ABSTRACT
We aimed to examine the roles of microRNA-873-5p and CXCL5 in thyroid cancer (TC) cells. qRT-PCR was adopted to measure the expression levels of CXCL5 mRNA and microRNA-873-5p in TC cells, and western blot was adopted to evaluate the CXCL5 protein expression level. Bioinformatics analysis was done to predict the upstream gene of CXCL5. Dual-luciferase assay was applied to validate the binding relationship of CXCL5 and the upstream regulatory gene. Cell experiments were done to detect the effects of microRNA-873-5p targeting CXCL5 on malignant progression of cancer cells. Western blot was adopted to demonstrate the phosphorylation level of P53 pathway related-proteins. CXCL5 was upregulated in TC cells and tissues. The results of in vitro assays displayed that CXCL5 downregulation dramatically suppressed the malignant behaviors of TC cells. MicroRNA-873-5p suppressed CXCL5 expression, but the suppressive effect of microRNA-873-5p on TC cells was abolished through CXCL5 overexpression. Additionally, microRNA-873-5p could mediate p53 pathway and thereby inhibit the malignant behaviors of TC cells through targeting CXCL5. In summary, we proved that microRNA-873-5p repressed the malignant behaviors of TC cells through targeting CXCL5 and P53 pathway, indicating that microRNA-873-5p can be a biomarker for TC.
Introduction
Tumor microenvironment (TME) refers to the complicated internal environment formed by the interaction between cancer cells and surrounding tissues, and it can affect the development and progression of cancers.Citation1 It is reported that chemokines can act as biomarkers and therapeutic targets in TME.Citation2 Chemokines can directly affect tumor growth by promoting the proliferation and inhibiting the apoptosis of tumor cells in TME.Citation3 CXCL5, as a member of the proangiogenic CXC chemokine family, can act as the ligand of CXCR2.Citation2 Relevant studies showed that CXCL5 has cancer-promoting effect on various types of malignant tumors.Citation4 For example, CXCL5-CXCR2 axis facilitates cell proliferation, promotes G1/S transition, and activates the JNK and P38 pathways in papillary thyroid carcinoma.Citation5 Overexpression of CXCL5 is associated with the development of cervical cancer.Citation6 Previous study had found that CXCL5 showed a high expression in TC, based on which we speculated that CXCL5 may act as a tumor promoting factor in TC.
MicroRNAs are short endogenous non-coding RNAs that participate in various biological activities. They can affect mRNA stability and target gene expression, thus involved in the occurrence of multiple malignant tumors.Citation7 As tumor suppressors, some microRNAs inhibit the development of TC by affecting target gene expression in TC cells.Citation8 MicroRNA-873-5p is under-expressed in a variety of tumors and has an inhibitory effect on cancer. For example, microRNA-873-5p suppresses colon cancer development via inhibiting TUSC3/AKT signaling.Citation9 Based on the bioinformatics results of the present study, we speculated that microRNA-873-5p had a similar action mechanism on CXCL5. Therefore, we conducted in-depth research on the mechanism by which microRNA-873-5p repressed the malignant process of TC cells via targeting CXCL5.
Herein, we investigated the regulatory role of microRNA-873-5p on the malignant behaviors of TC cells as well as the relationship between microRNA-873-5p and CXCL5 in TC. Elucidating the action mechanism of microRNA-873-5p will help deepen our the understanding of TC pathogenesis and is expected to offer a new therapeutic target for TC treatment.
Materials and methods
Bioinformatics analysis
The expression data of mature microRNAs (Normal: 59, Tumor: 514) and mRNAs (Normal: 58, Tumor: 510) of TC were offered by TCGA database (https://portal.gdc.cancer.gov/). Differential analysis was carried out using package “edgeR” (|logFC|>1.5, padj <0.05), and the differential expressions of microRNAs and mRNAs were obtained. The target mRNA of the present study was determined through a literature review. The upstream genes of target mRNA were predicted by starBase (http://starbase.sysu.edu.cn/) and mirDIP databases (http://ophid.utoronto.ca/mirDIP/index.jsp). Based on the prediction, the differential microRNAs with binding sites to target mRNA were obtained. Finally, the upstream microRNA was selected by correlation analysis. Gene set enrichment analysis (GSEA) was introduced to find CXCL5-involved significantly changed signaling pathways. Briefly, for GSEA analysis, the patients were classified into the high- and low-CXCL5 expression groups with the median of CXCL5 expression as the critical point. Subsequently, the enrichment result of p53-related signaling pathway could be calculated in the above two groups.
