ABSTRACT
An inactivated poliovirus vaccine candidate using Sabin strains (sIPV) grown on the PER.C6® cell line was assessed in infants after demonstrated immunogenicity and safety in adults. The study recruited 300 infants who were randomized (1:1:1:1) to receive one of 3 dose levels of sIPV or a conventional IPV based on Salk strains (cIPV). Poliovirus-neutralizing antibodies were measured before the first dose and 28 days after the third dose. Reactogenicity was assessed for 7 days and unsolicited adverse events (AEs) for 28 days after each vaccination. Serious AEs (SAEs) were recorded throughout the study. Solicited AEs were mostly mild to moderate. None of the SAEs reported in the study were judged vaccine related, including one fatal SAE due to aspiration of vomitus that occurred 26 days after the third dose of low-dose sIPV. After 3 sIPV vaccinations and across all dose levels, seroconversion (SC) rates were at least 92% against Sabin poliovirus types and at least 80% against Salk types, with a dose-response in neutralizing antibody geometric mean titers (GMTs) observed across the 3 sIPV groups. Compared to cIPV, the 3 sIPV groups displayed similar or higher SC rates and GMTs against the 3 Sabin types but showed a lower response against Salk types 1 and 2; this was most visible for Salk type 1. While the PER.C6® cell line-based sIPV showed an acceptable safety profile and immunogenicity in infants, lower seroprotection against type 1 warrants optimization of dose level and additional clinical evaluation.
Introduction
Inactivated poliovirus vaccines (IPVs) based on attenuated strains are needed to achieve global polio eradication.Citation1 After the certification of eradication of wild poliovirus type 2 in 2015Citation2 and type 3 in October 2019,Citation3 the next steps in the fulfillment of the polio eradication goal reside in the cessation of the circulation of wild poliovirus type 1 (WPV1) and of vaccine-derived polioviruses (VDPVs), stemming from decades-long use of attenuated oral polio vaccines (OPVs), which can, rarely, result in vaccine-associated paralytic poliomyelitis (VAPP). In 2020, there were 139 confirmed cases of acute flaccid paralysis (AFP) due to WPV1 reported in 2 countries, Afghanistan and Pakistan, and 116 reported AFP cases due to circulating VDPV (cVDPV); 34 due to cVDPV1 and 86 to cVDPV2.Citation4
The threat posed by both WPV and cVDPVCitation5 needs to be alleviated and will necessitate the eventual ending of vaccination with attenuated OPVs.Citation1 The withdrawal of OPV began in 2016 with the removal of the type 2 component from OPV immunization programs through a switch from trivalent OPV to bivalent OPV (bOPV).Citation2 At the same time, the administration of at least 1 dose of trivalent IPV was advocated to ensure immunity against type 2.Citation2 This was followed by the recent recommendation of the inclusion of a second IPV dose in countries that currently administer 1 IPV dose and bOPV in their routine immunization schedules.Citation6 Currently, the cost-of-goods to produce conventional inactivated poliovirus vaccines based on Salk strains cIPVs is high.Citation7 For global polio eradication to be successful, a greater number of affordable doses of immunogenic, safe IPV, both from clinical and manufacturing perspectives, is needed. Furthermore, since manufacturing of cIPV presents biosecurity risks for manufacturers as the process requires the propagation of pathogenic viral strains, the use of attenuated strains, such as the Sabin strains, which are inherently less risky from a biosecurity perspective, would ideally support the ultimate global transition to IPV. IPV based on Sabin strains (sIPVs) is now available and in routine use in Japan and China.Citation8,Citation9
The sIPV evaluated in this study is manufactured on the PER.C6® cell line, which has demonstrated high manufacturing yields,Citation10–12 allowing for increased manufacturing capacity and more affordable routine IPV immunization in the pre- and post-eradication era. PER.C6® cells are derived from primary human retina cells that were immortalized upon transfection with the E1 region of adenovirus type 5.Citation13 The PER.C6® cell line is suitable for the propagation of many viruses and has been used to produce viral vaccines, such as influenza and West Nile vaccines,Citation10 vaccines based on the adenovirus 26 vector such as those developed against Ebola virus diseaseCitation14 and COVID-19,Citation15 as well as other vaccine candidates.Citation16
The sIPV candidate had an acceptable safety profile and was able to stimulate potent increases in antibody titers in adults previously immunized with OPV,Citation17 supporting the assessment in infants reported here.
A number of sIPVs have been evaluated in infantsCitation18–27 or adults.Citation28 These were well tolerated, although sometimes associated with a higher reactogenicity than cIPV.Citation19,Citation24,Citation25 However, although all were immunogenic in infants, some of these vaccine candidates failed to induce satisfactory seroconversion rates against wild strains.Citation26 Notably, the immune response to sIPV often appears to be blunted by the presence of maternal antibodies against poliovirus, possibly to a larger extent than the response to cIPV.Citation25,Citation26
We describe here the results of a phase 2 dose-escalation study in infants with the sIPV produced in the PER.C6® cell line, a potentially more affordable method of vaccine production.Citation11 The primary objective of the study was to assess the safety and reactogenicity of 3 different dose levels of sIPV in healthy infants. The immunogenicity of the vaccine was assessed 28 days after the third vaccination as a secondary objective. The immune response to expanded program on immunization (EPI) vaccines Diphtheria, Tetanus, whole-cell Pertussis, Hepatitis B and Haemophilus influenzae type b, (DTwP-HBV-Hib) vaccine and 13-valent Pneumococcal Conjugate Vaccine (PCV13), administered concomitantly with IPV, was also assessed as an exploratory objective.
Methods
Design and objectives
The study was conducted in a randomized, controlled, double-blind fashion at 4 study centers in the Philippines (located at the Philippine General Hospital, Manila; the De La Salle Medical and Health Sciences Institute, Dasmariñas, Cavite) between 6 August 2018 and 17 October 2019 (ClinicalTrials.gov Identifier: NCT03566940).
