ABSTRACT
As an acute respiratory infectious disease, COVID-19 threatens the safety of global public health. Given the current lack of specific treatment against this disease, research and development of vaccines have become sharp weapons for overcoming the pandemic. mRNA vaccines have become the lead in COVID-19 vaccination strategies due to their advantages, such as rapid industrial production and efficacy. A total of 137 COVID-19 vaccines have entered the clinical trial stage, among which 23 are mRNA vaccines, accounting for 17% of the total vaccines. Herein, we summarize the research and developmental processes of mRNA vaccines as well as the approach for protecting the human body against infection. Focusing on the latest clinical trial data of two COVID-19 mRNA vaccines from Pfizer and Modena, we discuss their effectiveness and safety. Finally, we analyze the challenges and problems that mRNA vaccines face in controlling the COVID-19 pandemic.
1. Introduction
The 2019 coronavirus disease (COVID-19) pandemic caused by the new severe acute respiratory syndrome coronavirus 2 (SARS-CoV-2) has exerted a tremendous impact on human beings. Thus, it is particularly important to quickly develop a safe and effective vaccine to prevent coronavirus disease.
Many studies have shown that mRNA vaccines are safe and effective in many infectious diseases such as Acquired Immunodeficiency Syndrome (AIDS),Citation1 rabies,Citation2 and in cancer malignancies.Citation3 Phase I clinical trials of the first mRNA vaccines of H10N8, H7N9 influenza viruses and SARS-CoV-2 showed that the vaccine was well-tolerated and triggered a strong humoral immune response.Citation4,Citation5 Unlike DNA vaccines, mRNA vaccines are not embedded in the host DNA sequence, so they are safer.Citation6–8 In addition, the industrial production of mRNA vaccine is very rapid.Citation9–11 Over one billion doses can be produced by simple amplification technology, and the cost is low; thus, it can address large supply demands in a short period of time.Citation12 These advantages of mRNA vaccines also allow them to play a leading role in the COVID-19 pandemic.Citation13
In the past few decades, mRNA vaccines have evolved from a suspicious idea to a clinical reality. In 2020, the COVID-19 pandemic gave birth to the fastest vaccine development in history, among which mRNA vaccines were one of the earliest types that were urgently applied and popularized.Citation14 So far, 28 COVID-19 vaccines have been approved in different regions of the world, of which 3 are mRNA vaccines and they are from BioNTech-Pfizer, Moderna and Takeda which are the first to be approved to use. COVID-19 - living NMA initiative collected 461 RCTs and 222 non-randomised studies of vaccines from the International Clinical Trials Registry Platform, of which 170 studies are about mRNA vaccines. Results of many clinical trials exceeded expectations. The research of nano-transport system is very important for stabilizing mRNA, which includes lipid delivery systems (lipid nanoparticles and liposomes), polymer complexes, micelles, cationic peptides and so on.Citation15 At the same time, research on the mechanism of allergic reactions to mRNA vaccines has also attracted the attention of scientists. Contact system, complement, preexisting antibody and mast cell are gradually considered to be contributors to the allergic reactions.Citation16 In general, the prospect of mRNA vaccines is worth expectant.
Previous studies and clinical trials have shown that the BNT162b2 vaccine jointly developed by Pfizer and BioNTech and the Moderna vaccine developed by Moderna show high efficacy and good safety profiles,Citation17,Citation18 having considerable infection prevention againstSARS-CoV-2. This review quotes clinical trial data of BNT162b2 (Pfizer/BioNTech) and Moderna vaccines. While summarizing their efficacy and safety, the challenges and problems faced by mRNA vaccines are also discussed to allow acceptance of mRNA vaccines as an important reference and guide for controlling the pandemic situation of COVID-19.
2. Research and development of COVID-19 mRNA vaccine
2.1. Antigen selection
SARS-CoV-2 is an enveloped RNA virus. As a member of the coronavirus family, SARS-CoV-2 also has four major structural proteins, respectively called the spike protein S, small molecular envelope protein E, membrane protein M, and the nucleocapsid protein N. Two functional subunits, S1 and S2, are included in the S protein and play different roles. S1 subunit carries the virusʻs receptor binding domain and is involved in the adhesion of the virus to the host cell membrane surface, while S2 subunit mediates the fusion of the host cell membrane with the virus membrane.Citation19,Citation20
Located at the S1 subunit on the S protein of SARS-CoV-2, the receptor binding domain (RBD), possesses a strong affinity with the angiotensin-converting enzyme 2 (ACE2).Citation21,Citation22 Upon the binding of the S protein to ACE2 on the host cell membrane, ACE2 can be cleaved by proteases in the cell and the S protein be activated at the same time, consequently facilitating the virus membrane to fuse with the host cell membrane and enter the cell.Citation23 Therefore, the S protein is used as a target spot to develop vaccines.
Additionally, as the RBD is required for receptor docking responsible for the recognition of ACE2,Citation24 RBD is also a target for vaccine development.
In summary, both the S protein and RBD of the S protein in SARS-CoV-2 can be utilized as antigens for mRNA vaccine development ().
Figure 1. Membrane surface protein of SARS-CoV-2 and the mechanism of SARS-CoV-2 invading cells. A. S1 subunit recognizes and binds ACE2 on host cell membrane. B. Proteases from host cells disassemble ACE2 and activate S2 subunit C. Activated S2 subunit mediates the fusion of virus envelope and host cell membrane, and then the virus invades the cell.
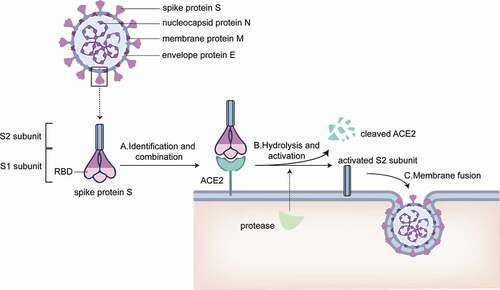
2.2. Manufacturing process
At the end of April 2021, Pfizerʻs messenger RNA vaccine manufacturing process was first introduced. Next, we will take its research and development process as an example to briefly describe the production process of mRNA vaccines (). The scientists first obtained the novel coronavirus gene sequence through high-throughput sequencing, and then selected and extracted the target gene sequence. Since novel coronavirus nucleic acid is an RNA fragment with poor stability, the scientists extracted the target fragment sequence and transformed it into a double-stranded DNA loop by reverse transcription, which was stored in the form of plasmid. After that, technicians injected plasmids into the engineered Escherichia coli host. During the process of Escherichia coli amplification in the culture medium, the plasmids were also replicated at a high speed as the raw material of the vaccine. After replication, the technicians collect the plasmid by means of purification, and then inspect its quality. After confirming that the gene sequence without mutation, the plasmid was cut and the desired DNA linear sequence was purified as well. Under the induction of key enzymes, mRNA was transcribed by using DNA template. It is well known that mRNA fragments could be easily destroyed by human cells, so the transcribed mRNA needed to be combined with lipids to form lipid nanoparticles after quality inspection. Finally, the vaccine particles were packaged in sterile injection vials, and the production of mRNA vaccine was completed.
Figure 2. Synthesis of the mRNA vaccine. A. mRNA of target protein is extracted from SARS-CoV-2. B. Targeted mRNA is transcribed into double-helix DNA through reverse transcription; C. DNA strands combine with plasmid. D. Plasmid incorporating fragments of DNA are amplified in Escherichia coli; E. the target DNA is sheared from many cloned plasmids and purified; F. the double stranded DNA (dsDNA) template is unwound by enzymes and transcribe into mRNA; G. the final assembly of the vaccine is achieved by enclosing mRNA with liposomes.
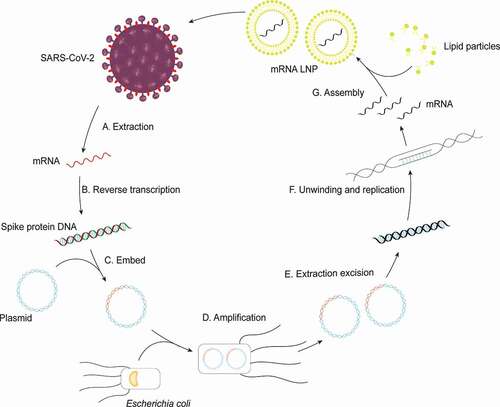
3. Mechanism of activity of COVID-19 mRNA vaccine
As early as the nineties of the 20th century, mRNA vaccines were proposed and studied by researchers. The theory, technology, and economic investment of mRNA vaccines have constantly accumulated and improved. Their underlying mechanism have increasingly been clarified and better understood by scientists. The breakthrough in the mechanism of action has allowed the novel coronavirus mRNA vaccines, which are a third-generation vaccine technology, to achieve a prominent role during this pandemic.Citation25
Currently, mRNA vaccines not only activate the exogenous immune response pathway of CD4+T cells like the inactivated vaccines, but also activates a large number of endogenous immune response pathways involving CD8+T cells, resulting in the activation of numerous of effector and memory CD8+T cells ()
Figure 3. Mechanism of inactivated vaccine and mRNA vaccine. A. Antigen synthesis stage: Myocytes capture inactivated virus from inactivated vaccine and target mRNA LNP from the mRNA vaccine; B. Antigen recognition stage: (1) Antigen presenting cell including DC cells and macrophages recognize the antigen phagocytosed from muscle cells and present it to DC cells; (2) Common features: Both vaccines activate CD4+T cell immunity through the MHC class II pathway; (3) mRNA vaccine advantages: mRNA LNP can activate more CD8+T cells because of the presence of the MHC class I pathway; C. Effector stage: CTL and B cells are activated and destroy the virus. a. Ribosome b. endoplasmic reticulum c. golgiapparatus d. lysosome.