Cell culture
Normal thyroid epithelial cell-line Nthy-ori3–1 (BNCC353368) and TC cell lines, including KTC-1 (BNCC100400), TPC-1 (BNCC338689), BCPAP (BNCC100390), and K1 (BNCC337627), were purchased from BeNa Culture Collection (BNCC, China). TC cell line BHP10–3 (GDC127350528) was purchased from Shanghai Guan&Dao Biological Engineering Co., Ltd. All the cells were placed in DMEM (Thermo Fisher Scientific, USA) supplemented with 10% fetal bovine serum (FBS), 100 U/mL penicillin, and 100 μg/mL streptomycin at 37°C with 5% CO2.
Cell transfection
MicroRNA-873-5p mimics and its negative controls (NC mimics) as well as si-CXCL5 and its negative controls (si-NC) were offered by GenePharma (Shanghai, China). The recombinant expression vectors (pcDNA3.1-CXCL5) and corresponding negative controls (pcDNA3.1-NC) were constructed by transfection. Transfection was conducted using Lipofectamine 2000 (Invitrogen, USA). After 48 h, transfection efficiency was evaluated. All cells needed to be cultured in complete medium for at least 24 h before transfection.
qRT-PCR
Total RNA was extracted from cells by using Trizol reagent (Thermo Fisher Scientific, USA). The purity and the concentration of RNA were determined by SMA6000 microspectrophotometer (Merinton Instrument Co., China). Then, 1 μg total RNA in each sample was used to synthesize cDNA using TransScript first-strand cDNA Synthesis superMix (TransGen, China) and TransScript® miRNA First-Strand cDNA Synthesis SuperMix (TransGen, China), respectively. mRNA expression was analyzed by qPCR using TransStart Green qPCR SuperMix (TransGen, China) (primers are listed in ). In this study, the reverse transcription parameters of miR-873-5p were as follows: 37°C for 1 h, 85 °C for 5 s; The reverse transcription parameters of CXCL5 were 45 °C for 15 min and 85 °C for 5 s. The parameters of qPCR were: pre-denaturation at 95 ℃ for 10 min, denaturation at 95 °C for 15 s, annealing/elongation at 60 °C for 1 min, melting curve analysis at 95 °C for 15 s, 60 °C for 1 min, 95 °C for 15 s, 60 °C for 15 s. Denaturation and annealing/elongation required 35 to 40 cycles. U6 gene and GAPDH gene were used as endogenous reference genes for microRNA-873-5p and CXCL5, respectively. Relative expression values of microRNA-873-5p and CXCL5 were calculated in accordance with 2−ΔΔCt method. The test was repeated 3 times.
Table 1. Primers for qRT-PCR.
Western blot
Radioimmunoprecipitation assay lysis buffer (Thermo Fisher Scientific, USA) was used for total protein extraction from cells, and the bicinchoninic acid protein assay kit (Thermo Fisher Scientific, USA) was applied to quantify the protein concentrations. The proteins were then isolated by SDS-PAGE and blotted onto a PVDF membrane (Bio-Rad, USA). After blocking with 5% skimmed milk for 1 h, the membrane was subjected to co-incubation with primary antibodies first overnight at 4 °C and secondary antibody then at room temperature for 1 h. Afterward, the protein bands were visualized using supersensitive enhanced chemiluminescence kit (Solarbio, China) and Chemiluminescence imager (Bio-Rad, USA) and analyzed by Gel Image software. All antibodies were produced by Abcam. The primary antibodies were all rabbit antibodies: anti-CXCL5 (ab126763, UK), anti-P53 (ab32389, UK), anti-P-P53 (ab33889, UK), and anti-GAPDH (ab9485, UK). The secondary antibody we chose was goat anti-rabbit IgG H&L (HRP) (ab205718, UK).
