in human cervical mucus, slowed only marginally compared to their diffusion in salineCitation18,Citation42 (). As Ab are considerably smaller than the pores in native mucus gels,Citation14,Citation18 this small reduction in diffusivity is not likely the result of steric hindrance, since much larger viruses and virus-sized particles can diffuse through mucus unhindered. Instead, it is likely the result of short-lived bonds between Ab and mucins, weak enough to be readily broken by thermal energy alone.
Table 2. Measurements of normalized diffusion coefficients for Abs in cervical mucus. If a particle diffuses in mucus as fast as it diffuses in buffer, Dmuc/Dpbs = 1. Abs with multiple entries are results of independent experiments. IgM, sIgA aggregates, and IgM Fc complex with removed Fab domains are slowed moderately in mucus. IgA and IgG were hardly slowed in mucus
Such weak affinity between individual Ab and mucins indicates that a single Ab alone could not directly crosslink a pathogen to mucins and immobilize it in mucus. This has led most researchers in the intervening years to assume that Ab cannot work in tandem with mucins to trap pathogens in mucus, and thus completely overlook the potential potency of muco-trapping effector function.
Arrays of Ab bound to the surface of a pathogen enable potent trapping by forming multivalent low-affinity Ab-mucin bonds
Since individual Ab-mucin bonds are very weak and transient, the key to investigating potential muco-trapping effector functions is to study how Abs in mucus can specifically impede the mobility of pathogens in mucus, rather than to probe for the affinity between individual Abs to mucins. depicts how multiple Ab bound to the surface of a pathogen creates an array of Ab that form multiple weak-affinity bonds to mucins, such that at any given time at least one Ab-mucin bond is present. This ensures that an individual virus or bacteria, even vigorously motile bacteria or sperm, can be trapped with near permanent avidity.Citation44 Even though thermal energy rapidly breaks these low affinity bonds, the vigorous motility of a human spermatozoa is not strong enough to break a single such bond.Citation45
Figure 1. Schematic of Ab-mediated trapping. Virus alone can freely diffuse through the mucin fibers. Weak interactions between the Fc portion of the Ab and the mucin allow individual Ab to diffuse freely. As the Fab arms bind to antigen and Ab accumulates on the viral surface, Ab-virus complexes become tightly complexed to the matrix.
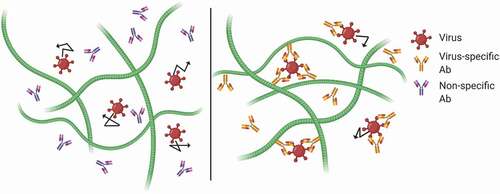
As noted above, the first evidence of muco-trapping functions of Ab was the shaking phenomenon as observed by Kremer and Jager for Ab-mediated trapping of sperm in mid-cycle endocervical mucus. Unfortunately, decades lapsed before Phalipon et al. found the SC component of sIgA may associate bacteria to mucins.Citation40 Another decade followed before my coworkers and I demonstrated that an IgG against a virus, Herpes Simplex Virus (HSV), can trap HSV in mucus and block vaginal Herpes transmission in mice due to its muco-trapping effector functions.Citation21
Sperm are much larger than viruses and bacteria; thus, it was unclear based on observations of sperm shaking in mucus whether there can be a sufficient number of Ab bound to individual viruses or bacteria to exert sufficient avidity to mucins to trap them in mucus. Because the motion of viruses is driven by Brownian forces, i.e. momentum transfer due to thermal excitation of surrounding water molecules, the number of bound Ab to trap viruses is almost certainly considerably less than the number needed to immobilize either sperm or motile bacteria, both of which possess vigorous motility due to active motility apparatuses. This is offset by the fact that viruses are much smaller than bacteria, which limits the total number of Ab that can accumulate on the virus surface. Furthermore, some viruses have a relative paucity of viral epitopes available for Ab to bind: for instance, there is an average of only 14 ± 7 Env glycoproteins on individual HIV virions,Citation46 in contrast to the 400–500 spike proteins present on the surface of similarly sized influenza virus.Citation47 These constraints can severely limit the number of Ab that accumulate on the virus surface. While motile bacteria are much larger than viruses, a larger number of bound Ab is needed to exert sufficient avidity to overcome their vigorous motility.
To investigate whether IgG could trap viruses in mucus, we used high-resolution multiple particle tracking to quantify the motion of fluorescent HSV-1 viruses in fresh and minimally diluted CVM specimens with different amounts of endogenous HSV-binding IgG.Citation21 We found that HSV-1 exhibited rapid diffusion in CVM with low to no endogenous anti-HSV IgG, but was effectively trapped in CVM with appreciable levels of anti-HSV-1 IgG. To confirm trapping was mediated by anti-HSV-1 IgG, we added different amounts of anti-HSV IgG into CVM specimens with low titers of anti-HSV-1 IgG, and saw a clear dose-dependent immobilization of HSV-1, with effective trapping occurring at sub-neutralizing levels of anti-HSV-1 IgG.Citation21 We found that even a non-neutralizing anti-HSV mAb conferred sterilizing protection against vaginal Herpes transmission in mice, and the protection was eliminated when vaginal mucus was removed by gentle lavage prior to viral challenge, indicating that the IgG was working in tandem with mucus to block transmission.Citation21 This work represents the first published evidence we are aware of that directly demonstrates IgG, which has no secretory component, can potently trap viruses in mucus, leading to protection against mucosal transmission.
