ABSTRACT
Botulinum neurotoxins (BoNTs) are the most toxic known proteins. Naturally occurring botulism in humans is caused by botulinum serotypes A, B, E, and F. Vaccination is an effective strategy to prevent botulism. In this study, a tetravalent botulinum vaccine (TBV) that can prevent serotypes A, B, E, and F was developed using the C-terminal receptor-binding domain of BoNT (Hc) as an antigen. To develop a suitable vaccine formulation, in vitro binding experiments of antigens and aluminum adjuvant in different buffers, and in vivo experiments of TBV at different antigen concentrations, were conducted. Our results showed that the optimal vaccine formulation buffer was a pH 6.0 phosphate buffer, and the suitable antigen concentration was 40 or 80 µg/ml of each antigen. A pilot-scale TBV was then prepared and evaluated for immunogenicity and stability. The results showed that TBV could elicit strong protective efficacy against each BoNT in mice, and remain effective after two years of storage at 4ºC, indicating that the preparation was stable and highly effective. Adsorption experiments also showed that the antigens could be well adsorbed by the aluminum adjuvant after 2 years of storage. Our results provide valuable experimental data supporting the development of a tetravalent botulinum vaccine, which is a promising candidate for the prevention of botulinum serotypes A, B, E, and F.
Introduction
Botulism is a serious illness caused by poisoning with neurotoxins produced by several genetically distinct groups of the bacterial species Clostridium botulinum (C. botulinum).Citation1,Citation2 There are seven serotypes of botulinum neurotoxins (BoNTs), among which serotypes A, B, E, and F cause botulism in humans, while serotypes C, D, and G cause botulism in birds, horses, and cattle. Novel BoNT types (e.g., BoNT/HA and BoNT/X) and BoNT-like proteins have been identified in recent years,Citation3 indicating the variability and diversity of BoNTs. Novel Clostridium botulinum neurotoxins raise several research concerns. Human botulism can take several forms: foodborne botulism, infant botulism, inhalation botulism, adult intestinal toxemia botulism, wound botulism, and iatrogenic botulism.Citation4,Citation5
BoNTs of various serotypes are structurally and functionally similar and are initially synthesized as 150-kDa proteins, and then cleaved into a 50-kDa light chain and 100-kDa heavy chain joined by a disulfide bond. Each BoNT consists of three functional domains: an N-terminal catalytic domain (light chain), an internal heavy-chain translocation domain (Hn domain, 50-kDa), and a C-terminal heavy chain receptor-binding domain (Hc domain, 50-kDa).Citation6,Citation7 The process of poisoning is divided into three steps. Through blood circulation, the C-terminus of the heavy chain binds to receptors on the nerve ending membrane,Citation8 which triggers the translocation of BoNT into the neuronal cytosol via receptor-mediated endocytosis. Upon entry into the cytoplasm, the light chain, which takes the form of a zinc endopeptidase, cleaves polypeptides that are essential for the release of neurotransmitters including acetylcholine into the synaptic cleft. Thereby, the extracellular release of neurotransmitters into the neuromuscular junction is blocked, resulting in paralysis of the airways and respiratory muscles that can lead to ventilation failure and death.Citation5
BoNTs have been classified as an important biological threat because of their high toxicity and lethality.Citation8 Vaccination is an effective strategy to prevent botulism and provide a source for the production of a therapeutic antitoxin.Citation9 An investigational pentavalent vaccine (Pentavalent Botulinum Toxoid Vaccine, PBT) is the mostly used human botulism vaccine, which induces neutralizing antibodies against BoNT serotypes A, B, C, D, and E. However, the use of PBT was limited to laboratory workers and soldiers at potential risk for botulism, and it failed to obtain Food and Drug Administration (FDA) approval for licensing due a high risk of allergic reactions and other side-effects.Citation10 Therefore, there is an urgent need to develop an efficient, safe, and convenient vaccine for the prevention of botulism.
A novel vaccine-design strategy has been developed using the nontoxic recombinant domains of BoNTs as antigens, but not the full toxin.Citation11,Citation12 Previous animal studies have demonstrated that the HC domains are nontoxic, and sufficient to generate a protective immune response against natural BoNTs. Besides, the recombinant Hc fragments can be synthesized in large quantities, and are less hazardous than traditional toxin-inactivated vaccines.Citation13,Citation14 In recent years, several botulism vaccines have been developed using recombinant proteins. A bivalent recombinant subunit vaccine rBV A/B for the prevention of BoNT/A and BoNT/B was developed, using the yeast-expressed recombinant BoNT Hc domain as an antigen, and is undergoing phase II clinical trials.Citation15–17 However, there is currently no available vaccine that can simultaneously prevent BoNT serotypes A, B, E, and F, which are known to cause botulism in humans.