Clinical procedures were approved by the ethical committees of the study centers and complied with Good Clinical Practice principles, in accordance with the Declaration of Helsinki.Citation29,Citation30
Parents or legal guardians of infant participants provided written informed consent before infants’ participation in the study and before any study procedure was performed.
Vaccines
The investigational vaccine was a trivalent IPV based on Sabin strains (sIPV) produced on PER.C6® cells.Citation11 Three vaccine formulations with differing target antigen contents of the 3 poliovirus types were produced: a low-dose (LD) formulation with 2.5 D-antigen units [DU] of type 1, 5 DU of type 2, and 12.5 DU of type 3; an intermediate-dose (ID) formulation with 5 DU of type 1, 10 DU of type 2, and 25 DU of type 3; and a high-dose (HD) formulation with 10 DU of type 1, 20 DU of type 2, and 50 DU of type 3. The selection of vaccine doses was based on a dose-finding study in cynomolgus monkeys.Citation12 Selection of vaccine doses was also based on the safety and immunogenicity of a higher dose vaccine administered in adults in a phase 1 clinical trial.Citation17 The D-antigen content of the sIPV formulations was determined using the cIPV standard reference,Citation31 as the sIPV international standard was not yet in use when trial enrollment began. The sIPV international standard was established by the World Health Organization (WHO) in November 2018.Citation32
A cIPV (Imovax Polio™, Sanofi Pasteur MSD) was used as an active control. This vaccine is based on Salk poliovirus strains produced on Vero cells and contains 40 DU of type 1 [Mahoney], 8 DU of type 2 [MEF-1], and 32 DU of type 3 [Saukett].
Study participants and vaccination
The study planned to recruit 300 healthy infants to be randomly assigned to 4 groups of 75 participants to receive either sIPV LD, sIPV ID, sIPV HD or cIPV. The vaccination schedule consisted of 3 intramuscular injections of sIPV or cIPV at approximately 6, 10 and 14 weeks of age. The study vaccines were co-administered with 3 doses of the DTwP-HBV-Hib and PCV13 vaccines in the opposite thigh at the 3 vaccination visits, and with 2 doses of rotavirus vaccine administered orally at 6 and 14 weeks of age. All participants received booster vaccination with cIPV approximately 24 weeks after the third IPV dose, in line with the WHO polio vaccine recommendations.Citation2 The protocol planned for the administration of an additional IPV dose in children who were not seroprotected within at least 28 days following booster vaccination; if an infant’s neutralizing antibody titer against at least 1 polio strain was <8, the infant was recommended to return to the site for a second booster dose of cIPV. IPV vaccines were administered intramuscularly in the anterolateral thigh.
For inclusion in the study, infants were to be born after a normal term pregnancy (≥37 weeks) with a birth weight of ≥2.5 kg and to be healthy, as confirmed by the investigator on the basis of physical examination, vital signs and medical history, including the course of the pregnancy and relevant medical history of the mother. The main exclusion criteria for study participation were a weight below the tenth percentile for weight and height according to the WHO growth chart at the time of recruitment; allergy; administration of immunosuppressants; autoimmune disease or impairment of the immune function; neurologic disease including seizures; congenital defects or genetic disorders; previously received polio vaccine or previously infected with poliovirus; travel to any country still encountering polio disease; significant acute or chronic illness and fever at the time of vaccination (see protocol in Supplemental Material).
The first part of the study progressed through dose-escalation in 3 sequentially recruited sets of 12 participants each, receiving, respectively, the LD, ID, and HD level of sIPV or cIPV in a 3:1 ratio. Recruitment of infants to receive a higher dose level of sIPV was conditional on an absence of safety concern through blinded review of the 7-day safety data for the previous set of 12 participants receiving the immediately lower dose level. The full study cohort was recruited after authorization by an independent data monitoring committee (IDMC) based on an unblinded 7-day safety data review for the complete set of the 36 initially enrolled participants. A second review of the safety data by the IDMC was performed once 25% of all participants (n = 75) had received their second sIPV or cIPV vaccination. A third review of safety data by the IDMC was conducted when all participants had received their second IPV vaccination and safety had been documented through encoded diary cards.
Safety analyses
After each vaccination, infants were observed for 30 minutes at the study site. Parents/guardians received diary cards for daily recording of body temperature and occurrences of solicited local and systemic adverse events (AEs) in the 7 days after each vaccination. Unsolicited AEs were recorded from the day of first vaccination until 28 days after each study vaccination and serious AEs (SAEs) throughout the study.
Immunogenicity analyses
Blood samples were collected before the first primary vaccination and 28 days after the third IPV dose. An additional blood sample was collected 28 days after cIPV booster vaccination to verify serostatus in infants who had a poliovirus-neutralizing antibody titer <8 after the third primary dose.
Polio
Neutralizing antibody titers against the wild-type Salk poliovirus strains (type 1 [Mahoney], type 2 [MEF-1] and type 3 [Saukett]), as well as against the Sabin poliovirus strains (types 1, 2 and 3), were measured in accordance with the WHO recommendations for immunogenicity assessment of IPV.Citation31 The assays were performed at the Centers for Disease Control and Prevention (CDC, USA). Neutralizing antibodies against Salk or Sabin polioviruses were measured as previously described,Citation17 using the assay range till 10.5 Log2.
Routine infant vaccines
The immune response to diphtheria, tetanus, pertussis, hepatitis B and pneumococcal antigens was assessed through the determination of concentrations of binding antibodies against their respective antigens by (multiplex) enzyme-linked immunosorbent assay (ELISA). Assays for diphtheria, tetanus, pertussis and hepatitis B antibodies were performed at PPD (Morrisville, NC, USA) and antibodies against the pneumococcal antigens were determined at Q2 (Morrisville, NC, USA). The results of the assessment of the immune response to the concomitant Haemophilus influenzae type b vaccine administration, performed at Public Health England (London, UK), were not available at the time of writing.
