Development of a Covid-19 vaccine: a head start
Professor Sir Andrew Pollard shared the currently available Phase I–III data for the Oxford coronavirus vaccine (ChAdOx1 nCoV-19) and provided a comprehensive description of the coronavirus vaccine types currently in development.
Mortality predictions at the beginning of the Covid-19 pandemic were extremely concerning, with a prediction of up to 510,000 deaths in the UK alone in the absence of any restrictions. Early on, the increased risk of severe disease/death in older individuals and those with underlying health conditions was established. Importantly, vaccine developers had a solid footing to allow a rapid response to the Covid-19 pandemic in terms of developing an effective vaccine. Firstly, the severe acute respiratory syndrome coronavirus 2 (SARS-CoV-2) genome sequence was available rapidly following the emergence of the virus. Secondly, there was already a wealth of information on the biology of the virus due to two previous coronavirus outbreaks, the severe acute respiratory syndrome (SARS) epidemic in 2002–3 and the Middle East respiratory syndrome (MERS) outbreak, which began in 2012. The SARS outbreak could be controlled using case isolation, as cases were severe enough to be easily detected. Furthermore, the transmissibility of the virus was not particularly good. Similarly, the MERS outbreak was controlled due to its lack of transmissibility from person to person. As such, there had already been work on coronavirus vaccine development, and a Phase I trial had been conducted with a MERS coronavirus vaccine.Citation1
This prior knowledge allowed the vaccine development process to be expedited. Firstly, animal studies began earlier than usual because initial work on vaccine design had been conducted before the pandemic. Secondly, manufacturing was initiated at the same time as animal studies. The phases of the trials were also rapidly escalated, with very close monitoring by data safety monitoring committees. Furthermore, manufacturing was upscaled in parallel with the earliest phases of the trials, with substantial financial risk. Finally, there were rolling reviews of data as they became available so that the vaccines could be licensed with due haste once final datasets were available.
The Oxford vaccine (ChAdOx1 nCoV-19)
The Oxford vaccine (ChAdOx1 nCoV-19) is a viral vector vaccine consisting of the replication-deficient simian adenovirus vector ChadOx1, containing the full-length structural surface glycoprotein (spike protein) of SARS-CoV-2, with a plasminogen activator leader sequence.
Phase I
Phase I studies to evaluate the safety and immunogenicity of the Oxford vaccine began in April 2020 (). A large number of participants were recruited to allow timely evaluation of efficacy. However, the reduction in cases due to social distancing measures and stay-at-home restrictions made evaluating efficacy difficult. The trial had to be conducted in line with COVID restrictions, and there was a global lack of access to personal protective equipment and medical supplies.
Table 1. Phase I–III trials with the Oxford vaccine (ChAdOx1 nCoV-19).
Reactogenicity during Phase I trials was as expected, with mostly mild or moderate localized injection site reaction and flu-like symptoms that could be mitigated by paracetamol.Citation2 A single dose of ChAdOx1 nCoV-19 elicited an increase in spike-specific antibodies, which peaked by day 28 and remained elevated until day 56. Neutralizing antibodies increased further in all participants who had a second dose.Citation2, Citation3 While a one-dose schedule was a viable option based on these data, the enhanced antibody response with a second dose supported the two-dose schedule used for the Phase II trial. The other important finding during the Phase I trial was the generation of T cells against SARS-CoV2 spike peptides after the first dose, which peaked at day 14, and remained above baseline to day 56. As expected with a viral vector vaccine in a homologous prime-boost regimen, there was no boost in T cell response following the second vaccine dose; however, the increase above baseline persisted.Citation2
Phase II
The Phase II trial began in May 2020, recruiting volunteers aged 56–69 years and those aged >70 years (). Data from this trial were essential in demonstrating immune responses in older adults, similar to those seen in younger adults.
Phase III
Phase III studies were conducted in the UK, Brazil, South Africa (), with a small additional study in Kenya. During these trials, coronavirus cases were identified in 3 ways: through PCR tests conducted in symptomatic patients; via serum samples to determine evidence of infection (exploratory); and using weekly swabs for all study participants to identify asymptomatic infections. Phase III studies were also conducted, with AstraZeneca, in the US, Japan, Russia, and by Serum Institute of India, in India.
Amid a great deal of media attention, Phase III results were provided in a press release showing 70% overall efficacy; 90% in one dosing regimen and 62% in a second. The regimen associated with 90% efficacy had the most favorable tolerability profile. Importantly, there were no hospitalized or severe Covid-19 cases in anyone who received the vaccine (efficacy analysis cohort). Overall, the trials produced an equivalent of >6000 person-years of safety follow up data.
Other coronavirus vaccines in clinical development
At the time of the presentation, there were 47 vaccines in clinical development and 11 in Phase III trials, providing great potential for effective vaccines in the near future to bring the pandemic under control.Citation4 Based on pre-clinical data, it is clear that anti-spike protein neutralizing antibody correlates with protection against Covid-19 infection.Citation5 Furthermore, it is becoming clear that relatively low levels of immunity are required to protect against lower respiratory tract infections, providing hope that these vaccines might prevent severe disease and hospitalization, as was subsequently shown in the real world effectiveness studies after roll out. Several different vaccine types are in development, as described below.