In this study, we successfully developed a tetravalent botulinum vaccine using recombinant non-His-tagged Hc domains of botulinum serotypes A, B, E, and F (AHc, BHc, EHc, and FHc). In previous studies, the recombinant Hc antigens produced by our team were proven to be highly immunogenic and potent.Citation18–21 Here, we first developed a formula in which four antigens could be well adsorbed by an aluminum adjuvant and prepared the tetravalent botulinum vaccine (TBV) with pilot-scale purified antigens. Then, the TBV was evaluated for immunogenicity and stability. We also explored the protective efficacy of TBV against botulinum serotypes A, B, E, and F in mice.
Material and method
Animals
Balb/c mice (female, 6–8 weeks) and Kunming (KM) mice (female, 15–18 g) were purchased from Beijing Laboratory Animal Center (Beijing, China) and housed in pathogen-free conditions. All animal experiments were approved by our Institution Animal Care and Use Committee (Beijing Institute of Biotechnology) and performed in accordance with the ethical guidelines of our institution.
Preparation and characterization of each Hc antigen
Recombinant non-tagged Hc proteins of botulinum serotype A, B, E, and F (AHc, BHc, EHc, and FHc) were prepared and characterized in this study. Briefly, AHc, EHc, and FHc proteins expressed in E. coli and BHc protein expressed in yeast were purified by sequential chromatography. A 30 L pilot-scale purification of each Hc antigen was performed at the Pilot Production Base of our institute as previously described.Citation18–21 The character of pilot-scale Hc antigen was determined and qualified recombinant protein batches were stored at −80ºC for further studies.
Adsorption capacity assay of antigen by aluminum adjuvant in different buffer system
The vaccine formulation consists of buffer system, aluminum hydroxide adjuvant (Aluminum hydroxide gel, Brenntag Biosector, Denmark,aluminum 13 mg/mL), a stabilizer (5% mannitol), a preservative (.25% m-cresol), and antigens. To determine the optimal formulation of TBV, antigen adsorption experiments by aluminum hydroxide adjuvant under different buffer systems were conducted.Citation22 The selected buffer system includes phosphate, acetate, citrate, and succinate with 20 mM final concentration,Citation15,Citation23–25 which are common buffer systems used in vaccine studies. In all buffer systems, the concentration of aluminum adjuvant is 1 mg/mL. Antigens were formulated with aluminum hydroxide in buffer systems containing other components of TBV, and the adsorption capacity assay was conducted referring to Pharmacopoeia of the People’s Republic of China (PPRC, Appendix XII 3501: In vitro relative viability test of recombinant hepatitis B vaccine). Briefly, after incubating for 1 hour at room temperature, the mixture was centrifuged at 11,500 rpm for 5 min, and the supernatant was collected and the precipitate were resuspended in PBS (pH 7.4). The protein content in the supernatant and resuspended precipitate were determined by SDS-PAGE and NanoDrop Protein Quantification (Thermo Fisher Scientific Inc., Waltham, MA, USA). The optimal buffer system was determined according to the results of antigen adsorption by the aluminum hydroxide adjuvant.
Preparation and characterization of tetravalent vaccine
TBV with different buffer systems and different antigen concentrations were prepared according to the results of antigen adsorption. Briefly, monovalent vaccine of each BoNT serotype was prepared first. The desired monovalent vaccine with four times of each BoNT Hc antigen was mixed with other components of the vaccine and incubated at room temperature for 1 hour to allow the adjuvant to adsorb the antigens. TBV was developed by mixing equal volume of each monovalent vaccine and stored at 4ºC in separate packages. The adsorption capacity of TBVs wAS characterized as described above. TBV with 40 µg/mL of each antigen in pH 6.0 phosphate buffer system and pH 5.5 acetate buffer system was developed in the buffer system study. TBV with 40 µg/mL each antigen, 80 µg/mL each antigen, and 80 µg/mL of serotypes E/F antigen +120 µg/mL serotypes A/B antigen were developed in the antigen concentration study.