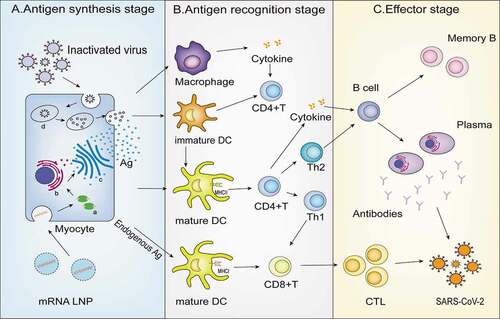
The exogenous immune response pathway of the mRNA vaccine is as follows: The mRNA vaccine is taken up by muscle cells after injection into the human body. Muscle cells translate many S protein fragments and transport them out of cells in a short time, which allows them to bind to different types of APCs, such as macrophages, and dendritic cells (DCs), to activate human immunity. Immature DC cells phagocytose S protein fragments and activate mature DC cells. At the same time, macrophages can process synthetic protein antigen information, release cytokines, and promote the maturation of DC cells. Mature DC cells splice S protein fragments and present them to CD4+T cells for recognition and binding through MHC class II molecules.Citation27 CD4+T cells activate B cells and produce plasma cells and memory B cells by directly binding and secreting cytokines, resulting in a series of downstream humoral immune effects dominated by antibodies. The mRNA vaccine can also activate the endogenous immune response pathway: both APC cells and other nucleated tissue cells can splice the S protein constituted in mRNA vaccine and then present it to CD8+T cells through MHC class I molecules on the membrane surface, thus activating CD8+T cells and producing memory and effector T cells. When reinfected with SARS-CoV-2, cellular immunity and humoral immunity are activated at the same time.Citation28–30 The memory immune cells produced by the body, such as memory B cells and antigen-specific cytotoxic T cells, can quickly recognize the virus, produce additional antibodies, accurately kill the infected cells, eliminate the virus, and prevent the continuation of infection.
4. Efficacy of COVID-19 mRNA vaccine
As a vaccine product of the latest technology, mRNA vaccines have made a historic breakthrough in the fight against COVID-19. Its clinical trial outcomes have been far beyond the public expectation. The vaccines not only exhibit a low incidence of adverse reactions, but they also hold a prominent position in clinical efficacy among all existing vaccines. The latest clinical trial data evaluating these two vaccines which highlight the superior clinical efficacy of mRNA vaccines will be briefly summarized below.
4.1. Phase III clinical trials of BNT162b2 and mRNA-1237
First, we will describe the phase III clinical trials of the BNT162b2 and mRNA-1237 vaccines. All the volunteers in this phase III trial were over age 16 years, while volunteers in mRNA-1237 trial were over 18 years.
The phase III trial of the BNT162b2 vaccine enrolled 43,548 volunteers who were randomly assigned. Compared to placebo group, BNT162B2 was 95% effective in preventing COVID-19 overall. In addition, as for prevention of severe COVID-19 infection, the preventive rate was 95% over all ages, and 94% in the elders over 65 years of age.Citation17 Moreover, the trial classified different age groups including 16–55 years, over 65 years and over 75 years. From the survey analysis mentioned in the trial, according to confidence interval (CI), we cannot clearly judge that the efficacy of the vaccine differs in different age groups. As a result, we have reasons to believe that BNT162b2 have considerable efficacy in all age group which is over 16 years.
The efficacy of the mRNA-1237 vaccine during phase III clinical trials also exceeded researchersʻ expectations. Researchers carried out III trial, which involved 30,000 people, and found that vaccination reduced the rate of infection by 94.5%. In other words, individuals who were inoculated had a 94.5% lower risk of infection than those who were not.Citation18 We compare the efficacy classified in two age subgroups. One group which individuals aged from 18 to 65 years reached vaccine efficacy of 95.6% (90.6%-97.9%). The efficacy of another group which individuals aged over 65 years was 86.4% (61.4%-95%). From the data listed above, we can draw a conclusion that even the efficacy of two vaccines differ in different groups, the protective reaction of mRNA vaccine is quite receptable over all ages.
4.2. Clinical efficiency for specific populations
4.2.1. Medical staff
As the main physical force in the fight against COVID-19, the safety of medical staff must be guaranteed. Many countries and institutions are investigating the efficacy of mRNA vaccination among medical staff. Only by effectively protecting the safety of health care workers we can continue the fight against the virus.
In a survey conducted at the largest medical center in Israel, a total of 11,453 medical personnel received two doses of mRNA vaccine from 9 December 2020 to 28 April 2021 throughout the medical center. Thirty-nine SARS-CoV-2 breakthrough infections were detected among healthcare personnel with access to RT-PCR testing. As a result of survey, we found that the breakthrough infection rate among medical personnel was very low, and most symptoms were mild to absent, albeit persistent.Citation31
Three studies published on the same day by the New England Journal of Medicine included real-world data from four medical institutions across different countries. The results of the three studies showed that the mRNA vaccine could significantly reduce the risk of Novel coronavirus infection in first-line medical staff, especially after two doses of injection. We can reach a conclusion that according to data mentioned above, after two doses of mRNA vaccines, positive infection rate of people decreases to .05% or so compared to 2.6% of unvaccinated people. After the completion of two doses of vaccine, it still could not achieve “zero risk”. Thus, researchers suggest that front-line medical staff should continue to take necessary precautions even after vaccination is completed.Citation32–35
4.2.2. Teenagers
At present, the number of teenagers infected is also increasing, and the issue of whether teenagers should be vaccinated has attracted increasing attention in various countries, especially in America.Citation36 Majority of Children and adolescents infected with SARS-COV-2 have mild symptoms, but they are prone to suffer from long-term complications that affect their growth and development. Adolescents currently spend most of their time at school or at home, although school life is relatively shut-down. However, if the number of cases among adolescents continues to rise, the pandemic will inevitably worsen.Citation37 If the age of vaccination can be widened, it will contribute to an earlier return to normal school life among children.
Participants in Pfizer/Biotechʻs vaccine trial included 2260 U.S. adolescents aged 12 to 15, 50% of whom received placebo. In the placebo group, 18 participants were later infected with COVID-19, while none of the participants who received the actual vaccine were infected, achieving 100% immunity and experiencing no serious side effects.Citation38 On 10 May 2021, the United States Food and Drug Administration (FDA) approved Pfizerʻs COVID-19 vaccine BNT162b2 for emergency use authorization (EUA) in the United States for children aged 12 to 15 years. The follow-up surveys indicated that the vaccine sustains the efficacy of 91% against SARS-COV-2.Citation39 At the same time, clinical trials under 12 years of age are gradually being carried out, and clinical results currently shown in 5–11 years of age are similar in both safety and immunogenicity to other age groups which is over 16 years.Citation40,Citation41
4.2.3. Elders
It is particularly important to develop a vaccine for the elderly, who suffer from weakened body functions and inferior immune systems. It can effectively reduce the number of critically severe cases and reduce the spread of disease. 42% of participants in the BNT162b2 phase III trial who received two consecutive doses were over 55 years of age, suggesting that the vaccine is also highly effective in elder individuals.Citation17 The phase III trial of mRNA-1237 also encounter similar result.Citation18 A clinical trial of mRNA-1273 in people aged 56 and older found that the immune response of those aged 56 and older after the vaccine was similar to that of those aged 18–55.Citation42 A clinical test conducted in China found that the elderly (65–85) could produce robust interferon-γ T cell responses. Although one analysis found lower levels of antibody production in people over 80 years of age after vaccination, in particular B.1.1.7 (Alpha), B.1.351 (Beta) and P.1 were less potent neutralizing agents.Citation43 However, the elders, a high-risk group of virus infection, it is necessary to inoculate vaccine as vaccine protection is still acceptable.Citation44
4.2.4. Individuals with a history of SARS-CoV-2 infection
The COVID-19 pandemic is rapidly spreading around the world beyond our imagination. Although many vaccines have currently become industrialized, the supply of vaccines is unable to meet the high demand for vaccines in a short time. Therefore, in the face of insufficient supply, individuals questioned whether a single dose of vaccination could effectively improve the bodyʻs immunity. Scientists first investigated individuals with previous infections.Citation45 Could the immunity of previously infected patients be fully enhanced after vaccination with a single dose? It is observed that a single dose of BNT162B2 mRNA vaccine was sufficient for individuals with a history of SARS-CoV-2 infection, according to clinical researches.Citation46–48 The researchers found that at all time points, individuals previously infected with SARS-CoV-2 presented higher antibody levels for both IgG (N) and IgG (s-RBD). Both of them represented the immune reaction to prior infection. In addition, IgG (s-RBD) also represented the immune reaction to prior infection or vaccine. Furthermore, there was no significant discrepancy in IgG (s-RBD) levels between individuals who previously infected and revived only a single dose and individuals who were uninfected before and received two doses. According to serum antibody analysis of COVID-19 patients, we found that serum-associated antibodies are closely associated with the prognosis and severity of the disease. High titers of neutralizing antibodies can help patients recover more quickly and ward off a reinfection.Citation49 Scientists are starting to develop neutralizing antibodies to prevent and treat COVID-19 infections.Citation50 Therefore, serum neutralizing antibodies highly predict that only one dose of vaccine is needed to achieve the effect of two doses of vaccine for persons previously inflected.
4.3. Neutralizing effects in the real world
As the time goes by, mRNA vaccines are being promoted in more and more countries, providing rich data support for efficient statistics of mRNA vaccines in the real world. The first two mRNA vaccines approved for use are Moderna mRNA-1273 and Pfizer-BioNTech BNT162b2, which still show excellent effectiveness in the environment of large-scale vaccination.