Inactivated vaccines
Several inactivated vaccines are being developed. One advantage of these vaccines is the wealth of experience with the traditional chemical inactivation techniques used during development. Notably, the neutralizing antibody responses are produced with inactivated vaccines. For example, in a Phase I/II trial of the inactivated SARS-CoV-2 vaccine BBIBP-CorV, neutralizing antibodies against infectious SARS-CoV-2 were detected in 18% of vaccine recipients after the first dose and 100% of recipients on day 42 after the second dose.Citation6
Protein subunit vaccines
Protein subunit vaccines, in which a recombinant protein is mixed with an adjuvant, are also in development. For example, 15,000 volunteers in the UK have been vaccinated with NVX-CoV2373, a recombinant SARS-CoV-2 nanoparticle vaccine composed of trimeric full-length SARS-CoV-2 spike glycoproteins and Matrix-M1 adjuvant. Early studies with this vaccine showed good antibody responses at several different dose levels, showing high levels of neutralizing antibodies after the second dose.Citation7
RNA vaccines
The development of RNA vaccines, in which the spike protein RNA is synthesized and then encapsulated in a lipid nanoparticle, is an exciting development for vaccine technology. There are two main examples, developed by Moderna and Pfizer,Citation8 which had recently released their Phase III results at the time of the presentation. An RNA vaccine was also in development at Imperial College London. With the Moderna vaccine, rising neutralizing antibodies were shown with the two doses tested.Citation9 The trial included 30,000 participants and demonstrated high efficacy (as presented in a recent press release). With the Pfizer vaccine, strong neutralizing responses were shown after 3 µg and 10 µg doses.Citation10 Higher doses were not taken forward due to poor tolerability. The trial included 3000 people, and at the time of the presentation data showing high protective efficacy had also recently been released.
DNA vaccines
DNA coronavirus vaccines, consisting of plasmid DNA containing spike protein DNA,Citation11 are also in development, although there is currently less information about this vaccine type than those previously described. Assisted uptake is required using, for example, electroporation or hypertonic saline. At the time of the presentation, INOVIO was investigating a novel, Covid-19 DNA vaccine.
Viral vector vaccines
Several viral vector vaccines are also being investigated, including ChAdOx1 nCoV-19 as previously described, as well as vaccines developed by Janssen and Cansino, and one being developed in Russia. In China, safety, tolerability and immunogenicity of the recombinant adenovirus type-5 (Ad5) vectored Covid-19 vaccine expressing the SARS-CoV-2 spike protein was evaluated in a Phase I trial. With the high dose tested, 75% of participants had a 4-fold rise in neutralizing antibodies after vaccination.Citation12 Russia assessed a heterologous Covid-19 vaccine comprising a recombinant adenovirus type-26 (rAd26) vector and a recombinant Ad5 vector carrying the gene for SARS-CoV-2 spike glycoprotein in two Phase I/II studies. Strong humoral and cellular immune responses were induced in vaccinated participants.Citation13
Summary
In summary, multiple vaccine types are being developed to protect against Covid-19 disease using different technologies. All appear to induce neutralizing antibodies, and some have also published data showing T cell responses, which could be important in controlling established infection. Although the majority of these vaccines do induce some side effects, overall, they are well tolerated.
Declaration of interest
AJP is the chair of the Department of Health and Social Care’s Joint Committee on Vaccination and Immunization but does not participate in the JCVI Covid 19 committee. He is a member of the World Health Organization’s SAGE. The University of Oxford has entered into a partnership with Oxford University on the development of a Covid 19 vaccine.
Acknowledgments
AJP is supported by the NIHR Oxford Biomedical Research Center. Writing and editorial assistance provided by the National Immunization Conference.
References
- Folegatti PM, Bittaye M, Flaxman A, Lopez FR, Bellamy D, Kupke A, Mair C, Makinson R, Sheridan J, Rohde C, et al. Safety and immunogenicity of a candidate Middle East respiratory syndrome coronavirus viral-vectored vaccine: a dose-escalation, open-label, non-randomised, uncontrolled, phase 1 trial. Lancet Infect Dis. 2020;20(7):816–22. doi:10.1016/S1473-3099(20)30160-2.
- Folegatti PM, Ewer KJ, Aley PK, Angus B, Becker S, Belij-Rammerstorfer S, Bellamy D, Bibi S, Bittaye M, Clutterbuck EA, et al.; Oxford COVID Vaccine Trial Group. Safety and immunogenicity of the ChAdOx1 nCoV-19 vaccine against SARS-CoV-2: a preliminary report of a phase 1/2, single-blind, randomised controlled trial. Lancet. 2020;396(10249):467–78. doi:10.1016/S0140-6736(20)31604-4.
- Ramasamy MN, Minassian AM, Ewer KJ, Flaxman AL, Folegatti PM, Owens DR, Voysey M, Aley PK, Angus B, Babbage G, et al.; Oxford COVID Vaccine Trial Group. Safety and immunogenicity of ChAdOx1 nCoV-19 vaccine administered in a prime-boost regimen in young and old adults (COV002): a single-blind, randomised, controlled, phase 2/3 trial. Lancet. 2020;396(10267):1979–93. doi:10.1016/S0140-6736(20)32466-1.