In the years since, we have published a series of investigations that collectively suggests muco-trapping is a universal effector function for IgG in all major mucosal secretions. In the female reproductive tract, we showed that a monoclonal anti-gD IgG can effectively trap HSV-1 in CVM collected at different times across the menstrual cycle, as well as in CVM from women with different vaginal microbiota.Citation20 More recently, we also found that antigen-specific IgGs can trap HIV-1 in CVM (data not shown), suggesting that even a very limited number of virion-bound IgG may be adequate to enable trapping in mucus. In the respiratory tract, we showed that the immobilization of influenza in fresh human AM is attributed to endogenous influenza-binding IgG and IgA, rather than the longstanding belief that the virus is trapped in AM due to direct binding to sialic acids of mucins via its hemagglutinin.Citation48 We also showed that mAb against Ebola virus-like particles (VLP) potently trapped Ebola VLP in human AM, leading to rapid clearance of most Ebola VLPs form the airways within 30 mins in a mouse airway clearance model.Citation49 Finally, we found that LPS-binding IgG is able to impede the motion of Salmonella bacteria in mouse gastrointestinal mucus without inhibiting flagellar motility (they shake in place like Ab-trapped sperm in cervical vaginal mucus).Citation23 This underscores the ability for IgG to contribute to protection at mucosal tissues where IgG is not usually associated with mucosal immunity, and that the array of bacteria-bound IgG can exert adequate avidity to mucins to enable potent trapping of even vigorously motile bacteria in mucus gel.
IgG-mucin crosslinks is Fc- and N-glycan dependent
While the precise biochemistry supporting Fc effector functions are well understood, little was known about how IgG can form transient bonds with mucins. By selectively modifying anti-HSV-1 IgG that otherwise enabled potent trapping of HSV-1 in CVM, we discovered that the muco-trapping function of IgG is critically dependent on IgG-Fc, and more specifically the N-glycans on the Fc domain (),Citation21 since F(ab’)2 and deglycosylated IgG both failed to trap HSV-1. The interactions are likely attributed to specific sugars on IgG-FcCitation50,Citation51 forming weak and transient hydrogen bonds with sugars on mucins.Citation52
Figure 2. Deglycosylation of IgG abolishes trapping of HSV in CVM. (a) Fc removal from anti-HSV1, confirmed by SDS-PAGE. (b) Deglycosylation of anti-HSV1, confirmed by lectin-ELISA. (c) Effective diffusivity of HSV1 in the CVM treated with intact, Fc-removed, and deglycosylated IgG. Reproduced with permission from.Citation21 Copyright 2014 Springer nature.
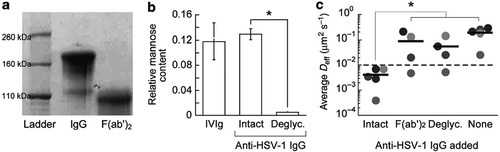
Modest Ab-mucin affinity key to maximal muco-trapping potency
As discussed above, it was long assumed that high-affinity bonds between IgG and mucins would maximize the ability for IgG to facilitate trapping in mucus, due to the expectation that a single strong bond between mucin-bound IgG and the virus would effectively crosslink the virus to mucins and lead to trapping. To test this hypothesis, we created high-affinity bonds between IgG and a biological mucin-like matrix (Matrigel®), based on using neutravidin to crosslink biotinylated anti-PEG IgG to biotinylated Matrigel®. PEG-coated beads introduced into this IgG-anchored matrix diffused largely unhindered, similar to Matrigel® with no anti-PEG IgG added.Citation44 In contrast, when we add unmodified anti-PEG IgG to the same biotinylated Matrigel®, we found that PEG-coated nanoparticles were effectively immobilized within minutes, similar to anti-PEG IgG immobilizing PEG-coated nanoparticles in native Matrigel®Citation44 (). It was not until many hours later that Matrigel® with matrix-bound IgG slowly began to immobilize an appreciable fraction of PEG-coated nanoparticles. These results suggest that low-affinity IgG-mucin bonds are actually far more effective than high-affinity IgG-mucin bonds at immobilizing nanoparticulates in mucus.
Figure 3. Transient vs. stable Ab-matrix bonds. Diffusion of 200 nm polyethylene glycol (PEG)-conjugated latex nanoparticles in biotinylated Matrigel® modified with neutravidin. (a) Representative traces of nanoparticles in Matrigel® with no added IgG (control), anti-PEG IgG (IgG), or biotinylated anti-PEG IgG (IgG-biotin) exhibiting effective diffusivities within one SEM of the ensemble average at a timescale of 1 s. (b) Distributions of the mean logarithms of individual particle effective diffusivities (Deff) at a timescale of 0.2667 s. Log (Deff) values to the left of the dashed line correspond to particles with displacements of less than 100 nm (i.e., roughly the particle diameter) within 0.2667 s. (c) Ensemble-averaged geometric mean square displacements (<MSD>) as a function of timescale, (d) mean Deff of all particles in each condition, and (e) fraction of mobile nanoparticles in Matrigel® treated with different IgG. Reproduced under creative commons license from.Citation44
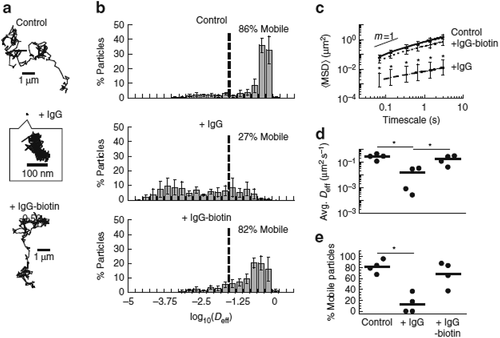
To understand this seemingly counterintuitive finding, we must fully consider the interplay between the kinetics of Ab diffusion and binding to pathogen, as well as pathogen concentration and diffusion/mobility. It is important to note that most virions can diffuse quite rapidly through a mucus layer, leaving a very limited window of opportunity for Ab to bind the virion prior to the virion diffusing through mucus and reaching the underlying epithelium.Citation53 Thus, Ab must accumulate on an impinging virus and reach the threshold of Ab-mucin bonds needed to crosslink the virus to mucins before the virus transits through the mucus layer. When an Ab binds strongly to mucins, the Ab becomes effectively immobilized, which leads to greatly reduced rates of encountering the virus and correspondingly very slow rate of mucin-anchored Ab actually capturing the virus. Even just one bond takes many hours to form. In contrast, when Ab only associates transiently to mucins, it is still able to rapidly diffuse through mucus and quickly accumulate on the virus surface. In turn, this array of Abs can crosslink the virus to mucins with near-permanent avidity as soon as a sufficient number of bound IgG is achieved. These results agree well with the Smoluchowski diffusional encounter rate, which is proportional to the sum of the diffusivity of both species.Citation54 The likelihood of collision is thus much higher when both Ab and virion are diffusive (owing to the much higher diffusivities of the Ab vs. pathogen), than when the Ab is immobilized to mucins (where only the much larger and thus much slower virus is diffusive).