In 2020, studies have begun to try to count the effectiveness of mRNA vaccines in population vaccination. In a large-scale study on the effectiveness of mRNA COVID-19 vaccine among U.S. veterans from May to July 2020 (n = 6 647 733), the overall vaccine effectiveness (VE) was 95% (95% CI, 93%-96%) for complete vaccination (≥14 days after the second dose) and 64% (95% CI, 59%-68%) for partial vaccination (≥14 days after the first dose) and the estimated VE for two doses of vaccination against COVID-19-related hospitalizations is 91% (95% CI 83%-95%).Citation51 In the same study of American veterans, a case-control study (n = 108 720) from 15 December 2020 to 4 March 2021 showed that the overall vaccine effectiveness was 97.1% (95% CI, 96.6% to 97.5%) injected with the second dose after 7 days or more.Citation52 This study also reached a more detailed conclusion that the VE of Moderna mRNA-1273 and Pfizer-BioNTech BNT162b2 were 96.2% (CI, 95.5% to 96.9%) and 98.2% (CI, 97.5% to 98.6%), respectively.Citation52
It is worth celebrating that by the end of 2021, similar results have been obtained in the vaccine effectiveness studies in the context of extensive mRNA vaccination in many regions and countries such as the United Kingdom, Italy, and Israel.Citation46,Citation53,Citation54
In addition, the number of inoculation doses and the time after inoculation are very important influencing factors for VE. Studies have shown that the more doses of Pfizer-BioNTech BNT162b2 are administered, the more effective the vaccine is; and as the time of inoculation of Pfizer-BioNTech BNT162b2 increases, the VE will gradually decrease.Citation55–58 In particular, the interim results of a prospective observational cohort study indicate that in individuals with a history of COVID-19, vaccination with Moderna mRNA-1273 may provide additional protection beyond the immunity gained from previous infections, with a VE range of 8.2%-33.6%.Citation59
4.4. Mutant virus variants
Over time, novel Coronavirus variants have spread throughout the world. To escape the attack of the human immune system in different environments, the virus has evolved different mutant variants, which have greatly altered the pathogenic potential of the virus and have enhanced concern regarding the development of effective vaccines. As of December 2021, more than 10 mutant variants have been identified by the World Health Organization (WHO),Citation60 which four are highly virulent, widely diffused, and have attracted widespread public attention, namely variants Alpha (B.1.1.7), Beta (B.1.351), Gamma (P.1), and Delta (B.1.617.2).Citation61 Here are some clinical trials testing the efficacy of mRNA vaccines defending mutants.
As for the alpha variant, the results of the recently published vaccineʻs efficacy tracking displayed a 93.7% prevention rate against the mutant strain after two doses of Pfizer vaccine.Citation62 As for Beta mutant strain, two real-world studies in Qatar determined that two doses of the Pfizer and Modena vaccines were 75% and 96.4% effective against the mutant strain, respectively.Citation62,Citation63 However, over 95% of severe cases were averted, and the Pfizer vaccine even achieved a 100% protection rate.Citation63 Thus, it is not difficult to conclude that despite the obvious immune escape potential of the mutant strain, the currently developed mRNA vaccines still present good preventive and protective effects. As for Gamma, investigations showed that the Gamma strain spread 1.7 to 2.4 times faster than the non-Gamma strain, and that prior (non- Gamma) infection did not provide 100% protection against Gamma infection, with immune escape rates ranging from 21% to 46%.Citation64 In a recent real-world study in Chile, the Pfizerʻs vaccine was 92.6% effective. As for Delta, with regard to the strongest mutant strain Delta, the BNT162b2 vaccine also holds a certain effective defense against it. A clinical trial compared patients infected with Alpha and Delta mutants who were inoculated with the BNT162b2 vaccine. The results of the trial showed that the efficacy of the vaccine was significantly lower in those infected with the Delta variant than in those infected with the Alpha variant when only one dose was administered. After two doses, however, the efficacy was almost identical.Citation65
In addition to the statistical analysis of cases, researchers have also measured the efficacy of the mRNA vaccine by measuring antibody levels in the serum of vaccinated individuals. An experimental study by Pfizer and the Texas University School of Medicine revealed that antibodies produced by mRNA coronavirus vaccine against the three mutant strains: Alpha(B.1.1.7), Beta(B.1.351)and Gamma(P.1), still present great neutralization efficacy.Citation66 One study assessed the neutralization ability of serum from human subjects or non-human primates (NHP) inoculated with mRNA-1273 against strains Alpha and Beta. The results showed that the neutralization ability of the immune serum to the virus strain Alpha and Gamma did not decrease, while the neutralization ability of the immune serum to the virus strain Beta decreased, but remained constant at a high level.Citation67
In general, although several highly infectious variants have emerged, such as Omicron (B.1.1.529), studies have confirmed that the currently developed mRNA vaccines have considerable efficacy against virus mutants. While there are concerns about the vaccineʻs reduced protection against mutant strains, we should not ignore the fact that immunity decreases over time after vaccination.Citation68 As a result, the decrease in vaccine efficacy should not be entirely due to the mutation avoiding immune protection, but may be due to the time. To effectively deal with mutations, in addition to reducing the spread of the virus and ensure personal protection, individuals should actively advocate vaccination, control the pandemic as early as possible, and avoid the generation of mutant strains. Furthermore, it is also necessary to inoculate the third dose of vaccine to reach the purpose above.Citation56,Citation69
4.5. Retransmission inhibition
The BNT162b2 mRNA vaccine not only provides protection to the vaccinees, but also effectively reduces the viral load in infection regions characterized by Coronavirus outbreaks, which allows to further inhibit the retransmission of the virus. In an analysis of data sets that tested positive for SARS-CoV-2 after vaccination with the BNT162b2 mRNA vaccine, researchers found a significant reduction in viral load in infections that occurred 12 to 37 days after the initial vaccination. These reductions in viral loads suggest that vaccinated individuals are less infectious after re-infection with coronavirus compared to non-vaccinated individuals, further illustrating the impact of the vaccine on viral transmission.Citation70
5. Safety of COVID-19 mRNA vaccine
5.1. Adverse reaction of the vaccine
The Journal of the American Medical Association of the Center for Disease Control and Prevention (CDC) analyzed local and systemic response data voluntarily reported by 3.644 million people vaccinated with mRNA coronavirus vaccines from 14 December 2020, to 28 February 2021. Statistical data indicated that adverse events of varying severity may occur after vaccination with mRNA vaccine for COVID-19. As with vaccines for other diseases, pain at the injection site after vaccination is common and generally lasts up to 2 days.Citation71–74 In addition, the main systemic reactions are fatigue, headache, muscle pain, fever, while other adverse events are less frequent, such as chills, fever, joint pain, nausea, vomiting, local redness and swelling of the skin.Citation18,Citation75–77 Moreover, some common non-allergic symptoms such as pruritus, rash, scratchy throat and mild respiratory symptoms have also been reported.Citation78 But in general, most of these adverse reactions are temporary and will disappear within a short time.
Besides, some clinical studies have found that the occurrence of adverse events (AEs) in different groups is different. For example, AEs is more common in young adults, women, and people who received the second dose than in older adults, men, and people who received the first dose.Citation53,Citation79–82 In contrast, since the immune response of young people and women is generally more active, and the response will be more reactive after the second dose of the mRNA vaccine, we consider that the stronger the immune response in the human body, the greater the possibility of adverse reactions after vaccination.
In addition, the CDCʻs Vaccine Adverse Event Reporting System found that the incidence of severe anaphylaxis was 5 cases per million population and 2.8 cases per million population after the first injection of Pfizer vaccine and mRNA-1273 vaccine, suggesting that serious adverse reactions were rarer with the mRNA-1273 vaccine.
Reports of hypersensitivity reactions to COVID-19 vaccines can be divided into immediate and delayed reactions. Among them, the former is equivalent to anaphylaxis. Most of these patients have a history of allergic reaction, which usually occurs within the observation window of 30 minutes after inoculation and may be life-threatening, requiring immediate treatment.Citation83 Delayed large local reactions were most common after administration of mRNA-1273 vaccine.Citation78,Citation84 At the same time, further analysis of the components of the mRNA vaccine showed that the active components will not cause allergic reactions of the vaccine, but the excipient is the allergic sources, such as polyethylene glycol (PEG) and polysorbate. However, these excipients are essential to stabilize the lipid nanoparticles containing the mRNA, stimulate stronger immune response, and prevent bacterial contamination.Citation83,Citation85 In the future, the development of excipients with weak sensitization may become one of the important research directions of vaccine research and development institutions.
Furthermore, a number of rare serious untoward reactions, which were thought to be possibly related to the immunizations COVID-19 vaccine, were reported by different countries in succession in 2021, such as thrombosis with thrombocytopenia syndrome [TTS], Guillain-Barré syndrome [GBS], myocarditis, pericarditis, and myocardial infarction.Citation84,Citation86,Citation87 The above adverse reactions have aroused great concern and need to be confirmed by further studies. The occurrence of myocarditis has attracted much attention. According to the Advisory Committee on Immunization Practices (ACIP) statistical data, as of 11 June 2021, 1226 cases of possible myocarditis/pericarditis had been reported after about 300 million doses of COVID − 19 mRNA vaccine.Citation88 Clinical observation found that the incidence of myocarditis was concentrated in young men, aged between 16 and 29 years old.Citation82,Citation89,Citation90 Additionally, the latest study found an increased risk of myocarditis events in three cases: within 1 week after receiving the first dose of mRNA or adenovirus vaccine, after SARS-COV-2 infection in the same population, and after vaccination for people under 40 years old.Citation91 Concerns about these adverse events have led several countries to suspend mRNA vaccination for young people, limiting the popularization of vaccines.Citation92
Nevertheless, as more clinical trials were completed, the results show that the mRNA vaccineʻs safety is still satisfactoryCitation93and the mRNA vaccine has a good safety profile in terms of the response of recipients.Citation17,Citation94 Since 26 May 2021, the Global Advisory Committee on Vaccine Safety (GACVS) has declared that although myocarditis and pericarditis may be causally related, severe cases are rare and most cases are mild after all.Citation82 Meanwhile, at the ACIP meeting in July 2021, after assessing the balance of benefits and risks of the mRNA vaccine for COVID-19 in adolescents and young adults, it was concluded that the benefits of vaccination with mRNA vaccine significantly outweigh the risk of rare serious adverse events following vaccination, and that all eligible individuals were recommended to be actively vaccinated against COVID-19.Citation82,Citation86
According to the latest vaccination schedule, Pfizer BioNTechʻs BNT162b2 mRNA vaccine showed excellent safety and efficacy in phase 2/3 clinical trials in children aged 5–11 years. Therefore, people over the age of five are encouraged to vaccinate COVID-19 vaccine as soon as possible. In the context of the current global pandemic, COVID-19 vaccines are advantageous in preventing the occurrence and mortality of COVID-19 globally, as well as preventing the occurrence of COVID 19-related complications such as ARDS and myocarditis. Thus, vaccination should continue to be actively promoted around the world to jointly promote rapid global vaccine deployment.