Statistical analyses
The primary population for the safety and immunogenicity analyses consisted of all vaccinated participants with safety data available for at least 1 IPV vaccine administration (safety analysis set) and of all vaccinated participants with post–dose 3 immunogenicity data available, excluding participants with major protocol deviations expected to impact the immunogenicity outcomes (per protocol immunogenicity set).
Demographic characteristics were provided for age, sex, race, weight, height and body mass index.
Safety data were analyzed descriptively. The verbatim terms used to identify unsolicited adverse events were coded using the Medical Dictionary for Regulatory Activities (MedDRA version 21). All solicited and unsolicited AEs with onset within 7 days or 28 days after vaccination, respectively, were included in the analysis. For each AE, the percentage of participants who experienced at least 1 occurrence of the event was calculated per vaccine group.
Descriptive statistics were used for immunogenicity endpoints (geometric mean titers [GMTs] and proportions for seroprotection and seroconversion with unadjusted 95% confidence intervals [CIs]), as no formal hypotheses were tested. The proportion of participants with antibody titers against poliovirus ≥8, the seroprotective threshold in the Salk neutralizing assay, and GMT with 95% confidence intervals were calculated per treatment group for each poliovirus type against the wild type and the Sabin strains. Means with 95% CIs of the Log2 transformed values were back-transformed to estimate GMTs with 95% CIs. Seroconversion was defined, for participants with pre-vaccination antibody titer <8, as having a post-vaccination titer ≥8 and, for participants with a pre-vaccination antibody titer ≥8, as having a ≥ 4-fold increase in poliovirus antibody titer post-vaccination. Correction for maternal antibody decline with a half-life of 28 days was applied.Citation31
Results
Three hundred infants were randomized to the 4 study groups and received at least 1 dose of study vaccine: 75 participants in the sIPV LD group, 74 in the sIPV ID group, 75 in the sIPV HD group, and 76 in the cIPV group. The disposition of participants in study groups and analyses is presented in .
Figure 1. Disposition of participants. Notes: IPV = inactivated polio vaccine; sIPV = Sabin-IPV; LD = low dose; ID = intermediate dose; HD = high dose; cIPV = conventional Salk-IPV.
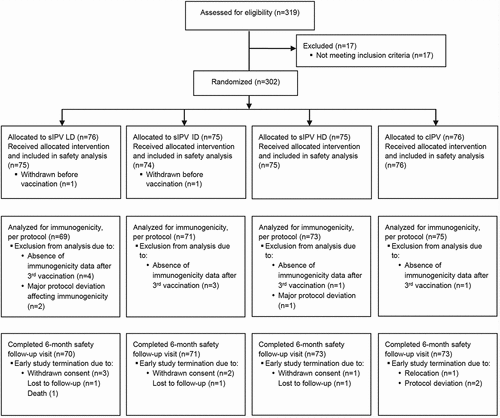
Demographics and baseline seropositivity against poliovirus
Baseline characteristics of participants were similar in the 4 study groups (). Overall, 59.7% of participants were male. Neutralizing antibodies against poliovirus were present on the day of first vaccination at 6 weeks of age in up to 37% of infants when measured against Salk strains and in up to 58% when measured against the Sabin strains.
Table 1. Baseline characteristics of participants in the per protocol immunogenicity set
Safety results
Solicited local AEs were mostly Grade 1 or 2 (). Grade 3 pain was reported in 6.7% to 10.4% of the infants over the vaccination course. An occurrence of Grade 4 pain (defined as emergency room visit or hospitalization) was reported in an infant in the sIPV LD group on the day of first vaccination and was considered resolved after a duration of 3 days. This grade 4 pain was not considered an SAE. Pain/tenderness was the most frequently reported solicited local AE at the IPV injection site, reported for 80% or more of study participants. No increase in the frequency of local AEs was observed with increasing sIPV vaccine dose level, and frequencies were similar in the sIPV and cIPV groups.
Table 2. Solicited and unsolicited adverse events [n (%)] after 3-dose primary vaccination
Solicited systemic AEs were mostly grade 1 or 2 (). The most frequent solicited systemic AEs in the 4 groups were irritability, increased or decreased sleep and reduced activity (). Fever was reported for 32.4% to 45.3% of participants over the sIPV vaccination course compared to 36.4% after cIPV vaccination (). No increase in frequency of fever or other systemic AEs was observed with increasing sIPV dose levels, and frequencies were similar in the sIPV and cIPV groups. Other vaccines (DTwP-HBV-Hib, PCV13 and rotavirus vaccine) were administered at the same time as IPV and the contribution of individual vaccines to systemic reactogenicity cannot be determined. A trend of reduced reactogenicity was observed with subsequent dosing, as shown for fever in , as well as across all types of events (data not shown).
Figure 2. Incidence of fever after 3-dose primary vaccination. The incidence of fever in categories as indicated in green, yellow, red, black and gray, after the 1st, 2nd and 3rd immunization as well as the cumulative incidence, is shown for the different dose levels of sIPV and for cIPV. IPV = inactivated poliovirus vaccine; sIPV = Sabin-IPV; LD = low dose; ID = intermediate dose; HD = high dose; cIPV = conventional Salk-IPV.