Immunization of mice and challenge with BoNTs
Balb/c mice (6–8 weeks) were randomly assigned to different treatment groups (8 mice per group). Mice were vaccinated intramuscularly (i.m.) twice with vaccines of different formulation.Citation20 TBV with Freund’s adjuvant (Sigma-Aldrich, Inc., St Louis, MO, USA) was prepared according the manufacturer’s instructions, in which complete Freund’s adjuvant (F5581) was used for the first immunization and incomplete Freund’s adjuvant (F5506) was used for the second immunization. A formulation without any antigens was used as a negative control. The monovalent vaccines of the four recombinant Hc antigen were used as positive control for the toxin challenge assays. The injections were administered at three-week intervals with 100 µl vaccine formulation. Three weeks after two vaccinations, mice were challenged with different doses of BoNTs by intraperitoneal injection and observed for 7 days to record their survival. According to previous studies, the suitable challenge dose were .5 mL/mouse per mouse containing an active preparation containing 105 LD50 BoNT/A, 104 LD50 BoNT/B, 103 LD50 BoNT/E, or 103 LD50 BoNT/F, respectively.Citation18–21 The BoNTs were assayed using botulinum antitoxin standard (from National Institutes of Food and Drug Control, Beijing, China).
The neurotoxicity of the BoNTs used in mice was determined using an LD50 assay. Groups of four mice were used for each concentration, and the BoNTs were diluted in sterile normal saline. The lowest amount of toxin that killed 50% of 20 g mice within four days was considered to be one minimal lethal dose (LD50), and was expressed as the number of LD50 units/mg of protein. In our study, the toxicity of BoNT/A is 3 × 107 LD50/mg; the toxicity of BoNT/B is 2 × 107 LD50/mg; the toxicity of BoNT/E is 1 × 107 LD50/mg; the toxicity of BoNT/F is 1 × 107 LD50/mg.
Serum antibody titer measurement and BoNTs neutralization assay
Sera from mice in different treatment groups were screened for anti-Hc antibody titer by ELISA as previously describe.Citation20 Sera antibody titers of individual mice were assayed, and the geometric mean titer (GMT) for each group was determined.
The neutralizing potency of the mouse sera was measured using a BoNT neutralization assay as previously described.Citation19 Briefly, serially diluted anti-BoNT sera were mixed with a standard concentration of each BoNT (102 LD50/mL), and incubated for 15 min at 37°C. KM mice (15–18 g) were intraperitoneally (i.p.) injected with the mixtures in a total volume of .5 mL/mouse (four mice in each group), then monitored for 7 days to record their survival. The neutralizing antibody titers in the sera were calculated as international units per milliliter (IU/mL) according to the WHO (World Health Organization) BoNT antitoxins. An IU for botulinum neurotoxin serotypes A, B, and F is defined as the neutralizing antibody that can neutralize 104 mouse LD50 of BoNTs, whereas for neurotoxin serotype E, neutralizes 103 mouse LD50 of BoNT/E.
Stability evaluation of the tetravalent vaccine
The TVB stored at 4°C was taken out at 1, 6, 12, 24 months for stability evaluation. The vaccine samples were centrifuged at 11,500 rpm (12,420 g) for 5 min, and the supernatant was collected and the precipitate were resuspended in equal volume of PBS (pH 7.4). Then, the supernatant and the resuspended precipitate were subjected to 12% SDS-PAGE to detect the protein content and NanoDrop Protein Quantification.
Effectiveness evaluation of the tetravalent vaccine
The TBV samples with 80 µg/mL each antigen concentration were taken out after stored at 4°C for 12 months and 24 months to immune Balb/c mice (10 mice per group). A formulation without antigen was used as a negative control. The injections were administered at three-week intervals. Three weeks after the second vaccination, mice were blood sampled and subjected to each BoNT challenge. The sera samples were screened for antibody titer measurement and BoNTs neutralization assay.
Statistical analysis
Differences in the antibody immune responses were analyzed statistically by ANOVA one-way variance test or using the Student’s t-test for the analysis of differences between groups. Fisher’s exact test was used to determine statistical differences in survival between the treatment groups. For all tests, only data resulting in P values <0.05 were regarded as statistically significant.
Results
The in vitro adsorption of Hc antigens by aluminum adjuvant in different formula
Recombinant botulinum Hc antigens were prepared and characterized as previously described.Citation18–21 Qualified pilot-scale antigen batches were used for the vaccine formula study.
Taking into account the isoelectric point of the antigens (AHc 9.1, BHc 6.9, EHc 9.1, FHc 8.9), which affect the ability of the protein to bind to the adjuvant, phosphate, acetate, citrate, and succinate buffer systems under acidic conditions were chosen for the adsorption experiments of antigen by aluminum adjuvant. The adsorption efficiency of AHc was first studied, the results showed that the aluminum adjuvant had the highest adsorption efficiency under pH 6.0 phosphate buffer (93.1%) and pH 5.5 acetate (90.9%) ().