- World Health Organization. COVID-19 vaccine tracker and landscape 2021. [accessed 2021 Aug 31st]. https://www.who.int/publications/m/item/draft-landscape-of-covid-19-candidate-vaccines
- Yu J, Tostanoski LH, Peter L, Mercado NB, McMahan K, Mahrokhian SH, Nkolola JP, Liu J, Li Z, Chandrashekar A, et al. DNA vaccine protection against SARS-CoV-2 in rhesus macaques. Science. 2020;369(6505):806–11. doi:10.1126/science.abc6284.
- Xia S, Zhang Y, Wang Y, Wang H, Yang Y, Gao GF, Tan W, Wu G, Xu M, Lou Z, et al. Safety and immunogenicity of an inactivated SARS-CoV-2 vaccine, BBIBP-CorV: a randomised, double-blind, placebo-controlled, phase 1/2 trial. Lancet Infect Dis. 2021;21(1):39–51. doi:10.1016/S1473-3099(20)30831-8.
- Keech C, Albert G, Cho I, Robertson A, Reed P, Neal S, Plested JS, Zhu M, Cloney-Clark S, Zhou H, et al. Phase 1-2 trial of a SARS-CoV-2 recombinant spike protein nanoparticle vaccine. N Engl J Med. 2020;383(24):2320–32. doi:10.1056/NEJMoa2026920.
- Jackson NAC, Kester KE, Casimiro D, Gurunathan S, DeRosa F. The promise of mRNA vaccines: a biotech and industrial perspective. NPJ Vaccines. 2020;5(1):11. doi:10.1038/s41541-020-0159-8.
- Jackson LA, Anderson EJ, Rouphael NG, Roberts PC, Makhene M, Coler RN, McCullough MP, Chappell JD, Denison MR, Stevens LJ, et al.; mRNA-1273 Study Group. An mRNA Vaccine against SARS-CoV-2 - preliminary report. N Engl J Med. 2020;383(20):1920–31. doi:10.1056/NEJMoa2022483.
- Mulligan MJ, Lyke KE, Kitchin N, Absalon J, Gurtman A, Lockhart S, Neuzil K, Raabe V, Bailey R, Swanson KA, et al. Phase I/II study of COVID-19 RNA vaccine BNT162b1 in adults. Nature. 2020;586(7830):589–93. doi:10.1038/s41586-020-2639-4.
- McDonnell WM, Askari FK. DNA vaccines. N Engl J Med. 1996;334(1):42–45. doi:10.1056/NEJM199601043340110.
- Zhu FC, Li Y-H, Guan X-H, Hou L-H, Wang W-J, Li J-X, Wu S-P, Wang B-S, Wang Z, Wang L, et al. Safety, tolerability, and immunogenicity of a recombinant adenovirus type-5 vectored COVID-19 vaccine: a dose-escalation, open-label, non-randomised, first-in-human trial. Lancet. 2020;395(10240):1845–54. doi:10.1016/S0140-6736(20)31208-3.
- Logunov DY, Dolzhikova IV, Zubkova OV, Tukhvatulin AI, Shcheblyakov DV, Dzharullaeva AS, Grousova DM, Erokhova AS, Kovyrshina AV, Botikov AG, et al. Safety and immunogenicity of an rAd26 and rAd5 vector-based heterologous prime-boost COVID-19 vaccine in two formulations: two open, non-randomised phase 1/2 studies from Russia. Lancet. 2020;396(10255):887–97. doi:10.1016/S0140-6736(20)31866-3.
Beyond masks, social distancing, and handwashing, COVID-19 vaccines are a critical element in the public health COVID-19 pandemic control strategy.Citation1 However, COVID-19 vaccine acceptance is influenced by many contextual factors that are even more complex than for traditional vaccines, even for those as experienced with the pandemic influenza A(H1N1) vaccination campaigns.Citation2 There are high expectations: high visibility of COVID-19, a multitude of COVID-19 vaccines with differing platforms and efficacy rates – not just a single vaccine, accelerated development and approval, equity access concerns both within countries and across the globe, new target populations – across the age spans, risk of potential serious adverse events following immunization as are new vaccines, anti-vaccine activism and concerns about vaccine effectiveness in the face of COVID-19 mutations.Citation3 These have all generated much anxiety and stress given how widely the pandemic has impacted health, well-being, and the economy – globally, locally, and personally.