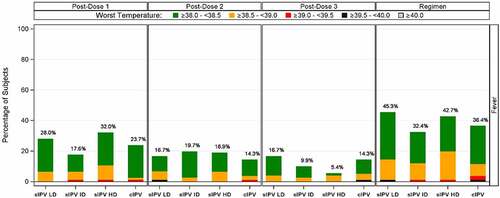
The most frequent unsolicited AEs (reported in ≥10% of participants in any study group) were upper respiratory tract infections (URTI), rhinitis and nasopharyngitis: frequencies were similar in the sIPV and cIPV groups (). One unsolicited AE was considered related to study vaccination: this was a case of grade 2 urticaria reported for 1 participant in the sIPV LD group after the first vaccination. This AE of urticaria was reported 1 day after the participant had received the first dose of sIPV LD and co-administered vaccines (DTwP-HBV-Hib, PCV13, and rotavirus vaccines). The event resolved after a duration of 6 days. The participant continued in the study and received unblinded, commercially available cIPV for the second and third vaccination.
Eight SAEs (including 1 fatal SAE) were reported in 6 participants during the vaccination phase (up to 28 days post-vaccination): these were pneumonia (1 participant in the sIPV HD group and 1 in the cIPV group), gastroenteritis (2 participants in the cIPV group), subcutaneous abscess (1 participant in the sIPV ID group), urinary tract infection (1 participant in the cIPV group), febrile convulsion (1 participant in the sIPV HD group) and aspiration of vomitus with fatal outcome reported in 1 participant in the sIPV LD group 26 days after the third vaccination. None of the SAEs were considered related to study vaccination. None of the SAEs reported in the period beyond 28 days post-vaccination (pneumonia, gastroenteritis, gastroenteritis bacterial, measles, pneumonia measles, URTI bacterial, skin abrasion, urinary tract infection, amoebic dysentery, febrile convulsion and seizure) were considered related to study vaccination.
Immunogenicity results
The primary IPV vaccination course of 3 doses at 6, 10 and 14 weeks of age led to seroconversion in more than 90% of infants in terms of neutralizing antibodies against the 3 Sabin strains in all study groups: 93% to 100% in the sIPV groups and 91% to 100% in the cIPV group (). Seroprotection rates against the Sabin strains were also similar (99% to 100%) in all study groups (). GMTs of antibodies against the 3 Sabin strains were higher in the 3 sIPV groups than in the cIPV group except for a lower GMT against Sabin type 3 in the sIPV LD group compared to cIPV (). See also Table S1 for data on seroconversion, seroprotection and GMTs. A dose-dependent pattern in post-vaccination antibody titers against Sabin strains was observed across the sIPV groups.
Figure 3. Anti-poliovirus seroconversion rates (with 95% CI) 28 days after dose 3 – per protocol immunogenicity set. Seroconversion rates at 28 days after the 3rd immunization for Salk and Sabin virus types 1, 2 and 3 are plotted for each group. Seroconversion was determined as described in the Methods. IPV = inactivated poliovirus vaccine; sIPV = Sabin-IPV; LD = low dose; ID = intermediate dose; HD = high dose; cIPV = conventional Salk-IPV; CI = confidence interval.
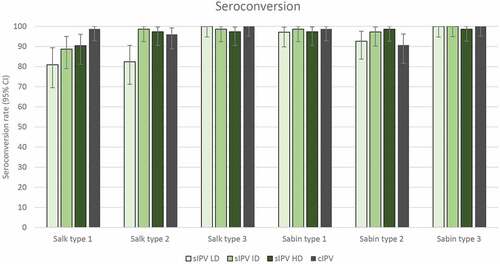
Figure 4. Anti-poliovirus seroprotection rates (with 95% CI) 28 days post-dose 3 – per protocol immunogenicity set. Seroprotection rates at 28 days after the 3rd immunization for Salk and Sabin virus types 1, 2 and 3 are plotted for each group. Seroprotection was determined as described in the Methods. IPV = inactivated poliovirus vaccine; sIPV = Sabin-IPV; LD = low dose; ID = intermediate dose; HD = high dose; cIPV = conventional Salk-IPV; CI = confidence interval.
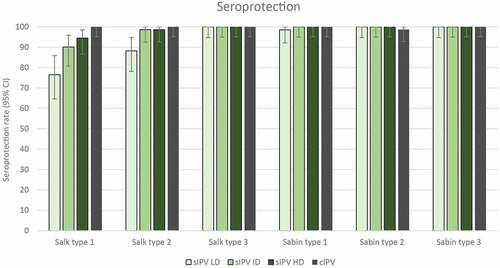
Figure 5. Anti-poliovirus geometric mean titers (with 95% CI) 28 days after dose 3 – per protocol immunogenicity set. Neutralizing antibody titers at 28 days after the 3rd immunization for Salk and Sabin virus types 1, 2 and 3 are plotted as geometric mean titers for each group. Neutralizing antibody titers were determined as described in the Methods. IPV = inactivated poliovirus vaccine; GMT = geometric mean titer; sIPV = Sabin-IPV; LD = low dose; ID = intermediate dose; HD = high dose; cIPV = conventional Salk-IPV; CI = confidence interval.
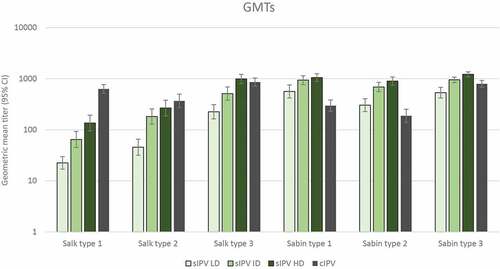
Regarding neutralizing antibodies against the Salk strains, seroconversion rates were similar in the 4 study groups (97% to 100%) for type 3, but differences were observed for types 1 and 2 (). For Salk type 1, seroconversion rates were noticeably lower in all sIPV groups (81% to 90%) compared to cIPV (99%). Regarding Salk type 2, sIPV ID and HD led to similar seroconversion rates (99% and 97%, respectively) as cIPV (96%), whereas sIPV LD led to 82% seroconversion.