Table 1. The adsorption rate of AHc antigen by aluminum adjuvant in different buffer systems
Then, the adsorption of the four recombinant antigens with final concentration of 200 µg/mL to 600 µg/mL under these two buffer systems are further studied. All four antigens could be well adsorbed by aluminum adjuvant. The maximum adsorption capacity is above 395 µg antigen/mg aluminum adjuvant for AHc, BHc, FHc, and 308 µg antigen/mg adjuvant for EHc, which is satisfactory for the preparation of the tetravalent botulinum vaccine.
In vivo characterization of tetravalent botulinum vaccine
TBV in different buffer systems with 40 µg/mL each antigen was prepared and characterized in Balb/C mice. The results of the serum antibody titers showed TBV in both phosphate buffer (PB) and acetate buffer (AC) induced strong immune response as the monovalent vaccines (). The anti-EHc antibody titers from TBV in both PB and AC are significantly better than that of TBV in Freund’s Adjuvant group (P < .05). In the BoNT challenge experiment, mice with two injections of TBV in PB were completely protected against four kinds of botulinum, which is comparable to the monovalent vaccines and better than the TBVs in AC buffer and Freund’s Adjuvant with incomplete protections. There is not protective potency in the negative control (). The results indicate that PB may be the most suitable buffer system for TBV.
Figure 1. Serum antibody titers against each antigen in mice immunized with TBV in different buffer systems. 1TVB in PB; 2tvb in AC; 3tvb in Freund’s Adjuvant; 4monovalent vaccines refer to the monovalent vaccine of each serotype with the same dose of AHc, BHc, EHc and FHc, in PB buffer containing aluminum adjuvant. the final concentration of each serotype of antigen is 40 µg/ml and the injection dose is 100 µl per mice. Serum samples from individual mice of each group (n = 8) were collected after two immunizations and specific antibody titers were measured by ELISA. Serum sample from individual mice were assayed and the geometric mean titer (GMT) was calculated for each group.
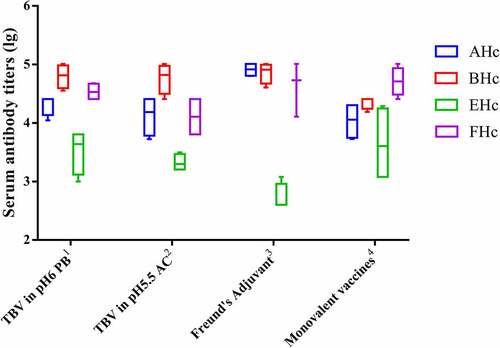
Table 2. Protection efficacy of TBV in different buffer systems
TBV in PB buffer with different antigen concentrations were prepared and characterized. Considering BoNT serotype A and B are more important, the final antigen concentrations were 40 µg/mL each antigen, 80 µg/mL each antigen, and120 µg/mL for AHc and BHc & 80 µg/mL for EHc and FHc (A/B 120 µg/mL + E/F 80 µg/mL). These results showed that all three specifications of TBV could protect mice against 105 LD50 BoNT/A, 104 LD50 BoNT/B, 103 LD50 BoNT/E, and BoNT/F challenge, respectively. The antibody titers in the sera were all higher than 1.5 IU/mL. TVB with 80 µg/mL each antigen elicited stronger antibody responses than that with 40 µg/mL each antigen (). However, after the concentrations of antigen AHc and BHc were increased to 120 µg/mL, the antibody titers did not increase and even decreased significantly (serotypes E and F, P < .05, ). This may be due to the competitive binding of antigens with the aluminum adjuvant considering the maximum adsorption capacity (308 µg antigen EHc/mg aluminum adjuvant).
Figure 2. Serum antibody titer in mice immunized with TBV in PB buffer with different antigen concentrations. The final antigen concentrations are 40 µg/ml for each antigen, 80 µg/ml for each antigen, and 120 µg/ml of type A/B antigen combined with 80 µg/ml of type E/F antigen. The injection dose is 100 µl per mice for each preparation. Therefore, the antigen dose is given in these immunization group. Serum samples from individual mice of each group (n = 10) were collected after two immunizations and specific antibody titers were measured by ELISA. Serum sample from individual mice sere assayed and the geometric mean titer (GMT) was calculated for each group.