5.2. Safety of the vaccine for pregnant women
There has been a long and heated debate about whether pregnant women should be vaccinated. Determining whether to vaccinate pregnant women should take into consideration not only the impact of the vaccine on womanʻs own health, but also the potential danger to the fetus.Citation95 Several recent experimental studies have shed light on whether advantages of mRNA vaccines outweigh the disadvantages in pregnant women. A mouse experiment showed that mice injected with the BNT162b2 vaccine induced a significant immune response, and the presence of neutralizing antibodies was also detected in fetuses and progeny. No effect of BNT162b2 on female fertility was observed. Local side effects were also similar to those found in clinical trials.Citation96 The results also enabled clinical trials to include pregnant women to be inoculated with COVID-19 mRNA vaccine and to proceed smoothly. A clinical trial enrolled total of 35,691 pregnant volunteers, and the outcomes of pregnancies of participants enrolled in the V-SAFE pregnancy registry were similar to those in the pre-pandemic study. Compared with non-pregnant women, pregnant women reported more pronounced local pain, while headaches, muscle aches, chills, and high fever seemed to be weaker. Therefore, based on the results of this study, we did not find any evidence that mRNA produced serious adverse reactions in pregnant women.Citation97 Of course, participants will still need to be followed longitudinally to obtain more accurate data and results.
5.3. Safety of the vaccine for teenagers
By the end of December 2021 in the United States, more than 21 million adolescents under the age of 18 had received the mRNA vaccines at least one dose. The injection number of adolescents under 18 years is expected to increase further as children aged 5–11 years are also approved for vaccination in November. As a result, concerns about the safety of mRNA vaccines in adolescents are rasised sequentially. Many clinical trials and surveys have been carried out to reveal the safety of mRNA vaccines in adolescents. The Vaccine Adverse Event Reporting System (VAERS) which administered by the CDC and FDA, collected 9,246 data reports from 12 to 17 years. The conclusion is that majority of adverse reactions (90.7%) are mild, and only 9.3% are considered serious, including 4.3% for myocarditis.Citation98 This means that, with the exception of myocarditis, multiple serious adverse reactions are considered rare. Two representative mRNA vaccines, BNT162b2 and mrnA-1273, also implemented placebo-controlled trials researching for adverse effects in adolescents.Citation99,Citation100 The results showed that all adverse reactions are common. Among them, injection-site pain, fatigue, and headache are most accessible. Furthermore, both trials found no serious adverse events associated with the mRNA vaccine.Citation99,Citation100 All studies indicate that mRNA vaccines may not bring serious adverse reactions to teenagers, and also reduce the hospitalization rate of severe infection and promote recovery.Citation101
5.4. Safety data from long-term monitoring
The long-term safety of the mRNA vaccine is worth expectation. Safety data from a phase 3 clinical trial with a median follow-up of two months after vaccination with the BNT162b2 mRNA vaccine showed that both local reactions and systemic reactions to the BNT162b2 mRNA vaccine were predominantly mild to moderate, with no grade four local reactions. The percentage of participants reporting local adverse events did not increase after vaccinating the second dose. Meanwhile, apart from fatigue and headache, the frequency of reported serious systemic events after vaccinating any one dose was less than 2%. Additionally, in the vaccine group and the placebo group, the incidence of serious adverse events was similar.Citation17 Researchers followed 12,006 participants in this clinical trial after the second dose of vaccination with the BNT162b2 mRNA vaccine for at least six months. The results of the follow-up showed that the safety data obtained from six months after vaccination were similar to those obtained from two months of previous follow-up; at the same time, none of the cases of myocarditis were identified.Citation39 The current study results suggest that the mRNA vaccine has a good long-term safety profile. Before the outbreak of COVID-19, no vaccines have been approved for human trials. Therefore, whether there are some potential adverse reactions after injection is indistinct. The excitement is that serious adverse reactions reported so far are rare, sporadic, and unrepresentative. As the follow-up period extends, more data will be available to demonstrate the safety of mRNA vaccines. But in the background of COVID-19 pandemic, the popularization of mRNA vaccines should not be affected by this concern.
6. Stability of COVID-19 mRNA vaccination
In addition to the vaccineʻs efficacy, many people are also concerned about how long the effect of the vaccine lasts and when revaccination will be required. If the duration of the vaccine effect is too short, efficacy cannot be maintained over a substantial period, even if the efficacy is strong, the clinical significance is not extensive. It is of interest that Moderna has recently released phase III data, showing that six months after completing the second dose, the vaccine was still more than 90% effective against the virus. The Pfizer vaccine has also been investigated and follow-up data from a trial of 46,307 participants showed that the vaccine was still 91.3% effective six months after one week of the second dose. In the United States, the efficacy rate was 92.6%.Citation102
Previously, the timeliness of vaccines was mostly evaluated by monitoring antibody levels in the peripheral blood. Recently, Jackson et al. focused their research on germinal center (GC), the birthplace of immune responses. The GC is the region where the immune memory is formed. Compared with the antibody, as the final product of the immune response, monitoring the GC can more effectively evaluate whether the human body effectively produces lasting immunity. The GC is the key to a lasting immune response, and the longer it exists, the longer the immune response lasts. This study shows that mRNA vaccines can induce a sustained GC B cell response, which continues to produce strong humoral immunity. This further demonstrates that mRNA vaccine can provide long-term protection to humans.Citation103
7. Discussion
7.1. Clinical efficiency
Due to the rapid spread of the COVID-19 pandemic, vaccines are urgently being developed and have been approved for use. Vaccine efficiency is currently based on results of phase III clinical trials. Phase III clinical trials involving the Pfizer and Moderna vaccines have revealed that the effective vaccine protection rate is approximately 95%. Currently, the efficacy rate of mRNA vaccine is much higher than that of inactive vaccines. However, at present, there is a lack of a standardized evaluation system for evaluating infection or vaccine efficiency in the world. For example, the symptoms that can be diagnosed as infection, are defined differently in different vaccine studies. In addition, the diagnosis generally depends on nucleic acid positive results, but if individuals in the trial do not undergo nucleic acid testing, despite the high likelihood of an infection, this will affect the accuracy of the trial. At the same time, in addition to paying attention to confirmed cases, suspected cases are also worthy of our attention. If there are too many suspected cases, this will introduce significant uncertainty to the trial results. Further, the number of individuals enrolled in trials are still limited compared to target population around the world, and with the current increase in the number of vaccinated individuals, vaccine efficiency will inevitably begin to fluctuate. Moreover, we need to consider differences in the efficacy of the vaccine in different trial groups, and whether the vaccine can prevent the transmission of coronavirus in COVID-19 patients with only mild symptoms.Citation104,Citation105
7.2. Transportation and storage
The stringent conditions required for the transport and storage of currently available vaccines is a central problem to be overcome in the promotion of mRNA vaccines. According to the temperature of storage conditions and shelf life of the mRNA COVID-19 vaccine published by the vaccine manufacturers, Pfizer vaccines are valid for six months when stored between −80 and −60°C but only for five days at 2 to 8°C At room temperature, its shelf life is greatly shortened to only two hours. The Moderna vaccine has made a breakthrough in the research and development of preparations, and its product can be stored at 2–8°C for 30 days, but only for 12 hours at room temperature.Citation106 Yet for economic constraints, it is tough to achieve such low-temperature preservation conditions in many countries, which hinders the promotion of the mRNA vaccine. How to improve the stability of mRNA vaccine has become an urgent problem due to scientists. Some researchers have determined that purified mRNA can maintain its activity at 4°C for up to 10 months after freeze-drying in trehalose, and its stability is similar to that of the traditional vaccines. Moreover, others have proposed to enhance the stability of the mRNA vaccine by modifying the preparation.Citation107 Relevant studies have found that various chemical modifications of mRNA through sequences and/or structural engineering contribute to enhance the stability of mRNA, such as extension of poly (A) tail, modification of the 5'cap, editing of UTRs, increasing the G-C fraction of mRNA, and so on.Citation108–111
Currently, sufficient progress has been made in the application of mRNA vaccine in influenza and rabies. The mRNA vaccine of influenza virus HA maintains its protective effect after being stored at 37°C for 3 weeks.Citation12 Scientists found that the mRNA vaccine encoding rabies virus glycoprotein (RABV-G) still retained immunogenicity after long-term storage at 2–8°C 25°C and 37°C in the phase I trial of mRNA rabies virus vaccine CV7201. At the same time, it can also be used as a lyophilized preparation, which can be stored for 3 years at 5°C to 25°C and 6 months at 40°C without significant loss of activity.Citation29,Citation112 Thus, we have reason to believe that in the future, it will not be too difficult to resolve problems related to transportation and storage.