The post-vaccination seroprotection rates to Salk type 1 ranged from 77% after sIPV LD to 95% after sIPV HD, compared to 100% after cIPV vaccination (). Seroprotection rates against Salk types 2 and 3 were similar (at least 99%) after sIPV and cIPV vaccination, except for a lower rate (88%) after sIPV LD for type 2. A clear dose-response relationship was observed across the sIPV groups for both seroconversion and seroprotection against Salk type 1. Post-vaccination GMTs against Salk type 1 were lower in all sIPV groups (range, 22 to 136; mean fold increase from baseline: LD, 3.1; ID, 8.9; HD, 16.7) compared to the cIPV group (mean, 630; mean fold increase from baseline: 84.2; Table S1), whereas GMTs to Salk types 2 and 3 were lower after sIPV LD and ID and similar after sIPV HD compared to cIPV (; Table S1). A dose-relationship effect was visible on neutralizing antibody GMTs against the 3 poliovirus types in the 2 assays, as titers increased with increasing sIPV dose levels. All infants who had titers <8 after 3-dose IPV primary vaccination had titers ≥8 after the cIPV booster dose administered 6 months later.
A high proportion of infants had preexisting poliovirus-neutralizing antibodies of maternal origin. No polio vaccine had been received before vaccination in the study. Post-vaccination GMTs are presented based on neutralizing antibody serostatus at baseline in .
Figure 6. Geometric mean poliovirus-neutralizing antibody titers (with 95% CI) 28 days post-dose 3 according to pre-vaccination serostatus–per protocol immunogenicity set. Neutralizing antibody titers at 28 days after the 3rd immunization for Salk and Sabin virus types 1, 2 and 3 are plotted as geometric mean titers for each group for participants that were seronegative at baseline (open circles) or seropositive at baseline (full circles). Neutralizing antibody titers were determined as described in the Methods. IPV = inactivated poliovirus vaccine; sIPV = Sabin-IPV; LD = low dose; ID = intermediate dose; HD = high dose; cIPV = conventional Salk-IPV; CI = confidence interval; ULOQ, upper limit of quantification; SP = seroprotection threshold.
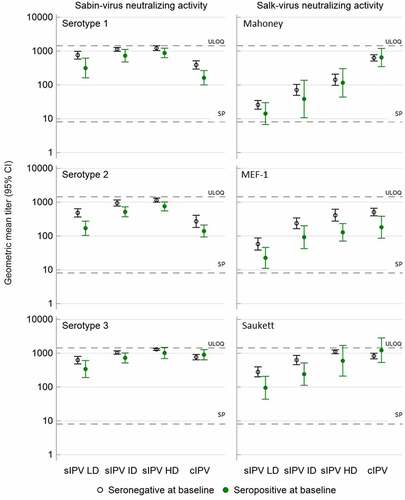
There was evidence of a blunting effect of preexisting antibodies on the post-vaccination GMTs in all sIPV groups against the 3 Salk and Sabin types: titers were lower in infants with preexisting antibodies than in initially seronegative infants (titer <8). This blunting effect was less pronounced with sIPV HD and was not observed with cIPV for Salk type 1 and Salk and Sabin type 3.
The immune response to the concomitantly administered DTwP, HBV, and PCV13 vaccines showed no differences between the sIPV and cIPV groups (Tables S2 and S3).
Discussion
This dose-finding study in infants was initiated after the safety and immunogenicity of an initial formulation of the candidate vaccine with higher antigen content had been confirmed in adults.Citation17 A single dose of this vaccine was able to boost neutralizing antibody titers against both Salk and Sabin strains in adults previously vaccinated with OPV.
The 3 escalating sIPV dose levels were well tolerated in infants and no increase in reactogenicity was observed with increasing antigen content, nor relatively to the control group receiving licensed cIPV. This is in contrast with some other studies that found their high sIPV dose level, and sometimes also the ID level, associated with higher frequencies of AEs such as swelling,Citation23 local AEs in generalCitation25 and more specifically fever.Citation18,Citation19,Citation22–24 Authors have attributed this effect to the higher D-antigen content of the sIPV formulation. Since IPV was co-administered with routine DTwP-HBV-Hib, PCV13 and rotavirus vaccines in our study, it is still possible that a difference in systemic reactogenicity across sIPV groups may have been masked by reactions to these other vaccines.
Seroconversion and seroprotection rates against the 3 Sabin strains were high after vaccination with the 3 sIPV dose levels, and GMTs of neutralizing antibodies against those strains were either similar to or higher than after cIPV vaccination. With regard to the wild poliovirus strains, seroconversion and seroprotection rates were similar in sIPV groups and in the cIPV control group for type 3; they were similar after sIPV ID and HD and cIPV for type 2 and were noticeably lower in all sIPV groups compared to cIPV for type 1. GMTs of antibodies against the 3 wild type strains showed a dose-dependent pattern in the sIPV groups. Compared to cIPV, GMTs to wild type 1 were lower in all sIPV groups, while they were similar after sIPV HD for wild types 2 and 3. Additional D-antigen ELISA and Biacore analyses in which Janssen sIPV was compared to cIPV, the new international standard sIPV and Intravacc (Bilthoven, The Netherlands) sIPV batches (data not shown) did not yield an immediate explanation for the observed lower Salk type 1 responses. The obtained antigen content values were in a similar range as measured for a batch of sIPV produced by Intravacc. This sIPV has recently been shown to induce both non-inferior seroprotection and seroconversion compared to cIPV in a 6, 10, 14-week vaccination regimen.Citation27 It should be noted, however, that GMT levels against Salk type 1 (Mahoney) were substantially lower after sIPV vaccination than after cIPV vaccination, showing a reduction in GMT (89.9 vs 665.43) similar in magnitude to that observed in our study for sIPV HD (135.52 vs. 630.29). Differences in GMTs versus cIPV were less pronounced for types 2 and 3 as in our study, and seroprotection rates against the 3 wild types ranged from 98.4% to 100% post-vaccination compared to 94.5% to 100% in the present study. Further analyses with a broader panel of monoclonal antibodies against type 1 epitopes are being planned. A continued development of this sIPV for infant immunization would warrant an adjustment of the formulation through an increase in the target antigen content for Sabin type 1. The safety profile and immunogenicity of new formulations would need to be assessed in clinical trials.