These results and the corresponding mechanism are fully confirmed by mathematical modeling. Chen et al. found that even exceedingly transient IgG-mucin bonds (where IgG diffusivity is slowed only by ~10-15% in mucus compared to in water) is theoretically sufficient to trap particles, leading to the formation of a front of immobilized particles farthest from the epithelial cells.Citation55 Subsequent computational work investigating a range of theoretical Fc-mucin interaction strengths showed that once Ab exceed a certain affinity to mucins, the Abs that bind more and more tightly to mucins are less and less able to trap diffusive species.Citation56 Conversely, if Ab-mucin interactions are too weak, too many Ab must be accumulated on the surface of a pathogen in order to mediate trapping, again resulting in suboptimal potency. The computational model suggested that trapping was most effective when the affinity of Ab-mucin interactions reduced Ab diffusivity in mucus to 25%-50% of its diffusivity in water. This mucin-affinity is most consistent with the measured diffusivity of IgM in mucus.Citation18,Citation42 This theoretical prediction was confirmed by experiments using mouse mucus and other biogels that showed IgM exhibited considerably greater muco-trapping potencies than IgG.Citation52,Citation57,Citation58
A key attribute to weak Ab-mucin bonds is the ability for the same mucus gel to enable trapping of a diverse array of pathogens simultaneously. In the same study above, we found that addition of excessive quantities of a second IgG does not impact the trapping potency of the first IgG. Experiments using fresh mucus ex vivo showed potent trapping occurs with IgG (i.e. Ab that binds weakly to mucins) at concentrations well below 1–3 ug/ml in mucus.Citation21,Citation57 If IgG anchors tightly to mucins, computational models predict comparable trapping potency (i.e. trapping a comparable fraction of viruses) would necessitate the presence of antigen-specific IgG in excess of 250 ug/ml,Citation44 nearly a quarter of the total IgG typically found in CVM. In other words, weak IgG-mucin bonds directly reduce the amount of IgG needed to facilitate potent trapping in mucus. Furthermore, since the total concentration of mucin fibers in a mucus gel is confined to a few percent by weight, the number of available sites that IgG can bind to is by extension also finite. Should specific IgG actually bind tightly to mucins (i.e. low unbinding rates), this would greatly limit the potential number of distinct nanospecies which could be crosslinked to the mesh and trapped in mucus at any particular moment in time. In contrast, with low IgG-mucin affinity, binding sites on mucins will only be occupied for sustained durations (i.e., very slow unbinding) with IgG/pathogen complexes with polyvalent interactions. Altogether, weak Ab-mucin affinity is essential to enable a mucus gel to trap as many diverse species as possible, while also requiring lower concentration of Ab against any individual pathogen species.
A framework for muco-trapping effector functions of Ab based on Ab-mucin interactions
The experimental observations in different mucus secretions as well as other biological matrices, coupled with theoretical modeling, point to a generalized system that harnesses weakly adhesive Ab as crosslinkers that can potently and rapidly trap diverse pathogen species within mucus or any similar matrix gel. The optimal system must meet the following conditions:Citation44
The Ab-mucin binding and unbinding kinetics must be distinctly faster than the binding kinetics between the Ab and the pathogen. Practically, this implies Ab must undergo rapid diffusion within mucus and rapidly accumulate on the pathogen surface.
The pathogen must possess a sufficient number of surface epitopes such that an array of pathogen-bound Ab can form sufficient number of crosslinks between the pathogen and the mucin gel to overcome the forces driving the pathogen motion. In virtually all cases, this must be greater than one target epitope per pathogen. A particle with only one potential epitope for Ab binding would only be able to maximize trapping when binding affinity between the Ab and mucin is very high, and could not rely on multiple crosslinks.
Ab must be smaller than the pathogen. This means Ab diffusivity is higher than the diffusivity of the pathogen under native gel conditions. It also means that multiple Ab can bind the pathogen without steric hindrance limiting their ability to accumulate on the pathogen surface. The overall encounter rate between Abs and pathogen is dictated by the Smoluchowski encounter rate.Citation54 When the diffusivity of the Ab is high, the encounter rate between the pathogen to be captured and Abs will be maximized. Although higher pathogen diffusivity can also increase the encounter rate, this also reduces the amount of time available for Ab to accumulate on the surface of the pathogen before the pathogen transits through the mucus gel. Thus, a smaller and more diffusive Ab is more effective for trapping.