The infodemic (i.e., an overabundance of information, some accurate and some not, both online and offline) that has flourished around COVID-19 has added stress and confusion for the public, healthcare providers and policy makers.Citation4 In May 2020 the World Health Assembly passed a resolution concerning COVID-19 pandemic management which focused on addressing the infodemic.Citation5 This called on member states to provide reliable COVID-19 content, take measures to counter mis- and disinformation and leverage digital technologies across the response. The resolution also called on international organizations to address mis- and disinformation in the digital sphere, work to prevent harmful cyber activities undermining the health responses and support the provision of science-based data to the public. The December 2020 Vaccine Misinformation Management Field Guide developed by UNICEF emphasizes the difference between misinformation and information that is false but not created with the intention of causing harm, and disinformation and information that is false and deliberately created to cause harm.Citation6 The latter is created and disseminated for economic gain or intentionally to deceive the public for political gain, prestige or attention. More importantly misinformation and disinformation are “sticky,” traveling faster and farther than truth.Citation7 The Organization for Economic Co-operation and Development has emphasized four key actions that governments and platforms can take to counter COVID-19 disinformation: 1) support a multiplicity of independent fact-checking organizations; 2) ensure human moderators in place to complement technological solutions; 3) voluntarily issue transparency reports about COVID-19 disinformation and 4) improving users’ media, digital and health literacy skills.
Why do we fall prey to disinformation and misinformation?
Health decision making is complex, including the decisions to accept vaccines. In this regard, context matters, as does which vaccine.Citation8 Social, cultural, historical, and political factors influence how people feel and decide about vaccination. Furthermore, we are strongly influenced by what we think others around us are doing or expecting us to do, including social networks where disinformation is rife. We see causation in coincidences, and see what we believe, rather than believing what we see. We prefer anecdote and stories to data and evidence and pay more attention to negative information. This is why safety concerns and lack of trust may be especially prominent as the COVID pandemic provides an ideal context for the spread of mis- and disinformation and conspiracy theories, as they tend to arise in societal crisis situations that generate increased stress, sense of uncertainty, and increased concerns for the future.Citation9
The COVID-19 Vaccine Communication Handbook notes that to achieve high COVID-19 vaccine acceptance levels requires context-specific and data-driven behavioral interventions, working at multiple levels supported by targeted communications with messages and tools and techniques that help reach specific subgroups.Citation10 At the community level, effective communication from public health needs to be proactive about COVID-19 and COVID-19 vaccines, not just reactive, as well as paying attention to traditional media reports and social media to detect misinformation that needs to be corrected. Some only want a clear statement on what to do (the information bite) while others want more details (the information snack) and there are those who want links to the evidence (the information full lunch). For the latter, links to vetted evidence based credible websites such as those with a Vaccine Safety Net designation are helpful.Citation11 When crafting communications, remember the marketing principle that data tells but stories are more likely to sell. With the rapidly changing COVID-19 picture, linking to local context and known community leaders is key to enhancing credibility.
With respect to misinformation, do not fear that correction will lead to a backfire effect. More recent research has shown that the backfire effect is not as fixed as previously thought and that it is important to correct misinformation.Citation12 Furthermore, evidence from research shows that attitudinal resistance to misinformation can be conferred on the public and patients by preemptively highlighting false claims, refuting potential counterarguments and unmasking the common techniques being used (fake experts, conspiracies, selectivity, impossible expectations, logical fallacies and misrepresentation – lies).Citation13–15
The United Kingdom has supported development and access to the online browser game “Bad News”Citation16 which teaches players how to become disinformation creators, and in the process, strengthen their ability to detect and resist disinformation, i.e. avoid the con.Citation17 Education of children and adolescents to become critical thinkers, science literate and able to recognize misinformation and understand the critic al role vaccines do to support health and well-being is a major building block for ensuring the next generation of adults support immunization and vaccine programs. Kids Boost Immunity is a helpful web-based game developed in Canada, now also used in Ireland and Scotland, to help children learn about immunization, cognitive bias, correlation versus causation and the scientific method as well as about different vaccines including COVID-19 vaccines.Citation18
Given that healthcare professionals’ advice and recommendations on immunization are very influential in a patient’s and individual’s vaccine acceptance decision, it is critical that all healthcare professionals are well versed about the risks of the vaccine preventable disease and the safety and efficacy of the vaccines in order to appropriately advise their patients.Citation19 This is especially important for COVID-19 and COVID-19 vaccines given the infodemic currently raging. Healthcare professionals need to present these vaccines in a manner that shows their competence and caring to build trust, remembering that some only want a bite, others a snack, and the rest a full lunch of information. Clear language, positive framing and motivational interviewing have all been shown to be helpful. Alerting patients to the power of disinformation and teaching how to recognize the cons are key in this period of information overload. The World Health Organization’s useful infographic on top tips for navigating the infodemicCitation20 and the UK Bad News game21 previously mentioned are helpful tools to share with patients. Healthcare professionals also need to speak up in family and social gatherings if mis- and disinformation is being promulgated, as not speaking up could send the message that you as a healthcare professional agree with this false information. Finally, the attitudes of healthcare providers’ toward COVID-19 vaccines can also be negatively influenced by mis- or disinformation. Given their important role in building and maintaining vaccine acceptance in patients, tailored strategies to inform (bites, snacks, and full lunch) and address healthcare providers’ concerns are also critical.
Conclusions
The COVID-19 pandemic will continue for some time. Public health and healthcare professionals need to be prepared, anticipate needs and concerns, listen, update communication content and strategies as new data comes in. Beware of changing context and how this may affect how messages are heard. Remember that tailoring communication to fit the needs, concerns, level and amount of information needed – bites, snacks, and full lunch – can help combat the infodemic and lead to more COVID-19 science literacy and acceptance of COVID-19 vaccines.