7.3. Facing the mutant
Although the mRNA COVID-19 vaccine has a certain neutralizing effect against mutant novel coronavirus, it is particularly important to significantly improve the neutralizing ability. A clinical trial conducted by Moderna Company showed that among volunteers who had received two doses of mRNA-1273 vaccine, the third dose of COVID-19 vaccine could significantly improve the neutralization ability against COVID-19 mutant strains B.1.351 and P.1. Fifteen days after inoculation with mRNA- 1273. 35,115 designed for B.1.351 mutant virus, the Geometric mean titer (GMT) of the inoculant serum to B.1.351 was 1400, while the value for inoculated mRNA-1273 was 864. These preliminary data suggest that mRNA-1273.351 may more effectively enhance the neutralization ability of B.1.351 strain. In addition, local and systemic adverse reactions after the third dose of the vaccine were similar to those after the second dose of the vaccine in phase II and phase III clinical trials. Thus, mRNA vaccines exhibit a larger breakthrough space and better protective efficacy in the face of mutant strains. Due to the more rapid industrial preparation of mRNA vaccines, it can be produced in large quantities in a short time. By splicing and synthesizing the gene sequences of different mutant strains, we can prevent multiple mutant strains by inoculating only a single vaccine. Nonetheless, new vaccines require to undergo a series of clinical trials. It remains to be seen whether the optimization of the mRNA COVID-19 vaccine can catch up with the speed of virus mutation and defeat the virus.
8. Conclusions
As a new technology vaccine, mRNA vaccines present good prospects for more widespread application. In this COVID-19 pandemic, the data of existing clinical trials has proved that compared with other COVID-19 vaccines, the COVID-19 mRNA vaccine has a higher protection rate and safety, less side effects, and good defense against mutated viruses. Thus, it is reasonable to propose that mRNA vaccines will become an effective weapon to defeat novel coronaviruses. In addition, the emergence of the COVID-19mRNA vaccine has promoted rapid progress in the field of vaccine research and development, and mRNA technology platform is likely to provide novel formulations for the development of vaccines for other infectious disease, which is expected to exert a positive “subversive” impact on vaccine development.
Author contributions
P.D. Zhang and H.L. Wang conceptualized and designed the study. Peixian Chen and Xiaoye Shi performed the literature search, drafted the manuscript and contributed equally to this manuscript. Weixin He and Yan Tang participated in manuscript writing and data collecting. Guowei Zhong were responsible for the formatting and image editing. All authors critically revised the manuscript and approved the final version.
Disclosure statement
No potential conflict of interest was reported by the author(s).
Correction Statement
This article has been corrected with minor changes. These changes do not impact the academic content of the article.
Additional information
Funding
References
- Moyo N, Vogel AB, Buus S, Erbar S, Wee EG, Sahin U, Hanke T. Efficient induction of T cells against conserved HIV-1 regions by mosaic vaccines delivered as self-amplifying mRNA. Mol Ther Methods Clin Dev. 2019;12:32–13. doi:10.1016/j.omtm.2018.10.010.
- Schnee M, Vogel AB, Voss D, Petsch B, Baumhof P, Kramps T, Stitz L. An mRNA vaccine encoding Rabies virus glycoprotein induces protection against lethal infection in mice and correlates of protection in adult and newborn pigs. PLoS Negl Trop Dis. 2016;10(6):e0004746. doi:10.1371/journal.pntd.0004746.
- Cafri G, Gartner JJ, Zaks T, Hopson K, Levin N, Paria BC. mRNA vaccine–induced neoantigen-specific T cell immunity in patients with gastrointestinal cancer. J Clin Invest. 2020;130(11):5976–88. doi:10.1172/jci134915.
- Feldman RA, Fuhr R, Smolenov I, Mick Ribeiro A, Panther L, Watson M, Senn JJ, Smith M, Almarsson Ӧ, Pujar HS, et al. mRNA vaccines against H10N8 and H7N9 influenza viruses of pandemic potential are immunogenic and well tolerated in healthy adults in phase 1 randomized clinical trials. Vaccine. 2019;37(25):3326–34. doi:10.1016/j.vaccine.2019.04.074.
- Mulligan MJ, Lyke KE, Kitchin N, Absalon J, Gurtman A, Lockhart S, Neuzil K, Raabe V, Bailey R, Swanson KA. Phase I/II study of COVID-19 RNA vaccine BNT162b1 in adults. Nature. 2020;586(7830):589–93. doi:10.1038/s41586-020-2639-4.
- Wong SS, Webby RJ. An mRNA vaccine for influenza. Nat Biotechnol. 2012;30(12):1202–04. doi:10.1038/nbt.2439.
- Karikó K, Muramatsu H, Welsh FA, Ludwig J, Kato H, Akira S, Weissman D. Incorporation of pseudouridine into mRNA yields superior nonimmunogenic vector with increased translational capacity and biological stability. Mol Ther. 2008;16(11):1833–40. doi:10.1038/mt.2008.200.
- Houseley J, Tollervey D. The many pathways of RNA degradation. Cell. 2009;136:763–76. doi:10.1016/j.cell.2009.01.019.
- Corbett KS, Edwards DK, Leist SR, Abiona OM, Boyoglu-Barnum S, Gillespie RA, Himansu S, Schäfer A, Ziwawo CT, DiPiazza AT. SARS-CoV-2 mRNA vaccine design enabled by prototype pathogen preparedness. Nature. 2020;586:567–71. doi:10.1038/s41586-020-2622-0.
- Pronker ES, Weenen TC, Commandeur H, Claassen EH, Osterhaus AD, Vasilakis N. Risk in vaccine research and development quantified. PloS One. 2013;8(3):e57755. doi:10.1371/journal.pone.0057755.
- Zhao T-X, Wang J-K, Shen L-J, Long C-L, Liu B, Wei Y, Han LD, Wei YX, Wu SD, Wei GH. Increased m6a RNA modification is related to the inhibition of the Nrf2-mediated antioxidant response in di-(2-ethylhexyl) phthalate-induced prepubertal testicular injury. Environ Pollut. 2020;259:259. doi:10.1016/j.envpol.2020.113911.
- Petsch B, Schnee M, Vogel AB, Lange E, Hoffmann B, Voss D, Schlake T, Thess A, Kallen KJ, Stitz L. Protective efficacy of in vitro synthesized, specific mRNA vaccines against influenza A virus infection. Nat Biotechnol. 2012;30:1210–16. doi:10.1038/nbt.2436.
- Verbeke R, Lentacker I, De Smedt SC, Dewitte H. The dawn of mRNA vaccines: the COVID-19 case. J Control Release. 2021;333:511–20. doi:10.1016/j.jconrel.2021.03.043.
- Chaudhary N, Weissman D, Whitehead KA. mRNA vaccines for infectious diseases: principles, delivery and clinical translation. Nat Rev Drug Discov. 2021;20:817–38. doi:10.1038/s41573-021-00283-5.
- Li M, Li Y, Li S, Jia L, Wang H, Li M, Deng J, Zhu A, Ma L, Li W. The nano delivery systems and applications of mRNA. Eur J Med Chem. 2022;227:113910. doi:10.1016/j.ejmech.2021.113910.
- Risma KA, Edwards KM, Hummell DS, Little FF, Norton AE, Stallings A, Wood RA, Milner JD. Potential mechanisms of anaphylaxis to COVID-19 mRNA vaccines. J Allergy Clin Immunol. 2021;147(6):2075–82.e2. doi:10.1016/j.jaci.2021.04.002.
- Polack FP, Thomas SJ, Kitchin N, Absalon J, Gurtman A, Lockhart S, Perez JL, Marc GP, Moreira ED, Zerbini C. Safety and efficacy of the BNT162b2 mRNA Covid-19 vaccine. N Engl J Med. 2020;383:2603–15. doi:10.1056/NEJMoa2034577.
- Baden LR, El Sahly HM, Essink B, Kotloff K, Frey S, Novak R, Diemert D, Spector SA, Rouphael N, Creech CB. Efficacy and safety of the mRNA-1273 SARS-CoV-2 vaccine. N Engl J Med. 2021;384(5):403–16. doi:10.1056/NEJMoa2035389.
- Rota PA, Oberste MS, Monroe SS, Nix WA, Campagnoli R, Icenogle JP, Peñaranda S, Bankamp B, Maher K, Chen MH. Characterization of a novel coronavirus associated with severe acute respiratory syndrome. Science (New York, NY). 2003;300(5624):1394–99. doi:10.1126/science.1085952.
- Li F. Structure, function, and evolution of coronavirus Spike proteins. Ann Rev Virol. 2016;3(1):237–61. doi:10.1146/annurev-virology-110615-042301.
- Wang Q, Zhang Y, Wu L, Niu S, Song C, Zhang Z, Lu G, Qiao C, Hu Y, Yuen KY. Structural and functional basis of SARS-CoV-2 entry by using Human ACE2. Cell. 2020;181(4):894–904.e9. doi:10.1016/j.cell.2020.03.045.
- Li W, Moore MJ, Vasilieva N, Sui J, Wong SK, Berne MA, Somasundaran M, Sullivan JL, Luzuriaga K, Greenough TC. Angiotensin-Converting enzyme 2 is a functional receptor for the SARS coronavirus. Nature. 2003;426:450–54. doi:10.1038/nature02145.
- Letko M, Marzi A, Munster V. Functional assessment of cell entry and receptor usage for SARS-CoV-2 and other lineage B betacoronaviruses. Nat Microbiol. 2020;5:562–69. doi:10.1038/s41564-020-0688-y.
- Ou X, Liu Y, Lei X, Li P, Mi D, Ren L, Guo L, Guo R, Chen T, Hu J. Characterization of spike glycoprotein of SARS-CoV-2 on virus entry and its immune cross-reactivity with SARS-CoV. Nat Commun. 2020;11:1620. doi:10.1038/s41467-020-15562-9.
- Dolgin E. How COVID unlocked the power of RNA vaccines. Nature. 2021;589:189–91. doi:10.1038/d41586-021-00019-w.
- Eberhardt CS, Siegrist CA. Is there a role for childhood vaccination against COVID-19? Pediatric allergy and immunology : official publication of the European Society of Pediatric Allergy and Immunology. Pediatr Allergy Immunol. 2021;32(1):9–16. doi:10.1111/pai.13401.