Ab-pathogen binding must be fast enough that a sufficient number of Abs will bind and accumulate on the pathogen before the pathogen permeates through mucus. In this regime, the kon of the binding kinetics dominates over both the koff and overall binding affinity.Citation53
Ab-mucin interactions must be sufficiently short-lived relative to their concentration in mucus such that individual Ab will not saturate binding sites on mucins and out compete cross-linked complexes.
Other immunoglobulins in mucus
As discussed above, IgM possesses more suitable mucin-affinity for greater muco-trapping potency than IgG. Monomeric IgA, due to its similar mucin-affinity as IgG, likely can facilitate muco-trapping with comparable potency as IgG. As mentioned above, Phalipon et al. suggested that secretory component on sIgA could anchor (trap) pathogenic bacteria to the mucus gel in mouse nasal secretions, thereby excluding them from contact and entry into target cells.Citation40
An additional protective mechanism often associated with mucosal surfaces is immune exclusion via agglutination, which describes the agglutination of microorganisms into clusters too large to diffuse through the pores in mucus.Citation59 Protection by polymeric immunoglobulins, such as secretory IgA (sIgA) that is abundant in select mucus secretions,Citation60 is often attributed to their ability to induce aggregate formation,Citation61 particularly against large and actively motile organisms such as bacteria and sperm.Citation11,Citation59 sIgA is also well suited for mucosal protection due to protease resistance imparted by the secretory component.Citation62 The limited capacity of sIgA to activate complement or phagocytic uptake further limits inflammatory damage to the epithelium.Citation62,Citation63 These results have led many to attempt to elicit sIgA at mucosal surfaces to combat disease transmission, including vaccines targeting sIgA response,Citation64,Citation65 IgA immunoprophylaxis using adeno-associated viral vectors,Citation66 and hematopoietic stem/progenitor cells pre-transduced with an IgA gene.Citation67
Despite its advantages, sIgA is not the dominant Ab type at all mucosal surfaces. Efforts to elicit sIgA responses in vaccine and anti-viral prophylaxis development are predicated in part on the notion that the agglutinating activity of sIgA would enhance the efficacy of the Ab effector response. However, computational modeling suggests there are severe limits to harnessing agglutination to preventing viral transmission.Citation68 To agglutinate two virions together, the two virions must first collide with each other. However, at physiological viral loads, the vast majority of virions could be expected to pass through the mucosal layer without having ever made a collision with another virion ().Citation68 This indicates that agglutination is unlikely to play a dominant role in protection for species undergoing only passive diffusion, and becomes significant only when pathogen concentrations are exceptionally high, or possess active motility together with high concentration to confer a higher collision rate. In the GI tract, since few viruses can survive the acidic and highly degradative gastric environments, high endogenous levels of IgG may be unnecessary. The high levels of sIgA likely reflect the immunological mechanism through which beneficial commensal bacteria can be retained in the small and large intestines without overgrowth and without the commensal bacteria penetrating across the unstirred adherent mucus layer, consistent with the histological findings that the commensal bacteria are generally found only in the outermost luminal mucus layer.
Figure 4. Simulations of virion collisions at different viral loads and particle sizes. The fraction of virions over the first 12 hours post ejaculation as a function of virion density in semen which have undergone no collision (singlets), one collision (doublets) or two or more collisions (triplets+) for all HIV virions (a) in semen/CVM mixture and (b) that have reached the vaginal epithelium. (c–d) The fraction pf (c) 50 nm pathogens and (d) 300 nm pathogens in diameter that have diffused across CVM and reached the vaginal epithelium. Solid line represents an exponential function approximation of the virion load arriving at the epithelial layer that experiences no collisions with other virions. Modified and reproduced under creative commons license from.Citation68
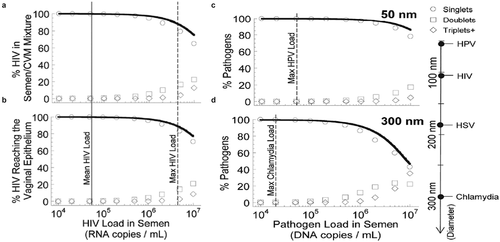
How trapping pathogens in mucus protects against infections
First, trapping in mucus directly reduces the flux of virions that can reach target cells, whether it reflects new viruses deposited at a mucosal surface as part of initial transmission, or the local spread of progeny viruses shed from infected cells. In turn, a large fraction of trapped virions will be rapidly eliminated by natural mucus clearance mechanisms, such as cough and mucociliary clearance in the airways, or as part of post-coital discharge from the female reproductive tract that usually occurs within minutes. By reducing the presence of antigens at the local mucosa via mucus clearance, muco-trapping may reduce potential inflammation arising from classical IgG effector functions. Furthermore, by impeding pathogen motion and increasing the time it takes for pathogens to permeate through mucus and reach target cells, even partial muco-trapping (i.e. slowing) may also lead to more complete inactivation of pathogens via other innate protective mechanisms, such as by defensinsCitation69 or via spontaneous thermal degradation.Citation70
Trapping virions in mucus is likely an especially effective means of preventing transmission when the transmission rate is relatively low. For instance, with heterosexual HSV-2 and HPV transmission, the rates of transmission are actually quite low, with women acquiring HSV-2 at a rate of ~8.9 per 10,000 sex acts,Citation71 and HPV at a rate of ~15% per year over the initial 3 years from first intercourse.Citation72 Such low rates of transmission imply that very few, if any, viruses actually reach and infect the epithelium per exposure even in the absence of a pathogen-specific mAb. Thus, mAb-mediated trapping will further decrease the fraction of virions that reach target cells, resulting in a proportional reduction in transmission of infection.