CCK-8 assay
CCK-8 (Solarbio, China) was implemented for detecting cell proliferation. A 96-well plate was adopted to seed the cells (1 × 104 per well). After maintaining for 0 h, 12 h, 24 h, and 48 h, 10 μL CCK-8 solution was supplemented into each of the wells of the plate. Then, the plate was incubated at 37 °C with 5% CO2 for 2 h. The optical density value was read at 450 nm by a strip reader (Thermo Fisher Scientific, USA).
Colony formation assay
TPC-1 cells (1 × 103 cells/mL) were seeded into 6-well plates and cultivated in complete medium for 1–2 weeks, until visible colonies were formed. Subsequently, the plates were rinsed with 1×PBS twice, and the cells were fixed with 4% formaldehyde (Thermo Fisher, USA) for 15 min. After removing formaldehyde, the cells were colored with 0.25% crystal violet for 25 min. Pictures were photographed and the numbers of colonies in each well were calculated.
Wound healing assay
48 h later, the cells were planted into 6-well plates. When the cells reached 80% confluence, a 10 μL sterile pipette tip was applied to gently cross the center of the well. After rinsing with PBS, the cells were cultured for another 24 h. Cell photographs were obtained by inverted microscope (Olympus, Japan). The wound width ratio of TC cells was calculated.
Transwell assay
TPC-1 cells (1 × 104) were seeded into the upper chamber precoated with Matrigel (BD, USA), while a medium containing 10% FBS (Thermo Fisher Scientific, USA) was filled into the lower chamber, followed by culture of 24 h. The cells that failed to invade were removed, and the invasive cells were treated with paraformaldehyde (4%) and then colored with crystal violet (0.1%). The samples were imaged under a microscope (Olympus, Japan) and analyzed with Image J software.
Dual-Luciferase assay
The wild type (WT) and the mutant (MUT) of 3’UTR fragment (200 bq upstream and downstream of the binding site) of CXCL5 cDNA were copied and inserted into the pmirGLO vector (Promega, USA). The restriction sites are PmeI and XbaI, which were constructed by chemical synthesis. By using of Lipofectamine™2000 (Invitrogen, USA), the pmirGLO-CXCL5-WT/pmirGLO-CXCL5-MUT and NC mimics/microRNA-873-5p mimics were used to treat the cells. After 24 h of culture, the cells were harvested. Luciferase activity assay kit (Promega, USA) was used to measure the fluorescence intensity of each transfection group.
Statistical analysis
All data was processed with GraphPad Prism 8.0 (La Jolla, USA). The results were shown in the form of mean ± standard deviation (SD). The inter-group differences were obtained by t-test or one-way ANOVA. P < 0.05 was considered significantly different. All assays were done in triplicate.
Results
CXCL5 is remarkably upregulated in TC
Bioinformatics methods were applicated to analyze the role of CXCL5 in TC, and the results told that CXCL5 expression was remarkably up-regulated in TC tissues (). The Kaplan-Meier analysis demonstrated that CXCL5 was a poor prognostic marker of TC (). Subsequently, qRT-PCR and western blot results demonstrated that compared to the normal cell line (Nthy-ori3–1), CXCL5 expression level was significantly increased in all TC cells (KTC-1, TPC-1, BCPAP, K1, BHP10–3), especially in TPC-1 cell line. Thus, we chose TPC-1 cell line for subsequent analyses ().
Figure 1. CXCL5 expression in TC. (a) CXCL5 expression in TC tissues. the blue and pink box plots represent the normal samples and the tumor samples, respectively; (b) the effects of CXCL5 on the prognosis of TC. (c) mRNA expressions of CXCL5 in normal cell line (Nthy-ori3–1) and TC cell lines (KTC-1, TPC-1, BHP10–3, K1, BCPAP); (d) protein expressions of CXCL5 in different cell lines (*p < .05).