All sIPVs previously assessed in infants have shown a similar level of immunogenicity to cIPV against the Sabin strains. When neutralizing antibodies to the wild type strains were measured, mean post-vaccination titers were similar to those elicited by cIPV for some of these vaccinesCitation20–22 and lower for type 1 for 2 vaccines.Citation26,Citation27 In the former studies, infants received the first vaccine dose between 2 and 3 months of ageCitation21 or from 3 months up to 67 monthsCitation22 and 89 months of age,Citation20 while in the latter studies,Citation26,Citation27 infants were vaccinated at 6, 10, and 14 weeks of age like in our study. At this earlier age, maternal antibodies are present at higher titers in the serum of infants and are likely to exert a more pronounced blunting effect than later in infancy.
The interference of maternal antibodies on the response to polio vaccination of infants is documented.Citation2,Citation25,Citation33 An inhibitory effect of maternal antibodies on immune responses of infants to similar vaccine antigens has been shown for a number of vaccines and was found most pronounced for IPV; the effect was quantified as a 20% to 28% decrease in post-IPV antibody levels for every 2-fold increase in maternal antibody titer.Citation34 Of note, in a recent clinical study in the Philippines in 6-week-old infants, the high seroprotection rate against poliovirus measured before vaccination was not an obstacle to seroconversion in the majority of infants following vaccination with Salk-IPV adjuvanted on aluminum.Citation35
In our study, some influence of maternal antibodies on the immune response to sIPV was apparent for all the vaccine dose levels, although the effect was smaller in the group receiving the high vaccine dose level. Evidence of such an effect was also observed in the sIPV study conducted in 6-week-old infants in Panama.Citation26 In our study, this effect, overall moderate, is unlikely to account on its own for the observed lower responses, but suggests that higher dosages may be able to counteract the inhibitory effect of preexisting maternal antibodies against certain Salk and Sabin types. Administering a higher dose of Sabin type 1 to potentially increase GMTs and attenuate the impact of preexisting maternal antibodies could be further explored in future studies. The immune response to other recommended infant vaccination did not appear to be affected by the simultaneous administration of sIPV.
Limitations to this study include conduct in a single country (the Philippines), meaning geographical impact on the clinical profile of the vaccine could not be assessed. Furthermore, our study assessed only primary vaccination and was limited by lack of data from longer follow-up after the booster vaccination. Moreover, co-administration of the sIPV vaccine with other vaccines precluded detection of any subtle differences among safety profiles at different dose levels. Therefore, we cannot definitively establish a relationship to co-administered vaccines versus the investigational product of the reported solicited systemic AEs. However, given that the co-administration of other routine infant vaccines was consistent across treatment arms, it is likely that the difference between safety profiles was driven by the investigational product.
In conclusion, the sIPV vaccine evaluated in infants in this study showed a classical reactogenicity profile in co-administration with routine infant vaccines. With regard to wild poliovirus, the high antigen dose level led to a lower level of seroconversion to type 1 in 6-week-old infants, and adequate seroconversion against wild types 2 and 3. The vaccine showed however potential for adequate immunogenicity in this population through dose-level adaptation.
Data sharing statement
The data sharing policy of Janssen Pharmaceutical Companies of Johnson & Johnson is available at https://www.janssen.com/clinical-trials/transparency. As noted on this site, requests for access to the study data can be submitted through Yale Open Data Access (YODA) Project site at http://yoda.yale.edu.
Supplemental Material
Download MS Word (30.5 KB)Acknowledgments
The authors would like to thank the parents who agreed to have their child participate in this study. Thanks also go to all study personnel at study sites, to the CDC for performing the poliovirus neutralization assays and the Janssen study personnel. We also acknowledge Carlo Van Wordragen and the Janssen manufacturing group for their efforts in developing the Sabin IPV vaccine. Dr Salvacion Gatchalian of the Manila Doctors Hospital, Manila, Philippines, was the principal investigator of this clinical study. She passed away on 26 March 2020 before the finalization of this publication, and we are grateful for her contributions.
Disclosure statement
G.Sh., A.G., M.Du., G.Sc., V.v.P., M.L.G., H.S. and M.Do. are full-time employees of the study sponsor. C.P.C. was a full-time employee of the sponsor at the time of the trial, J.-M. J. is an independent consultant under contract with the study sponsor. A.L.O.-L, M.T.-A., and D.C.-Y. have received grants from Janssen Vaccines to finance the conduct of this study in their institution. G.Sh, G.Sc, M.L.G., H.S., and A.G hold shares in Johnson & Johnson.
Supplementary material
Supplemental data for this article can be accessed on the publisher’s website at https://doi.org/10.1080/21645515.2022.2044255.
Additional information
Funding
References
- Global Polio Eradication Initiative, World Health Organization. Polio endgame strategy 2019-2023. Eradication, integration, certification and containment. [accessed 2021 June 30]. http://polioeradication.org/wp-content/uploads/2019/06/english-polio-endgame-strategy.pdf .
- World Health Organization. Polio vaccines: WHO position paper – March, 2016. Wkly Epidemiol Rec. 2016;91(12):145–11.
- Chard AN, Datta SD, Tallis G, Burns CC, Wassilak SGF, Vertefeuille JF, Zaffran M. Progress toward polio eradication — worldwide, January 2018–March 2020. MMWR Morb Mortal Wkly Rep. 2020;69(25):784–89. doi:10.15585/mmwr.mm6925a4.