Conclusion
The muco-trapping functions of Ab-Fc, including IgG-Fc offers a powerful mechanism by which the immune system can reinforce the mucus barrier and block infections at all mucosal surfaces. By harnessing the somatic hypermutations processes that generate Ab with exceptional specificity and affinity, our body is able to transform mucus gel with relatively static mucin biochemistry into a mucus gel layer that can readily adapt to an ever-evolving range of pathogens. The consistency of this muco-trapping function across all the major mucosal secretions, as well as the potency against a wide range of viral and bacterial pathogens, highlight not only its physiological importance but also suggests it is likely an evolutionary feature of our overall immune system.
To date, this muco-trapping function of IgG-Fc remains little recognized and rarely used in therapeutic development. In addition to preventing initial infections and blocking the local spread of early infections, harnessing Fc-mucin interactions also opens up the possibility of targeting non-neutralizing but otherwise well conserved epitopes for monoclonal antibody (mAb) or vaccine development. Motivated in part by the biophysical and biochemical insights into the muco-trapping functions of IgG-Fc, we are beginning to see biomedical applications utilizing topically delivered muco-trapping mAbs, including vaginal contraception and inhaled therapy against diseases from respiratory infections such as COVID-19.
Finally, there are numerous opportunities that are untapped. Muco-trapping may be part of a greater matrix-trapping functions of Ab, as evident by our work investigating IgG- and IgM- mediated trapping in Matrigel®, laminin, collagen, alginate and agarose.Citation44,Citation52,Citation58 Continued advances in the chemistries involved with Fc-mucin interactions will likely lead to improved next generation Ab that can enable much more potent trapping in mucus, similar to efforts over the past two decades that have led to IgG-Fcs enabling prolonged circulation and tuning specific Fc effector functions.
Disclosure of potential conflicts of interest
S.K.L is founder of Mucommune, LLC and currently serves as its interim CEO. S.K.L is also founder of Inhalon Biopharma, Inc, and currently serves as its CSO, Board of Director, and Scientific Advisory Board. S.K.L has equity interests in both Mucommune and Inhalon Biopharma; S.K.L’s relationships with Mucommune and Inhalon are subject to certain restrictions under University policy. The terms of these arrangements are managed by UNC-CH in accordance with its conflict of interest policies. A.S and S.K.L are inventors on patents licensed by Mucommune and Inhalon Biopharma.
Acknowledgments
The senior author (Lai) is deeply grateful to Richard Cone for introducing him to the world of mucosal antibody functions. His encouragement and mentorship in the intervening years played an essential role in establishing many of the key aspects that have been established to date. The proverbial “standing on the shoulders of giants” is never more true than in this context. Although there are too many to name, Lai would also like to acknowledge the entire team of colleagues and collaborators that Lai had the privilege to work with on this topic over the past 10 years.
Additional information
Funding
References
- Casadevall A, Pirofski LA. A new synthesis for antibody-mediated immunity. Nat Immunol. 2012;13:1–10. doi:https://doi.org/10.1038/ni.2184.
- Turula H, Wobus CE. The role of the polymeric immunoglobulin receptor and secretory immunoglobulins during mucosal infection and immunity. Viruses. 2018;10(5):237. doi:https://doi.org/10.3390/v10050237.
- Kozlowski P, Neutra M. The role of mucosal immunity in prevention of HIV transmission. Curr Mol Med 2005;3:217–28. doi:https://doi.org/10.2174/1566524033479852.
- Burton DR, Mascola JR. Antibody responses to envelope glycoproteins in HIV-1 infection. Nat Immunol. 2015;16:571–76. doi:https://doi.org/10.1038/ni.3158.
- Dashti A, DeVico AL, Lewis GK, Sajadi MM. Broadly neutralizing antibodies against HIV: back to blood. Trends Mol Med. 2019;25:228–40. doi:https://doi.org/10.1016/j.molmed.2019.01.007.
- Klasse PJ. Neutralization of virus infectivity by antibodies: old problems in new perspectives. Adv Biol 2014;2014:1–24. doi:https://doi.org/10.1155/2014/157895.
- Dunkelberger JR, Song WC. Complement and its role in innate and adaptive immune responses. Cell Res. 2010;20:34–50. doi:https://doi.org/10.1038/cr.2009.139.
- Huber M, Olson WC, Trkola A. Antibodies for HIV treatment and prevention: window of opportunity? Curr Top Microbiol Immunol. 2008;317:39–66. doi:https://doi.org/10.1007/978-3-540-72146-8_2.
- Kilian, M., & Russell, M. W. (1994). Function of Mucosal Immunoglobulins. In J. B. Pearay L. Ogra, Jiri Mestecky, Michael E. Lamm, Warren Strober, Jerry R. McGhee (Ed.), Handbook of Mucosal Immunology (pp. 127–137). https://doi.org/https://doi.org/10.1016/b978-0-12-524730-6.50016–6
- Kremer J, Jager S. The sperm cervical mucus contact test: a preliminary report. Fertil Steril 1976;27:335–40. doi:https://doi.org/10.1016/S0015-0282(16)41726-7.
- Jager S, Kremer J, Kuiken J, Slochteren-Draaisma TV, Mulder I, de Wilde-Janssen IW. Induction of the shaking phenomenon by pretreatment of spermatozoa with sera containing antispermatozoal antibodies. Fertil Steril. 1981;36:784–91. doi:https://doi.org/10.1016/S0015-0282(16)45926-1.
- Jager S, Kremer J, Kuiken J, Mulder I. The significance of the Fc part of antispermatozoal antibodies for the shaking phenomenon in the sperm-cervical mucus contact test. Fertil Steril 1981;36:792–97. doi:https://doi.org/10.1016/S0015-0282(16)45927-3.