- Sadegh-Nasseri S, Kim A. Exogenous antigens bind MHC class II first, and are processed by cathepsins later. Mol Immunol. 2015;68:81–84. doi:10.1016/j.molimm.2015.07.018.
- Sahin U, Muik A, Derhovanessian E, Vogler I, Kranz LM, Vormehr M, Baum A, Pascal K, Quandt J, Maurus D, et al. COVID-19 vaccine BNT162b1 elicits human antibody and T(H)1 T cell responses. Nature. 2020;586(7830):594–99. doi:10.1038/s41586-020-2814-7.
- Alberer M, Gnad-Vogt U, Hong HS, Mehr KT, Backert L, Finak G, et al. Safety and immunogenicity of a mRNA rabies vaccine in healthy adults: an open-label, non-randomised, prospective, first-in-human phase 1 clinical trial. Lancet (London England). 2017;390(10101):1511–20. doi:10.1016/s0140-6736(17)31665-3.
- Bahl K, Senn JJ, Yuzhakov O, Bulychev A, Brito LA, Hassett KJ, Gottardo R, Bica MA, Garofano A, Koch SD, et al. Preclinical and clinical demonstration of immunogenicity by mRNA vaccines against H10N8 and H7N9 influenza viruses. Mol Ther. 2017;25:1316–27. doi:10.1016/j.ymthe.2017.03.035.
- Bergwerk M, Gonen T, Lustig Y, Amit S, Lipsitch M, Cohen C, Mandelboim M, Levin EG, Rubin C, Indenbaum V, et al. Covid-19 breakthrough infections in vaccinated health care workers. N Engl J Med. 2021;385(16):1474–84. doi:10.1056/NEJMoa2109072.
- Daniel W, Nivet M, Warner J, Podolsky DK. Early evidence of the effect of SARS-CoV-2 vaccine at one medical center. N Engl J Med. 2021;384:1962–63. doi:10.1056/NEJMc2102153.
- Benenson S, Oster Y, Cohen MJ, Nir-Paz R. Bnt162b2 mRNA Covid-19 vaccine effectiveness among health care workers. N Engl J Med. 2021;384:1775–77. doi:10.1056/NEJMc2101951.
- Thompson MG, Burgess JL, Naleway AL, Tyner HL, Yoon SK, Meece J, Olsho, LE, Caban-Martinez AJ, Fowlkes A, Lutrick K, et al. Interim estimates of vaccine effectiveness of BNT162b2 and mRNA-1273 COVID-19 vaccines in preventing SARS-CoV-2 infection among health care personnel, first responders, and other essential and frontline workers - Eight U.S. locations, December 2020-March 2021. MMWR Morb Mortal Wkly Rep. 2021;70(13):495–500. doi:10.15585/mmwr.mm7013e3.
- Keehner J, Horton LE, Pfeffer MA, Longhurst CA, Schooley RT, Currier JS, Abeles SR, Torriani FJ, et al. SARS-CoV-2 infection after vaccination in health care workers in California. N Engl J Med. 2021;384(18):1774–75. doi:10.1056/NEJMc2101927.
- Leeb RT, Price S, Sliwa S, Kimball A, Szucs L, Caruso E, Godfred-Cato S, Lozier M, et al. COVID-19 trends among school-aged children — United States, March 1–september 19, 2020. MMWR Morb Mortal Wkly Rep. 2020;69(39):1410–15. doi:10.15585/mmwr.mm6939e2.
- Auger KA, Shah SS, Richardson T, Hartley D, Hall M, Warniment A, Timmons K, Bosse D, Ferris SA, Brady PW, et al. Association between statewide school closure and COVID-19 incidence and mortality in the US. Jama. 2020;324:859–70. doi:10.1001/jama.2020.14348.
- Ebinger JE, Fert-Bober J, Printsev I, Wu M, Sun N, Prostko JC, Van Eyk JE, Braun JG, et al. Antibody responses to the BNT162b2 mRNA vaccine in individuals previously infected with SARS-CoV-2. Nat Med. 2021;27:981–84. doi:10.1038/s41591-021-01325-6.
- Thomas SJ, Moreira ED Jr., Kitchin N, Absalon J, Gurtman A, Lockhart S, Perez JL, Pérez Marc G, Polack FP, Zerbini C et al. Safety and efficacy of the BNT162b2 mRNA Covid-19 vaccine through 6 months. N Engl J Med. 2021;385:1761–73. doi:10.1056/NEJMoa2110345.
- Walter EB, Talaat KR, Sabharwal C, Gurtman A, Lockhart S, Paulsen GC, Barnett ED., Muñoz FM, Maldonado Y, Pahud BA, et al. Evaluation of the BNT162b2 Covid-19 vaccine in children 5 to 11 years of age. N Engl J Med. 2021. doi:10.1056/NEJMoa2116298.
- Woodworth KR, Moulia D, Collins JP, Hadler SC, Jones JM, Reddy SC, Chamberland, M, Campos-Outcalt, D, Morgan, RL, Brooks, O, et al. The advisory committee on immunization practicesʻ interim recommendation for use of Pfizer-BioNtech COVID-19 vaccine in children aged 5–11 years — United States, November 2021. MMWR Morb Mortal Wkly Rep. 2021;70(45):1579–83. doi:10.15585/mmwr.mm7045e1.
- Anderson EJ, Rouphael NG, Widge AT, Jackson LA, Roberts PC, Makhene M, Chappell JD, Denison MR, Stevens LJ, Pruijssers AJ, et al. Safety and immunogenicity of SARS-CoV-2 mRNA-1273 vaccine in older adults. N Engl J Med. 2020;383:2427–38. doi:10.1056/NEJMoa2028436.
- Collier DA, Ferreira I, Kotagiri P, Datir RP, Lim EY, Touizer E, Meng B, Abdullahi A, Elmer A, Kingston N, et al. Age-Related immune response heterogeneity to SARS-CoV-2 vaccine BNT162b2. Nature. 2021;596:417–22. doi:10.1038/s41586-021-03739-1.
- Haas EJ, Angulo FJ, McLaughlin JM, Anis E, Singer SR, Khan F, Brooks N, Smaja M, Mircus G, Pan K, et al. Impact and effectiveness of mRNA BNT162b2 vaccine against SARS-CoV-2 infections and COVID-19 cases, hospitalisations, and deaths following a nationwide vaccination campaign in Israel: an observational study using national surveillance data. Lancet (London, England). 2021;397(10287):1819–29. doi:10.1016/s0140-6736(21)00947-8.
- Marc GP, Alvarez-Paggi D, Polack FP. Mounting evidence for immunizing previously infected subjects with a single dose of SARS-CoV-2 vaccine. J Clin Invest. 2021;131(12):131. doi:10.1172/jci150135.
- Dagan N, Barda N, Kepten E, Miron O, Perchik S, Katz MA, Hernán MA, Lipsitch M, Reis B, et al. Bnt162b2 mRNA Covid-19 vaccine in a nationwide mass vaccination setting. N Engl J Med. 2021;384:1412–23. doi:10.1056/NEJMoa2101765.
- Gobbi F, Buonfrate D, Moro L, Rodari P, Piubelli C, Caldrer S, Riccetti S, Sinigaglia A, Barzon L, et al. Antibody response to the BNT162b2 mRNA COVID-19 vaccine in subjects with prior SARS-CoV-2 infection. Viruses. 2021;13(3):13. doi:10.3390/v13030422.
- Anderson M, Stec M, Rewane A, Landay A, Cloherty G, Moy J. SARS-CoV-2 antibody responses in infection-naive or previously infected individuals after 1 and 2 doses of the BNT162b2 vaccine. JAMA Network Open. 2021;4:e2119741. doi:10.1001/jamanetworkopen.2021.19741.
- Garcia-Beltran WF, Lam EC, Astudillo MG, Yang D, Miller TE, Feldman J, Hauser BM, Caradonna TM, Clayton KL, Nitido AD, et al. COVID-19 neutralizing antibodies predict disease severity and survival. medRxiv : the preprint server for health sciences. 2020. doi:10.1101/2020.10.15.20213512.
- Wang C, Li W, Drabek D, Okba NMA, van Haperen R, Osterhaus A, van Kuppeveld FJ, Haagmans BL, Grosveld F, et al. A human monoclonal antibody blocking SARS-CoV-2 infection. Nat Commun. 2020;11:2251. doi:10.1038/s41467-020-16256-y.
- Young-Xu Y, Korves C, Roberts J, Powell EI, Zwain GM, Smith J, Izurieta HS, et al. Coverage and estimated effectiveness of mRNA COVID-19 vaccines among US veterans. JAMA Network Open. 2021;4(10):e2128391. doi:10.1001/jamanetworkopen.2021.28391.
- Butt AA, Omer SB, Yan P, Shaikh OS, Mayr FB. SARS-CoV-2 vaccine effectiveness in a high-risk national population in a real-world setting. Ann Intern Med. 2021;174:1404–08. doi:10.7326/m21-1577.
- Menni C, Klaser K, May A, Polidori L, Capdevila J, Louca P, Sudre CH, Nguyen LH, Drew DA, Merino J, et al. Vaccine side-effects and SARS-CoV-2 infection after vaccination in users of the COVID symptom study app in the UK: a prospective observational study. Lancet Infect Dis. 2021;21:939–49. doi:10.1016/s1473-3099(21)00224-3.
- Flacco ME, Soldato G, Acuti Martellucci C, Carota R, Di Luzio R, Caponetti A, Manzoli L. Interim estimates of COVID-19 vaccine effectiveness in a mass caccination setting: data from an Italian Province. Vaccines. 2021;9(6):628. doi:10.3390/vaccines9060628.