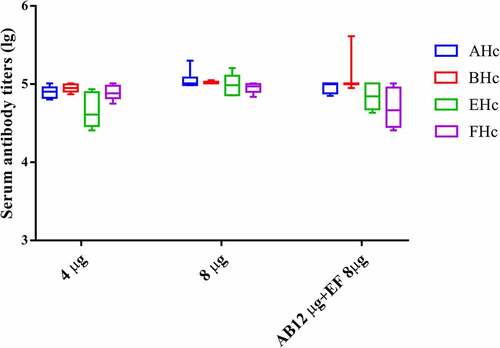
Table 3. Survival and serum neutralization titers of mice after two vaccinations of TBV with different antigen doses
Pilot scale preparation and effectiveness of tetravalent botulinum vaccine
Based on the above results, pilot scale TBV was prepared and evaluated for immunogenicity and stability. TBV in PB buffer with final antigen concentrations of 40 µg/mL and 80 µg/mL wwas prepared and characterized. The vaccine formulation consists of buffer system, aluminum hydroxide adjuvant (aluminum content 1 mg/mL), a stabilizer (5% mannitol), a preservative (0.25% m-cresol) and four kinds of antigens. Based on the above research results, in this study, each antigen of 40 µg/mL and 80 µg/mL is chosen to prepare the finished products.
We then studied the effectiveness and quality standards of the finished vaccine. The adsorption experiments of the vaccine samples were carried out and the samples were analyzed by SDS-PAGE and Western blot (). These results showed that all four recombinant antigens could be well adsorbed by the aluminum adjuvant and be bound by anti-neurotoxin antibodies of each BoNT serotype, indicating that the antigens have correct antigenicity.
Figure 3. Adsorption assay of aluminum adjuvant for tetravalent vaccine of pilot test antigen. Briefly, 200 µl of TBV samples with 40 µg/ml antigen concentration and 100 µl of TBV samples with 80 µg/ml antigen concentration was centrifuged at 11,500 rpm (12,420 g) for 5 min and the supernatant was collected and the precipitate were resuspended in 20 µl PBS (pH 7.4). the protein content in the supernatant and resuspended precipitate were determined by SDS-PAGE of 10 µl above samples and Western blot analysis using hyper immune anti-neurotoxin sera of each BoNT serotype. a: Resuspended precipitate and supernatant of TBV. Lanes 1–2, resuspended precipitate of TBV with 40 µg/ml and 80 µg/ml antigen concentration, the order of BoNT antigens in terms of molecular weight as followed are BHc, AHc, EHc, FHc, from top to bottom; Lanes 3–4, supernatant of TBV with 40 µg/ml and 80 µg/ml antigen concentration; b: Western blot analysis of AHc antigen in TBV using horse sera of anti-neurotoxin A, Lanes 5–6; c: Western blot analysis of BHc antigen in TBV using horse sera of anti-neurotoxin B, Lanes 7–8; d: Western blot analysis of EHc antigen in TBV using horse sera of anti-neurotoxin E, Lanes, 9–10; e: Western blot analysis of FHc antigen in TBV using horse sera of anti-neurotoxin F, Lanes 11–12; M1, protein markers 66.2, 45, and 35 kDa (from top to bottom); M2, protein markers 180,130, 95, 72, 55, 43, 34, and 26 kDa (from top to bottom); the horse sera of anti-neurotoxin A, B, E, and F were prepared and characterized in previous studies.Citation18–21
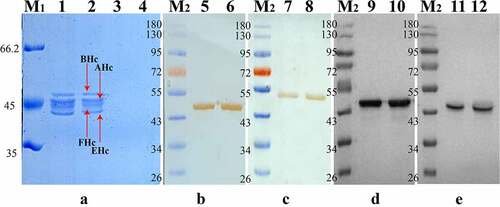
The in vivo characterization of tetravalent botulinum vaccine results () showed that both TBV with 40 µg/mL and 80 µg/mL antigen concentration could provide complete protection against four kinds of BoNT challenge (105 LD50 BoNT/A, 104 LD50 BoNT/B, 103 LD50 BoNT/E and BoNT/F) in mice. The sera neutralization assay indicate that the sera antibodies induced by TBV have biologically active neutralizing activity, and the antibody titers against four kinds of BoNTs are above 1 IU/mL. The above results show that the pilot scale TBV preparations possess high formula quality and good immunological effectiveness.
Table 4. Survival, group antibody ELISA titers, and serum neutralization titers of mice after vaccination with pilot scale TBV
Evaluation on the long-term effectiveness and stability of TBV
TBV was stored at 4°C for two years and assayed for its stability and immunity. The adsorption experiments showed that the antigens could still be well adsorbed by the aluminum adjuvant after being stored for two years. Our data also found that there was no protein in the supernatant of TBV by SDS-PAGE and NanoDrop Protein Quantification. The immunity assay in animals showed that TBV could elicit comparable immune responses and protect adequate protection against BoNT challenge after being stored for two years compared with the original stock (p < .05). The serum antibody titers to four types of neurotoxins were above 1 IU/mL (). These results indicate that TBV has good stability and remain effective after stored for two years.