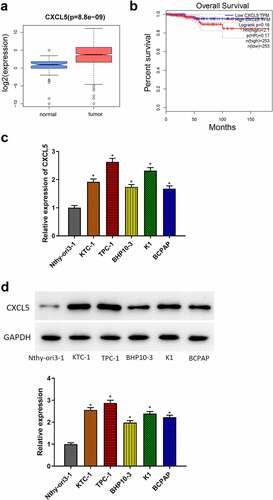
CXCL5 promotes proliferation, invasion, migration of TC cells
To verify the influence of CXCL5 on the biological function of TC cells, si-CXCL5 was used to transfect the TPC-1 cells. The transfection results were verified by qRT-PCR. The result of qRT-PCR showed that CXCL5 was remarkably repressed by si-CXCL5 in TPC-1 cells (). CCK-8 assay and colony formation assay results indicated that the CXCL5 silencing considerably repressed the growth of TPC-1 cells (). Cell scratch and Transwell invasion assays revealed that the migratory and invasive abilities of cancer cells in si-CXCL5 group were suppressed than those in the control groups (). These indicated that CXCL5 silencing suppressed the malignant behaviors of TC cells.
CXCL5 is a direct target of microRNA-873-5p
To clarify the mechanisms of CXCL5 regulating TC progression, we applied mirDIP and starBase databases to identify the upstream genes of CXCL5. By intersecting predicted results with the 10 down-regulated differential microRNAs, we obtained one differential microRNA with binding site to CXCL5, namely microRNA-873-5p (). Pearson correlation analysis found that microRNA-873-5p had a significant negative correlation with CXCL5 (). It was noted that microRNA-873-5p was significantly under-expressed in TC tissue samples (). Next, qRT-PCR results suggested that microRNA-873-5p expression level was notably decreased in TC cell lines (). Both bioinformatics prediction and experimental results showed that microRNA-873-5p was underexpressed in TC. Based on the above results, we identified microRNA-873-5p as an upstream gene of CXCL5. Also, the relationship between microRNA-873-5p expression and clinical data was investigated, revealing that the expression of microRNA-873-5p was associated with TC patients’ clinical stage (see Appendix ).
Figure 3. CXCL5 is a direct target of microRNA-873-5p. (a) Venn diagram of predicted microRnas and down-regulated differential microRnas; (b) Pearson correlation analysis of microRNA-873-5p and CXCL5; (c) microRNA-873-5p expression in TC tissues. The blue and pink box represent the normal samples and the tumor samples, respectively; (d) MicroRNA-873-5p expression in TC cells; (e) Targetscan predicts upstream regulatory genes of CXCL5; (f) Verification of the binding of microRNA-873-5p with CXCL5; (g) the expression level of CXCL5 mRNA in cells with microRNA-873-5p overexpression; (h) the expression level of CXCL5 protein in cells with microRNA-873-5p overexpression (*p < .05).
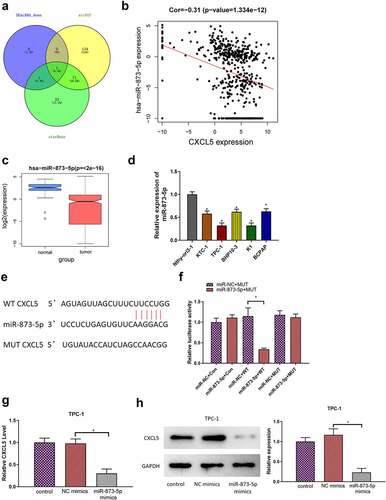
Next, we further investigated the molecular regulatory relationship between microRNA-873-5p and CXCL5. Firstly, the binding sequence between microRNA-873-5p and CXCL5 3’UTR were predicted through bioinformatics database (). Secondly, luciferase assay results demonstrated that microRNA-873-5p overexpression markedly reduced the relative luciferase activity of CXCL5-WT but not the mutant reporter (). This result indicated that CXCL5 was a direct target of microRNA-873-5p. Then, to validate the interaction between microRNA-873-5p and CXCL5, we transfected microRNA-873-5p mimics into TPC-1 cell lines. qRT-PCR and Western blot results demonstrated that the expression of CXCL5 mRNA and protein was significantly decreased after overexpression microRNA-873-5p in TPC-1 cells (). Taken together, the above results suggested that CXCL5 was a direct target of microRNA-873-5p and was negatively modulated by microRNA-873-5p.