- Lai SK, Wang YY, Wirtz D, Hanes J. Micro- and macrorheology of mucus. Adv Drug Delivery Rev. 2009;61:86–100. doi:https://doi.org/10.1016/j.addr.2008.09.012.
- Lai SK, Wang YY, Hida K, Cone R, Hanes J. Nanoparticles reveal that human cervicovaginal mucus is riddled with pores larger than viruses. Proc Natl Acad Sci USA 2010;107:598–603. doi:https://doi.org/10.1073/pnas.0911748107.
- Suk JS, Lai SK, Wang -Y-Y, Ensign LM, Zeitlin PL, Boyle MP, Hanes J. The penetration of fresh undiluted sputum expectorated by cystic fibrosis patients by non-adhesive polymer nanoparticles. Biomaterials. 2009;30:2591–97. doi:https://doi.org/10.1016/j.biomaterials.2008.12.076.
- Schuster BS, Suk JS, Woodworth GF, Hanes J. Nanoparticle diffusion in respiratory mucus from humans without lung disease. Biomaterials. 2013;34:3439–46. doi:https://doi.org/10.1016/j.biomaterials.2013.01.064.
- Ermund A, Gustafsson JK, Hansson GC, Keita ÅV. Mucus properties and goblet cell quantification in mouse, rat and human ileal Peyer’s patches. PLoS One. 2013;8. doi:https://doi.org/10.1371/journal.pone.0083688.
- Olmsted SS, Padgett JL, Yudin AI, Whaley KJ, Moench TR, Cone RA. Diffusion of macromolecules and virus-like particles in human cervical mucus. Biophys J. 2001;81:1930–37. doi:https://doi.org/10.1016/S0006-3495(01)75844-4.
- Lai SK, Hida K, Shukair S, Wang -Y-Y, Figueiredo A, Cone R, Hope TJ, Hanes J. Human immunodeficiency virus type 1 is trapped by acidic but not by neutralized human cervicovaginal mucus. J Virol. 2009;83:11196–200. doi:https://doi.org/10.1128/JVI.01899-08.
- Schroeder HA, Nunn KL, Schaefer A, Henry CE, Lam F, Pauly MH, Whaley KJ, Zeitlin L, Humphrys MS, Ravel J, et al. Herpes simplex virus-binding IgG traps HSV in human cervicovaginal mucus across the menstrual cycle and diverse vaginal microbial composition. Mucosal Immunol. 2018;11:1477–86. doi:https://doi.org/10.1038/s41385-018-0054-z.
- Wang YY, Kannan A, Nunn KL, Murphy MA, Subramani DB, Moench T, Cone R, Lai SK. IgG in cervicovaginal mucus traps HSV and prevents vaginal Herpes infections. Mucosal Immunol. 2014;7:1036–44. doi:https://doi.org/10.1038/mi.2013.120.
- Celli JP, Turner BS, Afdhal NH, Keates S, Ghiran I, Kelly CP, Ewoldt RH, McKinley GH, So P, Erramilli S, et al. Helicobacter pylori moves through mucus by reducing mucin viscoelasticity. Proc Natl Acad Sci USA. 2009;106:14321–26. doi:https://doi.org/10.1073/pnas.0903438106.
- Schroeder HA, Newby J, Schaefer A, Subramani B, Tubbs A, Gregory Forest M, Miao E, Lai SK. LPS-binding IgG arrests actively motile salmonella typhimurium in gastrointestinal mucus. Mucosal Immunol. 2020;13:814–23. doi:https://doi.org/10.1038/s41385-020-0267-9.
- Sharma A, Raman V, Lee J, Forbes NS. Mucus blocks probiotics but increases penetration of motile pathogens and induces TNF‐α and IL‐8 secretion. Biotechnol Bioeng 2020;117:2540–55. doi:https://doi.org/10.1002/bit.27383.
- Grönblad EA. Concentration of immunoglobulins in human whole saliva: effect of physiological stimulation. Acta Odontol Scand 1982;40:87–95. doi:https://doi.org/10.3109/00016358209041120.
- Brandtzaeg P. Secretory immunity with special reference to the oral cavity. J Oral Microbiol. 2013;5:20401. doi:https://doi.org/10.3402/jom.v5i0.20401.
- Spataforaa M, Mirabella A, Rossi GA, Bonanno A, Riccobono L, Carini C, Sacco O, Ravazzoni C. Lung inflammation in sarcoidosis: analysis of immunoglobulin levels in bronchoalveolar lavage fluid in active and inactive disease1. Respiration. 1985;48:127–35. doi:https://doi.org/10.1159/000194812.
- Stokes Peebles R, Liu MC, Lichtenstein LM, Hamilton RG. IgA, IgG and IgM quantification in bronchoalveolar lavage fluids from allergic rhinitics, allergic asthmatics, and normal subjects by monoclonal antibody-based immunoenzymetric assays. J Immunol Methods. 1995; (vol. 179, pp. 77–86)
- Samson RR, Mcclelland DBL, Shearman DJC. Studies on the quantitation of immunoglobulin in human intestinal secretions. Gut. 1973;14:616–26. doi:https://doi.org/10.1136/gut.14.8.616.
- O’mahony S, Barton JR, Crichton S, Ferguson A. Appraisal of gut lavage in the study of intestinal humoral immunity. Gut. 1990;(vol. 31, pp. 1341–1344)
- O’mahony S, Choudari CP, Barton JR, Walker S, Ferguson A. Gut lavage fluid proteins as markers of activity of inflammatory bowel disease. Scand J Gastroenterol. 2009. doi:https://doi.org/10.3109/00365529108996246.