Table 5. Survival, group antibody ELISA titers, and serum neutralization titers of mice after vaccination with TBV stored at 4°C for one or two years
Discussion
Botulinum neurotoxins (BoNTs) are the most toxic known proteins, and are easy to manufacture, deliver, and weaponize. Developing suitable drugs for the prevention and treatment of botulinum is of vital importance.Citation8 Vaccination is an effective way to prevent botulinum neurotoxin poisoning. In previous studies, the recombinant Hc antigens of BoNT serotypes A, B, E, and F, known to cause botulism in humans, were proved to be highly immunogenic and potent, and can be used as a monovalent vaccine against botulism.Citation18–21
In this study, a new TBV was developed and characterized. After two immunizations, TBV induced high titers of sera antibodies that protected mice against 105 LD50 BoNT/A, 104 LD50 BoNT/B, 103 LD50 BoNT/E, and BoNT/F challenges, respectively. The neutralizing antibody titers of the sera were above 1 IU/mL, which is much higher than the previously widely used toxoid vaccine.Citation26 The PBT vaccine previously administered in a clinical trial is not used because of its shortcomings.Citation27 This toxoid vaccine will be replaced by a bivalent Hc rBV A/B vaccine. The potency of our TBV is equivalent to this bivalent Hc rBV A/B vaccine evaluated in a Phase II clinical trial.Citation9, Citation17 These nontoxic recombinant BoNT Hc domains, synthesized in E. coli or yeast, are less expensive and less hazardous to prepare than the traditional toxin-inactivated vaccines, and can induce high levels of neutralizing antibodies to efficiently protect against BoNTs. Moreover, the TBV is a tetravalent Hc subunit vaccine that can prevent four botulinum serotypes simultaneously. The rBV A/B vaccine cannot protect against BoNT/E and BoNT/F, and the PBT vaccine also cannot protect against BoNT/F. Therefore, TBV is an effective and promising vaccine that can be used for the prevention of botulism.
Considering the importance of formulas in multivalent vaccines, developing a formulation in which four kinds of recombinant antigens with different isoelectric point (pI) can function simultaneously is very difficult. The formula for TBV was specially developed and the maximum binding concentration of each type of antigen was determined. This is the first study that describes the development of a tetravalent botulinum vaccine using recombinant antigens. Recombinant antigens have the advantages of low toxicity and high immunogenicity but have different characteristics. Thus, allowing four recombinant antigens to work effectively requires an accurate buffer system.
After considering the isoelectric point of these antigens, the buffer systems of different salts and pH values were studied, and the buffer system of the TBV was determined. The results indicate that all four antigens are well adsorbed by aluminum adjuvant at a pH of 6.0 PB and pH 5.5 Ac buffer system, with above 395 µg antigen/mg aluminum adjuvant for AHc, BHc, and FHc and 308 µg antigen/mg adjuvant for EHc, which is satisfactory for the preparation of the tetravalent botulinum vaccine.Citation22 The animal experiments further proved that pH6.0 PB as a vaccine formulation had a complete protective effect and was identified as the optimal choice. Therefore, pH6.0 PB was used as a preparation for the development of this tetravalent vaccine. The formulation using pH 6.0 phosphate buffer and aluminum adjuvant was first developed to allow the four antigens to work simultaneously.
Another important factor that needs to be considered is the concentration of the antigens in the TBV formula. Based on the maximum antigen-binding capacity of the aluminum adjuvant, a TBV with a final antigen concentration of 40 µg/mL, 80 µg/mL, and AHc/BHc 120 µg/mL & EHc/FHc 80 µg/mL was designed and evaluated. The results showed that the immunity of the TBV was significantly enhanced after increasing the antigen concentration to 80 µg/mL. However, when the AHc and BHc antigen concentrations were increased to 120 µg/mL, the antibody responses against serotypes A and B did not increase, but the antibody responses against serotypes E and F decreased significantly, indicating that competitive binding to aluminum adjuvant occurred between these antigens. These results suggested that the competitive binding problem must be considered when developing multivalent vaccines.
We also observed that there was an obvious antigen protein in the supernatant of the TBV based on SDS-PAGE and NanoDrop Protein Quantification. Without increasing the final amount of aluminum adjuvant in the TBV, adding other antigens did not improve its immunogenicity and protection, but may have affected the stability and effectiveness of the preparation. Therefore, under the premise of sufficient protection, it is a reasonable choice to lower the dose of each antigen appropriately. The two specifications of a TBV with either a 40 µg/mL or 80 µg/mL antigen concentration were both effective. Therefore, our study provided valuable experience for the future development of multivalent vaccines for other pathogens.