Acknowledgments
This is based in part upon a presentation at UK National Immunization Conference in December 2020. Writing and editorial assistance provided by the National Immunization Conference.
Declaration of interest
None.CONTACT Noni E MacDonald [email protected]
References
- Lytras T, Tsiodras S. Lockdowns and the COVID-19 pandemic: what is the endgame? Scand J Public Health. 2021;49(1):37–40. doi:10.1177/1403494820961293.
- Poland CM, Matthews AKS, Poland GA. Improving COVID-19 vaccine acceptance: including insights from human decision- making under conditions of uncertainty and human-centred design. Vaccine. 2021;39(11):1547–50. doi:10.1016/j.vaccine.2021.02.008.
- Haidere MF, Ratan ZA, Nowroz S, Zaman SB, Jung Y-J, Hosseinzadeh H, Cho JY. COVID-19 Vaccine: critical Questions with Complicated Answers. Biomol Ther (Seoul). 2021;29(1):1–10. doi:10.4062/biomolther.2020.178.
- World Health Organization. An ad hoc WHO technical consultation managing the COVID-19 Infodemic: call for action. [Accessed 2021 Oct]. https://www.who.int/publications/i/item/9789240010314
- World Health Assembly. Report 73rd World Health Assembly Agenda item 3. COVID-19 response. 2020 May 19. [Accessed Oct 2021]. https://apps.who.int/gb/ebwha/pdf_files/WHA73/A73_R1-en.pdf
- UNICEF. Vaccine misinformation management field guide. [Accessed Oct 2021]. https://www.unicef.org/mena/media/10591/file/VACCINE+MISINFORMATION+FIELD+GUIDE_eng.pdf%20.pdf
- Scheufele DA, Krause NM. Science audiences, misinformation, and fake news. Proc Natl Acad Sci U S A. 2019;116(16):7662–69. doi:10.1073/pnas.1805871115.
- MacDonald NE; SAGE Working Group on Vaccine Hesitancy. Vaccine hesitancy: definition, scope and determinants. Vaccine. 2015;33(34):4161–64. doi:10.1016/j.vaccine.2015.04.036.
- van Prooijen JW, Douglas KM. Conspiracy theories as part of history: the role of societal crisis situations. Mem Stud. 2017;10(3):323–33. doi:10.1177/1750698017701615.
- Lewandowsky S, et al. The COVID-19 vaccine communication handbook. A practical guide for improving vaccine communication and fighting misinformation 2021. [Accessed 2021 Oct]. https://ohei.med.umich.edu/sites/default/files/files/downloads/FINAL%20COVID19VaccineHandbook%204%20production.pdf
- World Health Organization. Vaccine safety net. [Accessed 2021 Oct]. https://www.vaccinesafetynet.org/andhttps://www.vaccinesafetynet.org/vsn/network
- Lewandowsky S, et al. The Debunking Handbook 2020. [Accessed 2021 Oct]. https://www.climatechangecommunication.org/wp-content/uploads/2020/10/DebunkingHandbook2020.pdf
- van der Linden S, Maibach E, Cook J, Leiserowitz A, Lewandowsky S. Inoculating against misinformation. Science. 2017;358(6367):1141–42. doi:10.1126/science.aar4533.
- Schmid P, Betsch C. Effective strategies for rebutting science denialism in public discussions. Nat Hum Behav. 2019;3(9):931–39. doi:10.1038/s41562-019-0632-4.
- van der Linden S, Roozenbeek J, Compton J. Inoculating against fake news about COVID-19. Front Psychol. 2020;11:566790. doi:10.3389/fpsyg.2020.566790.
- Social Decision-making Lab, Cambridge University UK. Get bad news online game. [accessed 2021 Oct]. https://www.getbadnews.com/#introjuniorversion/https://www.getbadnews.com/wp-content/uploads/2019/03/Bad-News-Junior-info-sheet-for-educators-English.pdf
- Basol M, Roozenbeek J, Van der Linden S. Good news about bad news: gamified inoculation boosts confidence and cognitive immunity against fake news. J Cogn. 2020;3(1):2. doi:10.5334/joc.91.
- British Columbia Centre for Disease Control Kids Boost Immunity online game. [Accessed 2021 Oct]. https://kidsboostimmunity.com/
- MacDonald NE, Dubé E. Unpacking vaccine hesitancy among healthcare providers. EBioMedicine. 2015;2(8):792–93. doi:10.1016/j.ebiom.2015.06.028.
- World Health Organization. Let’s flatten the infodemic curve. [Accessed 2021 Oct]. https://www.who.int/news-room/spotlight/let-s-flatten-the-infodemic-curve
KEYWORDS:
Introduction
The introduction of new COVID-19 vaccines raises important issues regarding communication of vaccine safety and effectiveness. Of particular importance has been the need to gain public trust and confidence in the different COVID-19 vaccines and schedules to achieve high vaccine uptake and public health control. Vaccine hesitancy and safety concerns are interlinked and can negatively impact vaccine uptake. Effective communication around the large-scale vaccination programme requires consideration of the target groups and delivery of the message such that it appeals to the relevant groups. Other important considerations are the tools used to support the message and the selection of the most appropriate messengers to convey the message. The Bite, Snack, Lunch model is proposed as a multi-layer, flexible vaccine communication framework that can be modified to the specific needs of target groups.