- Global Polio Eradication Initiative. GPEI polio today WPV reports. [accessed 2021 June 30]. https://polioeradication.org/polio-today/polio-now/this-week/ .
- Jorba J, Diop OM, Iber J, Henderson E, Zhao K, Quddus A, Sutter R, Vertefeuille JF, Wenger J, Wassilak SGF, et al. Update on vaccine-derived poliovirus outbreaks — worldwide, January 2018–June 2019. MMWR Morb Mortal Wkly Rep. 2019;68(45):1024–28. doi:10.15585/mmwr.mm6845a4.
- World Health Organization Strategic Advisory Group of Experts. Meeting of the strategic advisory group of experts on immunization, October 2020 – conclusions and recommendations. no. 48. Wkly Epidemiol Rep 2020;95:585–608.
- Okayasu H, Sutter RW, Jafari HS, Takane M, Aylward RB. Affordable inactivated poliovirus vaccine: strategies and progress. J Infect Dis. 2014;210(suppl_1):S459–64. doi:10.1093/infdis/jiu128.
- Shimizu H. Development and introduction of inactivated poliovirus vaccines derived from Sabin strains in Japan. Vaccine. 2016;34:1975–85. doi:10.1016/j.vaccine.2014.11.015.
- Yan S, Chen H, Zhang Z, Chang S, Xiao Y, Luo L, Zhang Z, Sun L, Chen X, Yang Y, et al. Immunogenicity and safety of different sequential schedules of Sabin strain-based inactivated poliovirus vaccination: A randomized, controlled, open-label, phase IV clinical trial in China. Vaccine. 2020;38:6274–79. doi:10.1016/j.vaccine.2020.07.042.
- Sanders BP, Edo-Matas D, Custers JH, Koldijk MH, Klaren V, Turk M, Luitjens A, Bakker WA, Uytdehaag F, Goudsmit J, et al. Per.C6® cells as a serum-free suspension cell platform for the production of high titer poliovirus: A potential low cost of goods option for world supply of inactivated poliovirus vaccine. Vaccine. 2013;31(5):850–56. doi:10.1016/j.vaccine.2012.10.070.
- Sanders BP, Oakes Ide L, van Hoek V, Liu Y, Marissen W, Minor PD, Wimmer E, Schuitemaker H, Custers JH, Macadam A, et al. Production of high titer attenuated poliovirus strains on the serum-free PER.C6® cell culture platform for the generation of safe and affordable next generation IPV. Vaccine. 2015;33(48):6611–16. doi:10.1016/j.vaccine.2015.10.091.
- Bockstal V, Tiemessen MM, Achterberg R, Van Wordragen C, Knaapen AM, Serroyen J, Marissen WE, Schuitemaker H, Zahn R. An inactivated poliovirus vaccine using Sabin strains produced on the serum-free PER.C6® cell culture platform is immunogenic and safe in a non-human primate model. Vaccine. 2018;36(46):6979–87. doi:10.1016/j.vaccine.2018.09.068.
- Fallaux FJ, Bout A, van der Velde I, van den Wollenberg DJ, Hehir KM, Keegan J, Auger C, Cramer SJ, van Ormondt H, van der Eb AJ, et al. New helper cells and matched early region 1-deleted adenovirus vectors prevent generation of replication-competent adenoviruses. Hum Gene Ther. 1998;9(13):1909–17. doi:10.1089/hum.1998.9.13-1909.
- Pollard AJ, Launay O, Lelievre JD, Lacabaratz C, Grande S, Goldstein N, Robinson C, Gaddah A, Bockstal V, Wiedemann A, et al. Safety and immunogenicity of a two-dose heterologous Ad26.ZEBOV and MVA-BN-Filo Ebola vaccine regimen in adults in Europe (EBOVAC2): A randomised, observer-blind, participant-blind, placebo-controlled, phase 2 trial. Lancet Infect Dis. 2021;21(4):493–506. doi:10.1016/S1473-3099(20)30476-X.
- Sadoff J, Gray G, Vandebosch A, Cardenas V, Shukarev G, Grinsztejn B, Goepfert PA, Truyers C, Fennema H, Spiessens B, et al. Safety and efficacy of single-dose Ad26.COV2.S vaccine against Covid-19. N Engl J Med. 2021;384(23):2187–201. doi:10.1056/NEJMoa2101544.
- Custers J, Kim D, Leyssen M, Gurwith M, Tomaka F, Robertson J, Heijnen E, Condit R, Shukarev G, Heerwegh D, et al. Vaccines based on replication incompetent Ad26 viral vectors: Standardized template with key considerations for a risk/benefit assessment. Vaccine. 2021;39:3081–101. doi:10.1016/j.vaccine.2020.09.018.
- Leroux-Roels I, Leroux-Roels G, Shukarev G, Schuitemaker H, Cahill C, de Rooij R, Struijs M, van Zeeburg H, Jacquet JM. Safety and immunogenicity of a new Sabin inactivated poliovirus vaccine candidate produced on the PER.C6® cell-line: A phase 1 randomized controlled trial in adults. Hum Vaccin Immunother. 2021;17(5):1366–73. doi:10.1080/21645515.2020.1812315.
- Liao G, Li R, Li C, Sun M, Li Y, Chu J, Jiang S, Li Q. Safety and immunogenicity of inactivated poliovirus vaccine made from Sabin strains: A phase II, randomized, positive-controlled trial. J Infect Dis. 2012;205(2):237–43. doi:10.1093/infdis/jir723.
- Liao G, Li R, Li C, Sun M, Jiang S, Li Y, Mo Z, Xia J, Xie Z, Che Y, et al. Phase 3 trial of a Sabin strain–based inactivated poliovirus vaccine. J Infect Dis. 2016;214(11):1728–34. doi:10.1093/infdis/jiw433.