- Haas EJ, McLaughlin JM, Khan F, Angulo FJ, Anis E, Lipsitch M, Singer SR, Mircus G, Brooks N, Smaja M, et al. Infections, hospitalisations, and deaths averted via a nationwide vaccination campaign using the Pfizer-BioNtech BNT162b2 mRNA COVID-19 vaccine in Israel: a retrospective surveillance study. Lancet Infect Dis. 2021. doi:10.1016/s1473-3099(21)00566-1.
- Barda N, Dagan N, Cohen C, Hernán MA, Lipsitch M, Kohane IS, Reis BY, Balicer RD. Effectiveness of a third dose of the BNT162b2 mRNA COVID-19 vaccine for preventing severe outcomes in Israel: an observational study. Lancet (London, England). 2021;398(10316):2093–100. doi:10.1016/s0140-6736(21)02249-2.
- Vasileiou E, Simpson CR, Shi T, Kerr S, Agrawal U, Akbari A, Bedston S, Beggs J, Bradley D, Chuter A, et al. Interim findings from first-dose mass COVID-19 vaccination roll-out and COVID-19 hospital admissions in Scotland: a national prospective cohort study. Lancet (London, England). 2021;397(10285):1646–57. doi:10.1016/s0140-6736(21)00677-2.
- Bajema KL, Dahl RM, Evener SL, Prill MM, Rodriguez-Barradas MC, Marconi VC, Beenhouwer DO, Holodniy M, Lucero-Obusan C, Brown ST, et al. Comparative effectiveness and antibody responses to Moderna and Pfizer-BioNtech COVID-19 vaccines among hospitalized veterans — Five veterans affairs medical centers, United States, February 1–september 30, 2021. MMWR Morb Mortal Wkly Rep. 2021;70(49):1700–05. doi:10.15585/mmwr.mm7049a2.
- Bruxvoort KJ, Sy LS, Qian L, Ackerson BK, Luo Y, Lee GS, Tian Y, Florea A, Takhar HS, Tubert JE, et al. Real-World effectiveness of the mRNA-1273 vaccine against COVID-19: interim results from a prospective observational cohort study. Lancet Reg Health Am. 2021;6:100134. doi:10.1016/j.lana.2021.100134.
- Aleem A, Akbar Samad AB, and Slenker AK. Emerging variants of SARS-CoV-2 and novel therapeutics against coronavirus (COVID-19). StatPearls Copyright © 2021, StatPearls Publishing LLC., 2021. Treasure Island (FL): StatPearls Publishing; 2022 Jan 5.
- Davies NG, Abbott S, Barnard RC, Jarvis CI, Kucharski AJ, Munday JD, Pearson CA, Russell TW, Tully DC, Washburne AD, et al. Estimated transmissibility and impact of SARS-CoV-2 lineage B.1.1.7 in England. Science (New York, NY). 2021;372. doi:10.1126/science.abg3055.
- Abu-Raddad LJ, Chemaitelly H, Butt AA. Effectiveness of the BNT162b2 Covid-19 vaccine against the B.1.1.7 and B.1.351 variants. N Engl J Med. 2021;385:187–89. doi:10.1056/NEJMc2104974.
- Chemaitelly H, Yassine HM, Benslimane FM, Al Khatib HA, Tang P, Hasan MR, Malek JA, Coyle P, Ayoub HH, Al Kanaani Z et al. mRNA-1273 COVID-19 vaccine effectiveness against the B.1.1.7 and B.1.351 variants and severe COVID-19 disease in Qatar. Nat Med. 2021;27(9):1614–21. doi:10.1038/s41591-021-01446-y.
- Faria NR, Mellan TA, Whittaker C, Claro IM, Candido DDS, Mishra S, Crispim MA, Sales FC, Hawryluk I, McCrone JT, et al. Genomics and epidemiology of the P.1 SARS-CoV-2 lineage in Manaus, Brazil. Science (New York, NY). 2021;372:815–21. doi:10.1126/science.abh2644.
- Lopez Bernal J, Andrews N, Gower C, Gallagher E, Simmons R, Thelwall S, Stowe J, Tessier E, Groves N, Dabrera G, et al. Effectiveness of Covid-19 vaccines against the B.1.617.2 (Delta) variant. N Engl J Med. 2021;385:585–94. doi:10.1056/NEJMoa2108891.
- Liu Y, Liu J, Xia H, Zhang X, Fontes-Garfias CR, Swanson KA, Cai H, Sarkar R, Chen W, Cutler M, et al. Neutralizing activity of BNT162b2-elicited serum. N Engl J Med. 2021;384:1466–68. doi:10.1056/NEJMc2102017.
- Wu K, Werner AP, Moliva JI, Koch M, Choi A, Stewart-Jones GBE, Bennett H, Boyoglu-Barnum S, Shi W, Graham BS, et al. mRNA-1273 vaccine induces neutralizing antibodies against spike mutants from global SARS-CoV-2 variants. bioRxiv. 2021. doi:10.1101/2021.01.25.427948.
- Tartof SY, Slezak JM, Fischer H, Hong V, Ackerson BK, Ranasinghe ON, Frankland TB, Ogun OA, Zamparo JM, Gray S, et al. Effectiveness of mRNA BNT162b2 COVID-19 vaccine up to 6 months in a large integrated health system in the USA: a retrospective cohort study. Lancet (London, England). 2021;398(10309):1407–16. doi:10.1016/s0140-6736(21)02183-8.
- Falsey AR, Frenck RW Jr., Walsh EE, Kitchin N, Absalon J, Gurtman A, Lockhart S, Bailey R, Swanson KA, Xu X, et al. SARS-CoV-2 neutralization with BNT162b2 vaccine dose 3. N Engl J Med. 2021;385:1627–29. doi:10.1056/NEJMc2113468.
- Levine-Tiefenbrun M, Yelin I, Katz R, Herzel E, Golan Z, Schreiber L, Wolf T, Nadler V, Ben-Tov A, Kuint J, et al. Initial report of decreased SARS-CoV-2 viral load after inoculation with the BNT162b2 vaccine. Nat Med. 2021;27:790–92. doi:10.1038/s41591-021-01316-7.
- Tomita K, Okada S, Sugihara S, Ikeuchi T, Touge H, Hasegawa J, Yamasaki A. Physical characteristics of injection site pain after COVID-19 mRNA BNT162b2 vaccination. Yonago Acta Med. 2021;64(4):339–44. doi:10.33160/yam.2021.11.003.
- Oxman MN, Levin MJ, Johnson GR, Schmader KE, Straus SE, Gelb LD, Arbeit RD, Simberkoff MS, Gershon AA, Davis LE, et al. A vaccine to prevent herpes zoster and postherpetic neuralgia in older adults. N Engl J Med. 2005;352:2271–84. doi:10.1056/NEJMoa051016.
- Syed YY. Recombinant Zoster vaccine (Shingrix®): a review in Herpes Zoster. Drugs Aging. 2018;35(12):1031–40. doi:10.1007/s40266-018-0603-x.
- Perez-Vilar S, Dores GM, Marquez PL, Ng CS, Cano MV, Rastogi A, Lee L, Su JR, Duffy J. Safety surveillance of meningococcal group B vaccine (Bexsero®), vaccine adverse event reporting system, 2015-2018. Vaccine. 2021;40(2):247–54. doi:10.1016/j.vaccine.2021.11.071.
- Chapin-Bardales J, Gee J, Myers T. Reactogenicity following receipt of mRNA-based COVID-19 vaccines. Jama. 2021;325:2201–02. doi:10.1001/jama.2021.5374.
- Zhu FC, Li YH, Guan XH, Hou LH, Wang WJ, Li JX, Wu SP, Wang BS, Wang Z, Wang L, et al. Safety, tolerability, and immunogenicity of a recombinant adenovirus type-5 vectored COVID-19 vaccine: a dose-escalation, open-label, non-randomised, first-in-human trial. Lancet (London, England). 2020;395(10240):1845–54. doi:10.1016/s0140-6736(20)31208-3.
- Rice SM, Ferree SD, Mesinkovska NA, Kourosh AS. The art of prevention: COVID-19 vaccine preparedness for the dermatologist. Int J Womenʻs Dermatol. 2021;7(2):209–12. doi:10.1016/j.ijwd.2021.01.007.
- CDC. COVID-19 response team and food and drug administration. Allergic reactions including Anaphylaxis after receipt of the first dose of Pfizer-BioNtech COVID-19 vaccine - United States, December 14-23, 2020. MMWR Morb Mortal Wkly Rep. 2021;70:46–51. doi:10.15585/mmwr.mm7002e1.
- Wi YM, Kim SH, Peck KR. Early adverse events between mRNA and adenovirus-vectored COVID-19 vaccines in healthcare workers. Vaccines. 2021;9(8):931. doi:10.3390/vaccines9080931.
- Zahid MN. Unfolding the mild to moderate short-term side effects of four COVID-19 vaccines used in Bahrain: a cross-sectional study. Vaccines. 2021;9(11):1369. doi:10.3390/vaccines9111369.
- Almufty HB, Mohammed SA, Abdullah AM, Merza MA. Potential adverse effects of COVID19 vaccines among Iraqi population; a comparison between the three available vaccines in Iraq; a retrospective cross-sectional study. Diabetes Metab Syndr. 2021;15(5):102207. doi:10.1016/j.dsx.2021.102207.
- Bozkurt B, Kamat I, Hotez PJ. Myocarditis with COVID-19 mRNA vaccines. Circulation. 2021;144:471–84. doi:10.1161/circulationaha.121.056135.
- Banerji A, Wickner PG, Saff R, Stone CA Jr., Robinson LB, Long AA, Wolfson AR, Williams P, Khan DA, Phillips E, et al. mRNA vaccines to prevent COVID-19 disease and reported allergic reactions: current evidence and suggested approach. J Allergy Clin Immunol Pract. 2021;9(4):1423–37. doi:10.1016/j.jaip.2020.12.047.
- Novak N, Tordesillas L, Cabanillas B. Adverse rare events to vaccines for COVID-19: from hypersensitivity reactions to thrombosis and thrombocytopenia. Int Rev Immunol. 2021;1–10. doi:10.1080/08830185.2021.1939696.