Approach to introducing new vaccines
The worldwide introduction of any new vaccine will inevitably raise important issues, particularly concerning vaccine safety and effectiveness. Gaining public trust and confidence in the different COVID-19 vaccines is essential to achieve high vaccine uptake. Based on experience and evidence, proven methods of communication regarding the safety and efficacy of vaccines should be utilized. Furthermore, preparedness to respond rapidly to any vaccine safety issues or scares that arise is prudent. In the context of COVID-19, a high level of vaccine hesitancy was expected based on the vaccines being new and their development being fast-tracked.
High levels of vaccine hesitancy can lead to individuals hesitating to participate in available vaccination programmes. The three key factors contributing to vaccine hesitancy, the delay in acceptance or refusal of vaccination despite its availability, are defined as complacency, lack of confidence and inconvenience.21,Citation1 Complacency occurs when the risk of disease is seen as low, and vaccination is seen as unnecessary or if other health issues prevail. Lack of confidence can be due to the vaccines not being perceived to be safe or effective, there is a lack of trust in policymakers, or there is a lack of trust in health systems to deliver a vaccine. Inconvenience can be a significant barrier to vaccination whereby it be physical or geographical availability, affordability or health and language literacy.21,Citation1
In addition to these factors, the interlinked relationship between vaccine hesitancy and vaccine safety scares must also be considered. Vaccine safety scares, situations where unwanted events which may or may not be attributable to the vaccine create feelings of distrust and insecurity in the vaccine, can increase levels of vaccine hesitancy, and vice versa.Citation2 Thus, to plan effective communication around a large-scale vaccination programme, health authorities need to consider their target groups and understand the respective barriers and motivators to vaccination.
The ‘Bite, Snack, Lunch’ communication model
A practical model has been proposed by MacDonald et al. (2021)Citation3 with which to communicate information regarding vaccines. The ‘Bite, Snack, Lunch’ model is designed to be a flexible communication framework that can be used to convey important messages. The bite, snack and ‘meal’ concept was originally devised as a content writing and editing strategy and was first put forth by Leslie O’Flahavan in 1997 during her web writing courses, but later popularized in the 2000s.Citation4,Citation5
The Bite is the headline message, which should be brief and simple to understand. It is important as for some people, this will be the only message considered when deciding whether to be vaccinated or not. The Snack is a more detailed message containing key information surrounding the rationale for a public policy decision. This level of information may be sufficient for people with confidence in the healthcare system and/or political system to proceed with vaccination. Lunch is the comprehensive message, which will include all the scientific evidence for a public policy decision. Some people will require this level of information before proceeding with vaccination. In addition to the scientific information, “lunch” may include detailed religious or cultural information that may be necessary for some people to bolster their confidence in a COVID-19 vaccine and accept vaccination.
This model is regarded as a method of chunking content for the web, the bite, snack and meal approach entails writing for the appetite of a variety of customers. Some will nibble at content, some will spend more substantial time with it, while others will spend more time with the information to get the most possible out of the content.
The model requires careful consideration of the target audience, the right and most appropriate message to be delivered, supplementary tools, all delivered by the most effective messenger.Citation1,Citation5 Target audiences should be defined so that messages can be delivered in a way that both appeal to the audience and are well understood. Supplementary tools can be used to enhance the communicated message. These may include written information, pictures, cartoons, radio, written media, television and/or social media. Consideration should be given to identifying the person best equipped to deliver the Bite, Snack or Lunch most effectively. The messenger may be the vaccinator, a trusted scientist or health professional, or a trusted community leader relevant to the community and/or key stakeholders.
Conclusion
COVID-19 vaccines have been subject to an unprecedented level of scrutiny from the scientific community and from public opinion around the world. This is to be welcomed as it provides the opportunity to communicate globally their impressive safety and efficacy data and hence encourage high vaccine uptake. However effective communication strategies must continually adapt to deal with vaccine safety issues as they inevitably arise and to counter misinformation and disinformation. During an everchanging COVID-19 infodemic, the Bite, Snack, Lunch model provides a simple and consistent framework on which to plan effective communication.
The Bite, Snack, Lunch model is a flexible vaccine communication tool, adaptable to the specific needs of the target group of patients. It provides a practical framework for communication advisors, vaccine programme managers and vaccinators to use in their daily practice. Useful resources to help in the preparation of messages for the delivery of a vaccine programme are provided in .
Table 2. Useful resources to facilitate vaccine programme communications.
Acknowledgments
Professor Noni MacDonald for giving a workshop on vaccine communication at the National Immunization Conference UK, 2020. Writing and editorial assistance provided by the National Immunization Conference.Declaration of interest
None.
References
- World Health Organization. Vaccination and trust: how concerns arise and the role of communication in mitigating crises. 2017. [ accessed 2021 Nov]. https://www.euro.who.int/__data/assets/pdf_file/0004/329647/Vaccines-and-trust.PDF
- MacDonald NE. 2021. Bites, snacks and lunch communications for COVID-19 and COVID vaccines. Hum Vaccin Immunother. ( submitted).