- Hein M, Petersen AC, Helmig RB, Uldbjerg N, Reinholdt J. Immunoglobulin levels and phagocytes in the cervical mucus plug at term of pregnancy. Acta Obstet Gynecol Scand 2005;84:734–42. doi:https://doi.org/10.1111/j.0001-6349.2005.00525.x.
- Usala S, Usala F, Haciski R, Holt JA, Schumacher GF. IgG and IgA content of vaginal fluid during the menstrual cycle - PubMed. J Reprod Med 1989;34:292.
- Bouvet J-P, Belec L, Piris R, Pillot J. Immunoglobulin G antibodies in human vaginal secretions after parenteral vaccination. Infection and immunity; 1994.
- Delacroix DL, Dive C, Rambaud JC, Vaerman JP. IgA subclasses in various secretions and in serum. Immunology. 1982;47:383–85.
- Marasco WA, Sui J. The growth and potential of human antiviral monoclonal antibody therapeutics. Nat Biotechnol. 2007;25:1421–34. doi:https://doi.org/10.1038/nbt1363.
- Brogan TD, Ryley HC, Allen L, Hutt H. Relation between sputum sol phase composition and diagnosis in chronic chest diseases. Thorax. 1971;26:418–23. doi:https://doi.org/10.1136/thx.26.4.418.
- CREETH JM. Constituents of mucus and their separation. Br Med Bull 1978;34:17–24. doi:https://doi.org/10.1093/oxfordjournals.bmb.a071454.
- Kremer J, OPINION: JS. The significance of antisperm antibodies for sperm-cervical mucus interaction*. Hum Reprod 1992;7:781–84. doi:https://doi.org/10.1093/oxfordjournals.humrep.a137737.
- Phalipon A, Cardona A, Kraehenbuhl J-P, Edelman L, Sansonetti PJ, Corthésy B. Secretory component: a new role in secretory IgA-mediated immune exclusion in vivo. Immunity. 2002;17:107–15. doi:https://doi.org/10.1016/S1074-7613(02)00341-2.
- Fahrbach KM, Malykhina O, Stieh DJ, Hope TJ. Differential binding of IgG and IgA to mucus of the female reproductive tract. PLoS One. 2013;8:e76176. doi:https://doi.org/10.1371/journal.pone.0076176.
- Saltzman WM, Radomsky ML, Whaley KJ, Cone RA. Antibody diffusion in human cervical mucus. Biophys J 1994;66:508–15. doi:https://doi.org/10.1016/S0006-3495(94)80802-1.
- Wang, Y. Y., Schroeder, H. A., Nunn, K. L., Woods, K., Anderson, D. J., Lai, S. K., & Cone, R. A. Diffusion of immunoglobulin g in shed vaginal epithelial cells and in cell-free regions of human cervicovaginal mucus. PLoS One. 2016;11(6). https://doi.org/https://doi.org/10.1371/journal.pone.0158338
- Newby J, Schiller JL, Wessler T, Edelstein J, Forest MG, Lai SK. A blueprint for robust crosslinking of mobile species in biogels with weakly adhesive molecular anchors. Nat Commun. 2017;8(833). doi:https://doi.org/10.1038/s41467-017-00739-6.
- Baltz JM, Katz DF, Cone RA. Mechanics of sperm-egg interaction at the zona pellucida. Biophys J 1988;54:643–54. doi:https://doi.org/10.1016/S0006-3495(88)83000-5.
- Zhu P, Liu J, Bess J, Chertova E, Lifson JD, Grisé H, Ofek GA, Taylor KA, Roux KH. Distribution and three-dimensional structure of AIDS virus envelope spikes. Nature. 2006;441:847–52. doi:https://doi.org/10.1038/nature04817.
- Ruigrok RWH, Andree PJ, Hooft Van Huysduynen RAM, Mellema JE. Characterization of three highly purified influenza virus strains by electron microscopy. J Gen Virol 1984;65:799–802. doi:https://doi.org/10.1099/0022-1317-65-4-799.
- Wang YY, Harit D, Subramani DB, Arora H, Kumar PA, Lai S. Influenza-binding antibodies immobilise influenza viruses in fresh human airway mucus. Eur Respir J. 2017;49:1601709. doi:https://doi.org/10.1183/13993003.01709-2016.
- Yang B, Schaefer A, Wang -Y-Y, McCallen J, Lee P, Newby JM, Arora H, Kumar PA, Zeitlin L, Whaley KJ, et al. ZMapp reinforces the airway mucosal barrier against ebola virus. J Infect Dis. 2018;218:901–10. doi:https://doi.org/10.1093/infdis/jiy230.
- Sam, L., Wang, Y. Y., Kannan, A., Nunn, K. L., Subramani, D. B., & Cone, R. A. (2018). Patent No. US20150284451A1. US
- Gunn BM, Schneider JR, Shansab M, Bastian AR, Fahrbach KM, Smith AD, Mahan AE, Karim MM, Licht AF, Zvonar I, et al. Enhanced binding of antibodies generated during chronic HIV infection to mucus component MUC16. Mucosal Immunol. 2016;9:1549–58. doi:https://doi.org/10.1038/mi.2016.8.
- Schiller JL, Fogle MM, Bussey O, Ker WJ, Hill DB, Lai SK. Antibody-mediated trapping in biological hydrogels is governed by sugar-sugar hydrogen bonds. Acta Biomater. 2020;107:91–101. doi:https://doi.org/10.1016/j.actbio.2020.03.002.