MicroRNA-873-5p mediates p53 pathway and thereby inhibits cell proliferation, invasion, migration in TC by targeting CXCL5
We had verified that down-regulation of CXCL5 could inhibit the malignant behaviors of TC cells and microRNA-873-5p was an upstream regulatory gene of CXCL5. To authenticate the effects of microRNA-873-5p/CXCL5 interaction on the malignant phenotypes of TC cells, we divided the cells into four treatment groups: control group, NC mimics (NC mimics+pcDNA3.1-NC) group, microRNA-873-5p mimics (microRNA-873-5p mimics+pcDNA3.1-NC) group and microRNA-873-5p mimics with pcDNA3.1-CXCL5 (microRNA-873-5p mimics+pcDNA3.1-CXCL5) group, and detected the mRNA expression level of CXCL5 by qRT-PCR (). Next, CCK-8 and colony formation results demonstrated that the proliferation of TC cells was inhibited in the microRNA-873-5p mimics group, but this inhibitory effect was restored when TC cells were co-transfected with microRNA-873-5p mimics and pcDNA3.1-CXCL5(). Wound healing assay and Transwell assay told that the migration and invasion of TC cells were significantly reduced in the group transfected with microRNA-873-5p mimics compared to in the NC mimics group, but the co-transfection of pcDNA3.1-CXCL5 could reverse the suppressive effect of microRNA-873-5p overexpression on the migration/invasion abilities of cancer cells (). In order to further explore the downstream regulatory pathway, GSEA was performed for patients with high- and low-expression of CXCL5, and the results revealed that the differential genes were significantly enriched in the P53 signaling pathway (). The effects of microRNA-873-5p and CXCL5 on P53 pathway-related proteins were investigated by Western blot, which demonstrated that compared to the NC mimics group, the microRNA-873-5p mimics group had remarkably enhanced phosphorylation expression of P53 and remarkably reduced expression of CXCL5. However, in the microRNA-873-5p mimics+pcDNA3.1-CXCL5 group, CXCL5 overexpression eliminated the effects of microRNA-873-5p overexpression on P53 phosphorylation. These results suggested that microRNA-873-5p targeted CXCL5 to facilitate the P53 signaling pathway in TC cells (). All the above results proved that microRNA-873-5p overexpression suppressed the malignant behaviors of TC cells, while CXCL5 overexpression overturned this inhibitory effect of microRNA-873-5p.
Figure 4. MicroRNA-873-5p mediates p53 pathway by targeting CXCL5 to inhibit cell proliferation, migration, invasion in TC. (a) The transfection efficiency of each group; (b) Cell viability in each group; (c) Cell proliferation in each group; (d) Cell migratory ability in each group; (e) Cell invasive ability in each group; (f) GSEA of CXCL5; (g) the expression levels of CXCL5 protein and P53-related pathway proteins (*p < .05).
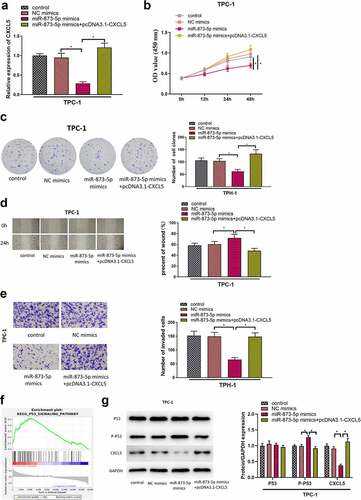
Discussion
The experimental results of this study uncovered that CXCL5 was a downstream target gene of microRNA-873-5p and was related to the malignant progression of TC. In TC, overexpression of CXCL5 promoted cancer proliferation, invasion, and migration. Therefore, CXCL5 may play a carcinogenic role in TC progression.