- Inc Magazine. The Bite, the Snack and the Meal. 2001. [ accessed 2021 Nov]. https://www.inc.com/articles/2001/06/23143.html
- Consultant Journal. Bite, snack and meal- original reference. [ accessed 2021 Nov]. https://consultantjournal.com/blog/bite-snack-and-meal-original-reference
- MacDonald NE, Law BJ. Canada’s eight-component vaccine safety system: a primer for health care workers. Paediatr Child Health. 2017;22(4):e13–e16. doi:10.1093/pch/pxx073.
KEYWORDS:
Introduction
Stockport is a unitary authority sited in the south of Greater Manchester that has an ethnically diverse population of nearly 300,000 people. A small research team based in the 775-bedded Stockport District General Hospital has been active in immunization studies and infectious diseases surveillance for over two decades. During the last decade, this team has performed several studies including a pilot study to implement a schools-based Human Papillomavirus vaccine programme prior to its national introduction, two meningococcal carriage studies, two Phase III vaccine studies, one involving a meningococcal B vaccine and one involving a Clostridium difficile vaccine, and two surveillance studies on meningococcal infection. This experience, together with a workforce that has a relatively low staff turnover rate, has resulted in an efficient and effective workforce that is skilled, confident, and able to deliver vaccination clinical studies safely. This report describes the team’s experiences during the delivery of a UK-based, Phase III, placebo-controlled, double-blind, multisite, coronavirus (Covid-19) recombinant protein vaccine study in 2020.
Preparing for the study
Staffing
Prior to seeing the first patient, organizing a clinical study is complex. Contracts, procedures, consumables, training, and staffing must all be considered. As the team in Stockport has only a small number of permanent staff, staffing the Covid-19 vaccine study during a pandemic was a challenge. To deliver the study safely, adhering to protocol requirements, a substantial number of staff of all disciplines was required. To achieve this, staff from neighboring trusts were invited to be involved. This alone created a considerable volume of administrative work in the form of checking staff employment contracts and ensuring that all staff had a letter of access to work under Stockport’s policies and procedures. Moreover, all staff involved in the study required appropriate training, and the correct roles had to be delegated. With over 150 staff at times during the study, this was a time-consuming process. Furthermore, the ongoing pandemic made managing and sourcing staff for the study more time-consuming than usual as availability often changed with little notice. In addition to the frontline staffing, a substantial number of administrative staff were also needed to input the study data in a timely manner and to manage contact with GP surgeries and reimbursement of travel expenses for study participants.
Venue
The team also had to decide upon and find an appropriate venue for the study. It was decided that the study should be held outside of the hospital to avoid bringing study participants into an environment that may increase their risk of encountering coronavirus. Using an off-site venue added another layer of complexity as portable equipment for the study had to be sourced and stored safely at the venue, contracts had to be drawn up, and insurance and wi-fi had to be organized. The venue also had to be large, with enough space to set up a one-way route through the building for study participants. Parking and proximity to motorway networks were also important. As the turnaround from site initiation visit to delivery of the first vaccine was just two weeks, there was a lot to achieve in a short time.
Preparing the team
In conjunction with sourcing equipment, the team also had to be prepared, which proved challenging. For past studies, training would be delivered several weeks before the study began and would be followed by a meal to support networking and encourage attendance. This approach had previously been successful where off-site venues had been used, as it provided an opportunity for the study team to meet and learn about each other’s roles. During the pandemic, however, training was delivered remotely, so there was less opportunity for staff to build rapport and establish foundations to their working relationships.
The study leadership team felt it was essential to address these issues, as having a team that does not work cohesively often provides opportunities for error.Citation1 As the team was made up of a number of different professionals from numerous sites, scrubs were purchased and loaned to all team members in order to support the staff in building a team mental model.Citation2 Past experience had shown that this approach creates a feeling of belonging among team members and portrays a professional image to study participants. While having all staff in the same uniform can pose issues such as quick identification of roles, this was managed through staff introductions at the beginning of each day, use of identification badges and the layout of the working environment (in line with study requirements). For example, participants may be met initially by the administration team, moved through to the doctor team for counseling, and consent and eligibility assessment, then to the nurse team for specimen collection, vaccination, and online diary training, and finally to the administration team who complete the process and organize the follow-up visit.
Conducting the study
Prior to the vaccination session: the pre-brief
At the beginning of each vaccination session, the whole team gathered for ten minutes and the primary investigator (PI) or allocated sub investigator (sub-I) led a pre-brief. This included welcoming new team members and identifying their roles, setting out the plan for the day, including the number of participants to be vaccinated and planned start and finish times for the session, and updates on any common errors being made or protocol changes and deviations. In addition, the brief outlined emergency procedures, providing the location of emergency equipment such as anaphylaxis kits and defibrillators and assigning roles for emergencies, including the primary responder, the secondary responder, a scribe and someone to call and communicate with ambulance crews. Finally, the pre-brief provided an opportunity for the team to give feedback on any changes that could be made to improve the participants’ experience. Ensuring staff had the opportunity to contribute to the pre-brief and suggest changes ensured efficiency and promoted team working and belonging.