In summary, we successfully developed a TBV using recombinant antigens of BoNT/A, B, E, and F. The vaccine formulation consists of a pH6.0 PB buffer system (20 mM), aluminum hydroxide adjuvant (aluminum 1 mg/mL), a stabilizer (5% mannitol), a preservative (0.25% m-cresol), and 40 µg/mL or 80 µg/mL each antigen. The TBV formula allows the four antigens to be well-adsorbed by the aluminum adjuvant at the same time, and it remains stable and effective after being stored at 4°C for two years. The TBV is also efficient in protecting mice against each BoNT challenge and remains efficient after being stored at 4°C for two years. The efficacy of TBV against each BoNT subtype should be refined in future experiments, which will further support its protective potency. The pre-clinical safety and efficacy of TBV need to be further characterized. After completing pre-clinical toxicity and safety studies, human clinical trials of this BoNT vaccine will be initiated.
Supplemental Material
Download MS Word (1.8 MB)Disclosure statement
No potential conflict of interest was reported by the author(s).
Supplementary material
Supplemental data for this article can be accessed on the publisher’s website at https://doi.org/10.1080/21645515.2022.2048621
Additional information
Funding
References
- Rossetto O, Montecucco C. Tables of Toxicity of Botulinum and Tetanus Neurotoxins Toxins 2019;11(12):686. doi:10.3390/toxins11120686.
- Hill KK, Smith TJ, Helma CH, Ticknor LO, Foley BT, Svensson RT, Brown JL, Johnson EA, Smith LA, Okinaka RT, et al. Genetic diversity among Botulinum Neurotoxin-producing clostridial strains. J Bacteriol. 2007;189(3):818–8. doi:10.1128/jb.01180-06.
- Tehran DA, Pirazzini M. Novel botulinum neurotoxins: exploring underneath the iceberg tip. Toxins. 2018;10(5):10. doi:10.3390/toxins10050190.
- Dembek ZF, Smith LA, Rusnak JM. Botulism: cause, effects, diagnosis, clinical and laboratory identification, and treatment modalities. Disaster Med Public Health Prep. 2007;1(2):122–34. doi:10.1097/DMP.0b013e318158c5fd.
- Smith LA. Botulism and vaccines for its prevention. Vaccine. 2009;27(Suppl 4):D33–9. doi:10.1016/j.vaccine.2009.08.059.
- Pirazzini M, Rossetto O, Eleopra R, Montecucco C. Botulinum neurotoxins: biology, pharmacology, and toxicology. Pharmacol Rev. 2017;69(2):200–35. doi:10.1124/pr.116.012658.
- Sinha J, Inan M, Fanders S, Taoka S, Gouthro M, Swanson T, Barent R, Barthuli A, Loveless BM, Smith LA, et al. Cell bank characterization and fermentation optimization for production of recombinant heavy chain C-terminal fragment of botulinum neurotoxin serotype E (rBonte(hc): Antigen E) by Pichia pastoris. J Biotechnol. 2007;127(3):462–74. doi:10.1016/j.jbiotec.2006.07.022.
- Shukla HD, Sharma SK. Clostridium botulinum: a bug with beauty and weapon. Crit Rev Microbiol. 2005;31(1):11–18. doi:10.1080/10408410590912952.
- Sundeen G, Barbieri JT. Vaccines against botulism. Toxins. 2017;9(9):26. doi:10.3390/toxins9090268.
- Webb RP, Smith LA. What next for botulism vaccine development? Expert Rev Vaccines. 2013;12(5):481–92. doi:10.1586/ERV.13.37.
- Lou J, Marks JD. Botulinum Neurotoxins (BoNTs)-antibody and vaccine. Toxins 2018;10(12):495. doi:10.3390/toxins10120495.
- Webb RP, Smith TJ, Smith LA, Wright PM, Guernieri RL, Brown JL, Skerry JC. Recombinant Botulinum Neurotoxin Hc Subunit (BoNT Hc) and Catalytically Inactive Clostridium botulinum Holoproteins (ciBont HPs) as vaccine candidates for the prevention of botulism. Toxins. 2017;9(9):9. doi:10.3390/toxins9090269.
- Zichel R, Mimran A, Keren A, Barnea A, Steinberger-Levy I, Marcus D, Turgeman A, Reuveny S. Efficacy of a potential trivalent vaccine based on Hc fragments of botulinum toxins A, B, and E produced in a cell-free expression system. Clin Vaccine Immunol. 2010;17(5):784–92. doi:10.1128/CVI.00496-09.