- Kounis NG, Koniari I, de Gregorio C, Velissaris D, Petalas K, Brinia A, Assimakopoulos SF, Gogos C, Kouni SN, Kounis GN, et al. Allergic reactions to current available COVID-19 vaccinations: pathophysiology, causality, and therapeutic considerations. Vaccines. 2021;9(3):221. doi:10.3390/vaccines9030221.
- Rosenblum HG, Hadler SC, Moulia D, Shimabukuro TT, Su JR, Tepper NK, et al. Use of COVID-19 vaccines after reports of adverse events among adult recipients of Janssen (Johnson & Johnson) and mRNA COVID-19 vaccines (Pfizer-BioNtech and Moderna): update from the advisory committee on immunization practices — United States, July 2021. MMWR Morb Mortal Wkly Rep. 2021;70(32):1094–99. doi:10.15585/mmwr.mm7032e4.
- Fazlollahi A, Zahmatyar M, Noori M, Nejadghaderi SA, Sullman MJM, Shekarriz-Foumani R, Kolahi AA, Singh K, et al. Cardiac complications following mRNA COVID-19 vaccines: A systematic review of case reports and case series. Rev Med Virol. 2021;e2318. doi:10.1002/rmv.2318.
- Centers for Disease Control and Prevention (CDC). Advisory committee on immunization practices (ACIP). Coronavirus disease 2019 (COVID-19) vaccines. Accessed 2021 December 25. https://www.cdc.gov/vaccines/acip/meetings/slides-2021-06.html
- Caforio ALP. Receipt of mRNA vaccine against Covid-19 and myocarditis. N Engl J Med. 2021;385:2189–90. doi:10.1056/NEJMe2116493.
- Luk A, Clarke B, Dahdah N, Ducharme A, Krahn A, McCrindle B, Mizzi, T, Naus, M, Udell, JA, Virani, S. et al. Myocarditis and pericarditis after COVID-19 mRNA vaccination: practical considerations for care providers. Can J Cardiol. 2021;37:1629–34. doi:10.1016/j.cjca.2021.08.001.
- Patone M, Mei XW, Handunnetthi L, Dixon S, Zaccardi F, Shankar-Hari M, Watkinson P, Khunti K, Harnden A, Coupland CA, et al. Risks of myocarditis, pericarditis, and cardiac arrhythmias associated with COVID-19 vaccination or SARS-CoV-2 infection. Nat Med. 2021. doi:10.1038/s41591-021-01630-0.
- Stuart ASV, Shaw RH, Liu X, Greenland M, Aley PK, Andrews NJ, Cameron JC, Charlton S, Clutterbuck EA, and Collins AM, et al. Immunogenicity, safety, and reactogenicity of heterologous COVID-19 primary vaccination incorporating mRNA, viral-vector, and protein-adjuvant vaccines in the UK (Com-COV2): a single-blind, randomised, phase 2, non-inferiority trial. Lancet (London England). 2021;399(10319):36–49. doi:10.1016/S0140-6736(21)02718-5.
- Li J, Hui A, Zhang X, Yang Y, Tang R, Ye H, Ji R, Lin M, Zhu Z, Türeci Ö, et al. Safety and immunogenicity of the SARS-CoV-2 BNT162b1 mRNA vaccine in younger and older Chinese adults: a randomized, placebo-controlled, double-blind phase 1 study. Nat Med. 2021;27:1062–70. doi:10.1038/s41591-021-01330-9.
- Jackson LA, Anderson EJ, Rouphael NG, Roberts PC, Makhene M, Coler RN, McCullough MP, Chappell JD, Denison MR, Stevens LJ, et al. An mRNA vaccine against SARS-CoV-2 — preliminary report. N Engl J Med. 2020;383(20):1920–31. doi:10.1056/NEJMoa2022483.
- Stafford IA, Parchem JG, Sibai BM. The coronavirus disease 2019 vaccine in pregnancy: risks, benefits, and recommendations. Am J Obstet Gynecol. 2021;224:484–95. doi:10.1016/j.ajog.2021.01.022.
- Bowman CJ, Bouressam M, Campion SN, Cappon GD, Catlin NR, Cutler MW, Diekmann, J., Rohde, C.M., Sellers, R.S, Lindemann, C. et al. Lack of effects on female fertility and prenatal and postnatal offspring development in rats with BNT162b2, a mRNA-based COVID-19 vaccine. Reprod Toxicol. 2021;103:28–35. doi:10.1016/j.reprotox.2021.05.007.
- Shimabukuro TT, Kim SY, Myers TR, Moro PL, Oduyebo T, Panagiotakopoulos L, Marquez PL, Olson CK, Liu R, Chang KT, et al. Preliminary findings of mRNA Covid-19 vaccine safety in pregnant persons. N Engl J Med. 2021;384:2273–82. doi:10.1056/NEJMoa2104983.
- Hause AM, Gee J, Baggs J, Abara WE, Marquez P, Thompson D, Su JR, Licata C, Rosenblum HG, Myers TR, et al. COVID-19 vaccine safety in adolescents aged 12–17 years — United States, December 14, 2020–july 16, 2021. MMWR Morb Mortal Wkly Rep. 2021;70(31):1053–58. doi:10.15585/mmwr.mm7031e1.
- Ali K, Berman G, Zhou H, Deng W, Faughnan V, Coronado-Voges M, et al. Evaluation of mRNA-1273 SARS-CoV-2 vaccine in adolescents. N Engl J Med. 2021;385:2241–51. doi:10.1056/NEJMoa2109522.
- Frenck RW Jr., Klein NP, Kitchin N, Gurtman A, Absalon J, Lockhart S, Perez JL, Walter EB, Senders S, Bailey R, et al. Safety, immunogenicity, and efficacy of the BNT162b2 Covid-19 vaccine in adolescents. N Engl J Med. 2021;385:239–50. doi:10.1056/NEJMoa2107456.
- Siegel DA, Reses HE, Cool AJ, Shapiro CN, Hsu J, Boehmer TK, Cornwell CR, Gray EB, Henley SJ, Lochner K, et al. Trends in COVID-19 cases, emergency department visits, and hospital admissions among children and adolescents aged 0–17 years — United States, August 2020–August 2021. MMWR Morb Mortal Wkly Rep. 2021;70(36):1249–54. doi:10.15585/mmwr.mm7036e1.
- Doria-Rose N, Suthar MS, Makowski M, Oʻ-Connell S, McDermott AB, Flach B, Ledgerwood JE, Mascola JR., Graham BS, Lin BC, et al. Antibody persistence through 6 months after the second dose of mRNA-1273 vaccine for Covid-19. N Engl J Med. 2021;384:2259–61. doi:10.1056/NEJMc2103916.
- Turner JS, Oʻ-Halloran JA, Kalaidina E, Kim W, Schmitz AJ, Zhou JQ, Lei, T., Thapa, M., Chen, R.E., Li T, Margolick JB, Pawelec G, et al. SARS-CoV-2 mRNA vaccines induce persistent human germinal centre responses. Nature. 2021;596:109–13. doi:10.1038/s41586-021-03738-2.
- Chen Y, Klein SL, Garibaldi BT, Li H, Wu C, Osevala NM, et al. Aging in COVID-19: vulnerability, immunity and intervention. Ageing Res Rev. 2021;65:101205. doi:10.1016/j.arr.2020.101205.
- Britton A, Jacobs Slifka KM, Edens C, Nanduri SA, Bart SM, Shang N, Harizaj A, Armstrong J, Xu K, Ehrlich HY, et al. Effectiveness of the Pfizer-BioNtech COVID-19 vaccine among residents of two skilled nursing facilities experiencing COVID-19 outbreaks — Connecticut, December 2020–february 2021. MMWR Morb Mortal Wkly Rep. 2021;70(11):396–401. doi:10.15585/mmwr.mm7011e3.
- Crommelin DJA, Anchordoquy TJ, Volkin DB, Jiskoot W, Mastrobattista E. Addressing the cold reality of mRNA vaccine stability. J Pharm Sci. 2021;110:997–1001. doi:10.1016/j.xphs.2020.12.006.
- Zhang NN, Li XF, Deng YQ, Zhao H, Huang YJ, Yang G, Huang WJ, Gao P, Zhou C, Zhang RR, et al. A thermostable mRNA vaccine against COVID-19. Cell. 2020;182(5):1271–83.e16. doi:10.1016/j.cell.2020.07.024.
- To KKW, Cho WCS. An overview of rational design of mRNA-based therapeutics and vaccines. Expert opinion on drug discovery. Expert Opin Drug Discov. 2021;16(11):1307–17. doi:10.1080/17460441.2021.1935859.
- Wadhwa A, Aljabbari A, Lokras A, Foged C, Thakur A. Opportunities and challenges in the delivery of mRNA-based vaccines. Pharmaceutics. 2020;12(2):12. doi:10.3390/pharmaceutics12020102.
- Kim SC, Sekhon SS, Shin WR, Ahn G, Cho BK, Ahn JY, Kim YH. et al. Modifications of mRNA vaccine structural elements for improving mRNA stability and translation efficiency. Mol Cell Toxicology. 2021;1–8. doi:10.1007/s13273-021-00171-4.
- Presnyak V, Alhusaini N, Chen YH, Martin S, Morris N, Kline N, Olson S, Weinberg D, Baker KE, Graveley BR, et al. Codon optimality is a major determinant of mRNA stability. Cell. 2015;160(6):1111–24. doi:10.1016/j.cell.2015.02.029.
- Stitz L, Vogel A, Schnee M, Voss D, Rauch S, Mutzke T, Ketterer T, Kramps T, et al. A thermostable messenger RNA based vaccine against rabies. PLoS Negl Trop Dis. 2017;11:e0006108. doi:10.1371/journal.pntd.0006108.