Previous evidence has demonstrated that CXCL5 is an important chemokine in TME and is highly expressed in various cancers. In tumor progression, CXCL5 recruits immune cells through binding to its receptor CXCR2 and thus promotes tumor growth, invasion, metastasis, and tumor angiogenesis.Citation6 For example, the release of CXCL5 facilitates the malignant phenotypes of pancreatic cancer cells,Citation10 and CXCL5 induces tumor angiogenesis in colorectal cancer by enhancing the FOXD1 expression mediated by the AKT/NF-KB pathway.Citation11 The present study revealed that CXCL5 was markedly overexpressed in TC cells, and the cell function experiment proved that CXCL5 promoted the malignant behaviors of TC cells.
Besides, studies have shown that the role of CXCL5 in cancer is regulated by upstream microRNAs. For example, in colorectal cancer, microRNA-432-5p represses cancer cell invasion, and migration by targeting CXCL5,Citation12 and CXCL5 contributes to carcinogenicity and subjects to microRNA-577 post-transcriptional regulation in cervical cancer.Citation6 We speculated that the influence of CXCL5 in TC was also regulated by its upstream microRNA. Through bioinformatics analysis, we found that CXCL5 had an upstream regulatory molecule, microRNA-873-5p. Studies have shown that microRNA-873-5p is under-expressed in TC cells and can suppress the malignant progression of TC by modulating expression of target genes. For example, microRNA-873-5p restrains the malignant phenotypes of tumor cells by regulating CXCL16 in TC.Citation13 To further verify the role of microRNA-873-5p targeting CXCL5 in TC, the present study performed further cell function experiments and verified that microRNA-873-5p could inhibit the malignant behaviors of TC cells through down-regulating CXCL5. To conclude, the present study found that microRNA-873-5p/CXCL5 regulatory axis could affect the malignant behaviors of TC cells in vitro.
P53 is a tumor suppressor protein that involves cell growth inhibition, cell apoptosis, and cell cycle progression, and it is currently found to be the most associated gene with tumor.Citation14 However, in tumor cells, mutated P53 can facilitate the malignant behaviors of cancer cells and participate in cancer metabolism in vivo.Citation15 Previous studies have shown that P53 mutation induces ONZIN up-regulation and promotes cancer cell metastasis in osteosarcoma through CXCL5-MAPK signaling pathway.Citation11 In the present study, the effects of microRNA-873-5p and CXCL5 on the expression of P53 signaling pathway-related proteins were investigated. Our experiments found that the overexpressed microRNA-873-5p evidently lifted the phosphorylation level of P53 in TC cells, while CXCL5 overturned the effect of microRNA-873-5p on P53 phosphorylation. Viewed in total, microRNA-873-5p targeted CXCL5 to promote the P53 signaling pathway in TC, so as to hamper the malignant behaviors of cancer cells.
In conclusion, the present study demonstrated that microRNA‑873-5p was underexpressed in TC and inhibited the malignant behaviors of TC cells. Further mechanism study showed that microRNA-873-5p affected the behaviors of TC cells by suppressing expression of CXCL5 and regulating P53 signaling. These findings may provide useful theoretical evidence for understanding TC pathogenesis and finding a new target for TC treatment.
Authors’ contributions
Conceptualization: Wang Chang, Qing Chang
Data curation: Wang Chang, Haodong Lu, Shiguang Liu
Formal analysis: Yanbing Li, Chunyou Chen
Methodology: Qing Chang, Haodong Lu, Shiguang Liu
Software: Yanbing Li, Chunyou Chen
Validation: Wang Chang, Qing Chang, Yanbing Li
Investigation: Shiguang Liu, Haodong Lu
Writing—original draft: Wang Chang, Yanbing Li, Shiguang Liu
Writing—review and editing: Chunyou Chen, Haodong Lu
All the authors read and approved the final manuscript.
Ethics approval and consent to participate
Our study did not require an ethical board approval because it did not contain human or animal trials.
Disclosure statement
No potential conflict of interest was reported by the author(s).
Data availability statement
All data generated or analyzed during this study are included in this published article.
Additional information
Funding
References
- Arneth B. Tumor microenvironment. Medicina (Kaunas). 2019;56(1). doi:10.3390/medicina56010015.