- McKinley SA, Chen A, Shi F, Wang S, Mucha PJ, Forest MG, Lai SK. Modeling neutralization kinetics of HIV by broadly neutralizing monoclonal antibodies in genital secretions coating the cervicovaginal mucosa. PLoS One. 2014;9:e100598. doi:https://doi.org/10.1371/journal.pone.0100598.
- Smoluchowski MV. Versuch einer mathematischen Theorie der Koagulationskinetik kolloiderLösnngen.
- Chen A, McKinley S, Wang S, Shi F, Mucha P, Forest M, Lai S. Transient antibody-mucin interactions produce a dynamic molecular shield against viral invasion. Biophys J. 2014;106:2028–36. doi:https://doi.org/10.1016/j.bpj.2014.02.038.
- Wessler T, Chen A, McKinley SA, Cone R, Forest MG, Lai SK. Using computational modeling to optimize the design of antibodies that trap viruses in mucus. ACS Infect Dis. 2016;2:82–92. doi:https://doi.org/10.1021/acsinfecdis.5b00108.
- Henry CE, Wang -Y-Y, Yang Q, Hoang T, Chattopadhyay S, Hoen T, Ensign LM, Nunn KL, Schroeder H, McCallen J, et al. Anti-PEG antibodies alter the mobility and biodistribution of densely PEGylated nanoparticles in mucus. Acta Biomater. 2016;43:61–70. doi:https://doi.org/10.1016/j.actbio.2016.07.019.
- Schiller JL, Marvin A, McCallen JD, Lai SK. Robust antigen-specific tuning of the nanoscale barrier properties of biogels using matrix-associating IgG and IgM antibodies. Acta Biomater. 2019;89:95–103. doi:https://doi.org/10.1016/j.actbio.2019.03.023.
- Roche AM, Richard AL, Rahkola JT, Janoff EN, Weiser JN. Antibody blocks acquisition of bacterial colonization through agglutination. Mucosal Immunol. 2015;8:176–85. doi:https://doi.org/10.1038/mi.2014.55.
- Holmgren J, Czerkinsky C. Mucosal immunity and vaccines. Nat Med 2005;11:S45. doi:https://doi.org/10.1038/nm1213.
- Mantis NJ, Rol N, Secretory Iga’s CB. complex roles in immunity and mucosal homeostasis in the gut. Mucosal Immunol. 2011;4:603–11. doi:https://doi.org/10.1038/mi.2011.41.
- Childers NK, Bruce MG, McGhee JR. Molecular mechanisms of immunoglobulin A defense. Ann Rev Microbiol. 1989;43:503–36. doi:https://doi.org/10.1146/annurev.mi.43.100189.002443.
- Imai H, Chen A, Wyatt RJ, Rifai A. Lack of complement activation by human IgA immune complexes. Clin Exp Immunol 1988;73:479–47983.
- Belyakov IM, Ahlers JD. Simultaneous approach using systemic, mucosal and transcutaneous routes of immunization for development of protective HIV-1 vaccines. Curr Med Chem 2011;18:3953–62. doi:https://doi.org/10.2174/092986711796957293.
- Alexander R, Mestecky J. Neutralizing antibodies in mucosal secretions: igG or IgA? Curr HIV Res 2007;5:588–93. doi:https://doi.org/10.2174/157016207782418452.
- Balazs AB, Chen J, Hong CM, Rao DS, Yang L, Baltimore D. Antibody-based protection against HIV infection by vectored immunoprophylaxis. Nature. 2012;481:81–86. doi:https://doi.org/10.1038/nature10660.
- Hur EM, Patel SN, Shimizu S, Rao DS, Gnanapragasam PNP, An DS, Yang L, Baltimore D. Inhibitory effect of HIV-specific neutralizing IgA on mucosal transmission of HIV in humanized mice. Blood. 2012;120:4571–82. doi:https://doi.org/10.1182/blood-2012-04-422303.
- Chen, A., McKinley, S. A., Shi, F., Wang, S., Mucha, P. J., Harit, D., Forest, G., Lai, S. K. (2015). Modeling of virion collisions in cervicovaginal mucus reveals limits on agglutination as the protective mechanism of secretory immunoglobulin A. PLoS One. 2015;10(7). https://doi.org/https://doi.org/10.1371/journal.pone.0131351
- Gallo SA, Wang W, Rawat SS, Jung G, Waring AJ, Cole AM, Lu H, Yan X, Daly NL, Craik DJ, et al. θ-defensins prevent HIV-1 Env-mediated fusion by binding gp41 and blocking 6-helix bundle formation. J Biol Chem. 2006;281:18787–92. doi:https://doi.org/10.1074/jbc.M602422200.
- Layne SP, Merges MJ, Dembo M, Spouge JL, Conley SR, Moore JP, Raina JL, Renz H, Gelderblom HR, Narat PL, et al. Factors underlying spontaneous inactivation and susceptibility to neutralization of human immunodeficiency virus. Virology. 1992;189:695–714. doi:https://doi.org/10.1016/0042-6822(92)90593-E.
- Wald, A., Langenberg, A. G. M., Link, K., Izu, A. E., Ashley, R., Warren, T., Tyring, S., Douglas, Jr, J. M., Corey, L. Effect of condoms on reducing the transmission of herpes simplex virus type 2 from men to women. J Am Med Assoc 2001;285:3100–06. doi:https://doi.org/10.1001/jama.285.24.3100
- Collins SI, Mazloomzadeh S, Winter H, Rollason TP, Blomfield P, Young LS, Woodman CBJ. Proximity of first intercourse to menarche and the risk of human papillomavirus infection: a longitudinal study. Int J Cancer. 2005;114:498–500. doi:https://doi.org/10.1002/ijc.20732.