- Okada K, Miyazaki C, Kino Y, Ozaki T, Hirose M, Ueda K. Phase II and III clinical studies of diphtheria-tetanus-acellular pertussis vaccine containing inactivated polio vaccine derived from Sabin strains (DTaP-sIPV). J Infect Dis. 2013;208(2):275–83. doi:10.1093/infdis/jit155.
- Verdijk P, Rots NY, van Oijen MG, Weldon WC, Oberste MS, Okayasu H, Sutter RW, Bakker WA. Safety and immunogenicity of a primary series of Sabin-IPV with and without aluminum hydroxide in infants. Vaccine. 2014;32:4938–44. doi:10.1016/j.vaccine.2014.07.029.
- Nakano T, Sumino S, Takanami Y, Mitsuya N, Nakatome K. A phase 2 study of a combined diphtheria-tetanus-acellular pertussis vaccine with a Sabin-derived inactivated poliovirus vaccine in children. Hum Vaccin Immunother. 2018;14(12):2940–49. doi:10.1080/21645515.2018.1504538.
- Chu K, Ying Z, Wang L, Hu Y, Xia J, Chen L, Wang J, Li C, Zhang Q, Gao Q, et al. Safety and immunogenicity of inactivated poliovirus vaccine made from Sabin strains: A phase II, randomized, dose-finding trial. Vaccine. 2018;36:6782–89. doi:10.1016/j.vaccine.2018.09.023.
- Hu Y, Wang J, Zeng G, Chu K, Jiang D, Zhu F, Ying Z, Chen L, Li C, Zhu F, et al. Immunogenicity and safety of a Sabin strain–based inactivated polio vaccine: A phase 3 clinical trial. J Infect Dis. 2019;220(10):1551–57. doi:10.1093/infdis/jiy736.
- Tang R, Li G, Zhang C, Zhi H, Zhu J, Wang J, Liang Q, Hu Y, Li C. A phase ΙΙ, randomized, controlled trial to evaluate the safety and immunogenicity of a Sabin strain-based inactivated polio vaccine. Hum Vaccin Immunother. 2020;16(11):2641–48. doi:10.1080/21645515.2020.1745593.
- Cramer JP, Jimeno J, Han HH, Lin S, Hartmann K, Borkowski A, Saez-Llorens X. Safety and immunogenicity of experimental stand-alone trivalent, inactivated Sabin-strain polio vaccine formulations in healthy infants: A randomized, observer-blind, controlled phase 1/2 trial. Vaccine. 2020;38:5313–23. doi:10.1016/j.vaccine.2020.05.081.
- Capeding MR, Gomez-Go GD, Oberdorfer P, Borja-Tabora C, Bravo L, Carlos J, Tangsathapornpong A, Uppala R, Laoprasopwattana K, Yang Y, et al. Safety and immunogenicity of a new inactivated polio vaccine made from Sabin strains: A randomized, double-blind, active-controlled, phase 2/3 seamless study. J Infect Dis. 2020. doi:10.1093/infdis/jiaa770.
- Piniaeva A, Ignatyev G, Kozlovskaya L, Ivin Y, Kovpak A, Ivanov A, Shishova A, Antonova L, Khapchaev Y, Feldblium I, et al. Immunogenicity and safety of inactivated Sabin-Strain polio vaccine “PoliovacSin”: Clinical trials phase I and II. Vaccines (Basel). 2021;9(6):565. doi:10.3390/vaccines9060565.
- World Medical Association. World Medical Association Declaration of Helsinki: Ethical principles for medical research involving human subjects. JAMA. 2013;310:2191–94. doi:10.1001/jama.2013.281053.
- European Medicines Agency (EMA). Guideline for good clinical practice E6(R2). Step 5. [ accessed 2021 June 30]. https://www.ema.europa.eu/en/documents/scientific-guideline/ich-e-6-r2-guideline-good-clinical-practice-step-5_en.pdf .
- World Health Organization & WHO Expert Committee on Biological Standardization. WHO Expert Committee on Biological Standardization, sixty-fifth report. World Health Organ Tech Rep Ser. 2015;1–262. [Accessed 2022 February 28]. https://apps.who.int/iris/handle/10665/173739 .
- Crawt L, Atkinson E, Tedcastle A, Pegg E, Minor P, Cooper G, Rigsby P, Martin J Report on the WHO collaborative study to establish the 1st International Standard for Sabin inactivated polio vaccine (sIPV). [ accessed 2018 October 29 – November 2]. https://cdn.who.int/media/docs/default-source/biologicals/bs-documents-(ecbs)/2019-documents/bs.2018.2338_sipv_is_collaborative_study_report_version.pdf?sfvrsn=9b150f78_5&download=true .
- Jia S, Tang R, Li G, Hu Y, Liang Q. The effect of maternal poliovirus antibodies on the immune responses of infants to poliovirus vaccines. BMC Infect Dis. 2020;20(1):641. doi:10.1186/s12879-020-05348-1.
- Voysey M, Kelly DF, Fanshawe TR, Sadarangani M, O’-Brien KL, Perera R, Pollard AJ. The influence of maternally derived antibody and infant age at vaccination on infant vaccine responses: An individual participant meta-analysis. JAMA Pediatr. 2017;171(7):637–46. doi:10.1001/jamapediatrics.2017.0638.
- Bravo LC, Carlos JC, Gatchalian SR, Montellano MEB, Tabora C, Thierry-Carstensen B, Tingskov PN, Sorensen C, Wachmann H, Bandyopadhyay AS, et al. Immunogenicity and safety of an adjuvanted inactivated polio vaccine, IPV-Al, compared to standard IPV: A phase 3 observer-blinded, randomised, controlled trial in infants vaccinated at 6, 10, 14 weeks and 9 months of age. Vaccine. 2020;38(3):530–38. doi:10.1016/j.vaccine.2019.10.064.