- Zhang W, Wang H, Sun M, Deng X, Wu X, Ma Y, Li M, Shuoa SM, You Q, Miao L, et al. CXCL5/CXCR2 axis in tumor microenvironment as potential diagnostic biomarker and therapeutic target. Cancer Commun (Lond). 2020;40(2–3):69–9. doi:10.1002/cac2.12010.
- Chow MT, Luster AD. Chemokines in cancer. Cancer Immunol Res. 2014;2(12):1125–31. doi:10.1158/2326-6066.CIR-14-0160.
- Zhao J, Ou B, Ham D, Wang P, Zong Y, Zhu C, Liu D, Zheng M, Sun J, Feng H, et al. Tumor-derived CXCL5 promotes human colorectal cancer metastasis through activation of the ERK/Elk-1/snail and AKT/GSK3beta/beta-catenin pathways. Mol Cancer. 2017;16(70). doi:10.1186/s12943-017-0629-4.
- Cui D, Zhao Y, Xu J. Activation of CXCL5-CXCR2 axis promotes proliferation and accelerates G1 to S phase transition of papillary thyroid carcinoma cells and activates JNK and p38 pathways. Cancer Biol Ther. 2019;20(5):608–16. doi:10.1080/15384047.2018.1539289.
- Bai L, Yao N, Qiao G, Wu L, Ma X. CXCL5 contributes to the tumorigenicity of cervical cancer and is post-transcriptionally regulated by miR-577. Int J Clin Exp Pathol. 2020;13:2984–93.
- Dong G, Zhang R, Xu J, Guo Y. Association between microRNA polymorphisms and papillary thyroid cancer susceptibility. Int J Clin Exp Pathol. 2015;8:13450–57.
- Han J, Zhang M, Nie C, Jia J, Wang F, Yu J, Bi W, Liu B, Sheng R, He G, et al. miR-215 suppresses papillary thyroid cancer proliferation, migration, and invasion through the AKT/GSK-3β/Snail signaling by targeting ARFGEF1. Cell Death Dis. 2019;10(3). doi:10.1038/s41419-019-1444-1.
- Zhu Y, Zhang X, Qi M, Zhang Y, Ding F. miR-873-5p inhibits the progression of colon cancer via repression of tumor suppressor candidate 3/AKT signaling. J Gastroenterol Hepatol. 2019;34(12):2126–34. doi:10.1111/jgh.14697.
- Ando Y, Ohuchida K, Otsubo Y, Kibe S, Takesue S, Abe T, Iwamoto C, Shindo K, Moriyama T, Nakata K, et al. Necroptosis in pancreatic cancer promotes cancer cell migration and invasion by release of CXCL5. PLoS One. 2020;15(1):e0228015. doi:10.1371/journal.pone.0228015.
- Zhang Y, Hu Q, Li G, Li L, Liang S, Zhang Y, Liu J, Fan Z, Li L, Zhou B, et al. ONZIN upregulation by mutant p53 contributes to osteosarcoma metastasis through the CXCL5-MAPK signaling pathway. Cell Physiol Biochem. 2018;48(3):1099–111. doi:10.1159/000491976.
- Luo M, Hu Z, Kong Y, Li L. MicroRna‑432‑5p inhibits cell migration and invasion by targeting CXCL5 in colorectal cancer. Exp Ther Med. 2021;21(4). doi:10.3892/etm.2021.9732.
- Wang Z, Liu W, Wang C, Ai Z. miR-873-5p. Inhibits cell migration and invasion of papillary thyroid cancer via regulation of CXCL16. Onco Targets Ther. 2020;13:1037–46. doi:10.2147/OTT.S213168.
- Mantovani F, Collavin L, Del Sal G. Mutant p53 as a guardian of the cancer cell. Cell Death Differ. 2019;26(2):199–212. doi:10.1038/s41418-018-0246-9.
- Zhou X, Hao Q, Lu H. Mutant p53 in cancer therapy—the barrier or the path. J Mol Cell Biol. 2019;11(4):293–305. doi:10.1093/jmcb/mjy072.