- Moreira G, Cunha C, Salvarani F, Gonçalves L, Pires P, Conceição F, Lobato F. Production of recombinant botulism antigens: A review of expression systems. Anaerobe. 2014;28:130–36. doi:10.1016/j.anaerobe.2014.06.003.
- Shearer JD, Manetz TS, House RV. Preclinical safety assessment of recombinant botulinum vaccine A/B (rBV A/B). Vaccine. 2012;30(11):1917–26. doi:10.1016/j.vaccine.2012.01.035.
- Rasetti-Escargueil C, Popoff MR. Antibodies and vaccines against botulinum toxins: available measures and novel approaches. Toxins. 2019:11. doi:10.3390/toxins11090528.
- Khouri JM, Motter RN, Arnon SS. Safety and immunogenicity of investigational recombinant botulinum vaccine, rBV A/B, in volunteers with pre-existing botulinum toxoid immunity. Vaccine. 2018;36(15):2041–48. doi:10.1016/j.vaccine.2018.02.042.
- Yu YZ, Na L, Zhu HQ, Wang RL, Yun D, Shuang W, Yu WY, Sun ZW. The recombinant Hc subunit of Clostridium botulinum neurotoxin serotype A is an effective botulism vaccine candidate. Vaccine. 2009;27(21):2816–22. doi:10.1016/j.vaccine.2009.02.091.
- Liu B, Shi D, Chang S, Gong X, Yu Y, Sun Z, Wu J. Characterization and immunological activity of different forms of recombinant secreted Hc of botulinum neurotoxin serotype B products expressed in yeast. Sci Rep. 2015;5:7678. doi:10.1038/srep07678.
- Shi DY, Liu FJ, Mao YY, Cui RT, Lu JS, Yu YZ, Dong XJ, Yang ZX, Sun ZW, Pang XB. Development and evaluation of candidate subunit vaccine and novel antitoxin against botulinum neurotoxin serotype E. Hum Vaccin Immunother. 2020;16:100–08. doi:10.1080/21645515.2019.1633878.
- Yu YZ, Li N, Wang RL, Zhu HQ, Wang S, Yu WY, Sun ZW. Evaluation of a recombinant Hc of clostridium botulinum neurotoxin serotype F as an effective subunit vaccine. Clin Vaccine Immunol CVI. 2008;15:1819. doi:10.1128/CVI.00239-08.
- Vessely C, Estey T, Randolph TW, Henderson I, Nayar R, Carpenter JF. Effects of solution conditions and surface chemistry on the adsorption of three recombinant botulinum neurotoxin antigens to aluminum salt adjuvants. J Pharm Sci. 2007;96:2375–89. doi:10.1002/jps.20880.
- Kissmann J, Ausar SF, Rudolph A, Braun C, Cape SP, Sievers RE, Federspiel MJ, Joshi SB, Middaugh CR. Stabilization of measles virus for vaccine formulation. Hum Vaccin. 2008;4:350–59. doi:10.4161/hv.4.5.5863.
- Vessely C, Estey T, Randolph TW, Henderson I, Cooper J, Nayar R, Braun LJ, Carpenter JF. Stability of a trivalent recombinant protein vaccine formulation against botulinum neurotoxin during storage in aqueous solution. J Pharm Sci. 2009;98:2970–93. doi:10.1002/jps.21498.
- Clark HF, Burke CJ, Volkin DB, Offit P, Ward RL, Bresee JS, Dennehy P, Gooch WM, Malacaman E, Matson D, et al. Safety, immunogenicity and efficacy in healthy infants of G1 and G2 human reassortant rotavirus vaccine in a new stabilizer/buffer liquid formulation. Pediatr Infect Dis J. 2003;22(10):914–20. doi:10.1097/01.inf.0000091887.48999.77.
- Torii Y, Tokumaru Y, Kawaguchi S, Izumi N, Maruyama S, Mukamoto M, Kozaki S, Takahashi M. Production and immunogenic efficacy of botulinum tetravalent (A, B, E, F) toxoid. Vaccine. 2002;20(19–20):2556–61. doi:10.1016/s0264-410x(02)00157-3.
- Centers for Disease Control and Prevention (CDC). MMWR Morb Mortal Wkly Rep. 2011 Notice of CDC’s discontinuation of investigational pentavalent (ABCDE) botulinum toxoid vaccine for workers at risk for occupational exposure to botulinum toxins. MMWR Morb Mortal Wkly Rep. 2011;60:1454–55.