During the vaccination session: leadership and process
During each vaccination session, two team leaders supported the team, responding to any protocol or process queries, addressing equipment requirements, managing stock, helping with anxious or nervous patients and managing the flow of participants. The team leaders were approachable and supportive so that team members felt comfortable to ask questions and escalate concerns. Overarching leadership for the day was provided by the PI. In any quiet times during a session, the team practised managing a patient with anaphylaxis.
In the past, the team had used two different models for delivering vaccine studies: moving the participant around different team members to conduct the required tasks or placing the participant with one nurse who conducts all the required tasks. While moving the participant around had been more time effective, it was found that placing participants with one nurse provides a more person-centered approach, supports participant retention in the study, and is preferred by staff who have time with the participants allowing them to build rapport and complete the required tasks without feeling under pressure to move the participant on. As such, this approach was chosen for the Covid-19 vaccine study. As the team was assembled of staff from many different fields of practice with different working cultures, working in this style required team members to be flexible and supportive of one another. Varying levels of confidence with certain equipment and procedures had to be considered when asking staff to perform procedures they may not perform regularly in their normal setting. In addition, it was important that team members and staff were made to feel supported and encouraged to ask for help when needed. Finally, due to staffing pressures created through isolation requirements during the pandemic, doctor and nurse teams were overstaffed so that there were enough available team members to allow for the one nurse-one participant approach.
After the vaccination session: the debrief
At the end of each session, the team was led in a debrief by the PI, which included participants who were completing their post-vaccination observation period. Including participants in this session aimed to prevent the risk of a “groupthink” culture and to keep the study team open and transparent.Citation3 The debrief was used to check that team members and participants were happy with the processes in place and provide opportunity for any issues to be raised. Seeking the opinion of the whole team not only aimed to improve the participants’ experience, but also to ensure that all team members felt included.
Conclusion
The preparation and implementation of a Phase III, placebo-controlled, double-blind, multisite, Covid-19 vaccine study in Stockport included effective staffing, venue preparation, team training and leadership, which facilitated safe, effective, and efficient delivery of the Covid-19 vaccine to more than 750 recruited participants. With a team of just over 150 members, the effective delivery of this study highlighted the importance of flexible and supportive teamworking approaches.
Acknowledgments
Stockport NHS Foundation Trust and Research teams across the Greater Manchester NIHR network and associated teams. Writing and editorial assistance provided by the National Immunization Conference.
Declaration of interest
None.
CONTACT Professor David Baxter Director of Medical Education / Consultant in Public Health, Pinewood House, Stepping Hill Hospital, Stockport, SK2 7JE, UK
References
- Baker DP, Day R, Salas E. Teamwork as an essential component of high-reliability organizations. Health Serv Res. 2006;41(4 Pt 2):1576–98. doi:10.1111/j.1475-6773.2006.00566.x.
- Timmons S, East L. Uniforms, status and professional boundaries in hospital. Sociol Health Illn. 2011;33(7):1035–49. doi:10.1111/j.1467-9566.2011.01357.x.
- Wilde J Building cultures of transparency and openness. Implementing culture change within the NHS: contributions from Occupational Psychology. Occupational Psychology in Public Policy (OPIPP) Group Report. 2014:64–72. [accessed 2021 Nov]. https://orca.cardiff.ac.uk/111317/1/BPS%20publication.pdf
Introduction
The Royal College of Nursing (RCN), the world’s largest nursing union and professional body, has an important role in supporting best practice, developing guidance, providing information and resources to support nursing and midwifery staff, and influencing national policies. The large-scale, ongoing COVID-19 vaccination programme implemented rapidly in the UK in 2020 highlighted some key considerations for nursing teams. In response, the RCN developed in collaboration with other nursing organizations and with the Nursing and Midwifery Council (NMC) developed guidance to help maintain patient safety and public trust in vaccination, this supplemented RCN resources already available for immunization services.Citation1 In particular, there was a need for clarity regarding which professionals should administer COVID-19 vaccines, with ability, authority and accountability all to be considered. This article summarizes the criteria that should be satisfied in this respect, highlighting relevant guidance as well as regulatory and legal aspects that should be used to support decision-making.
Vaccine administration
Vaccine administration is primarily a nursing role in the UK. Nursing teams are best placed for this role as they traditionally work across all disciplines and within communities and thus understand local populations, allowing services and information to be tailored accordingly. The RCN provides valuable resources to support vaccination programmes, including ‘Managing childhood immunisation clinics – best practice guidelines,’Citation2 and ‘Practical and clinical guidance for vaccine administration.’Citation3 In addition, several principles have been defined by the RCN to help ensure patient safety and public trust in vaccination programmes. These include ensuring the workforce is trained and supported to enable safe delivery of vaccines, providing access to useful, relevant links and resources that are disseminated to professionals (via the RCN website and social media platforms), responding to clinical queries and concerns from members (and using these as a framework to develop resources and guidance), and providing workplace support for nursing staff.
Vaccination policy and practice
The government-issued green book ‘Immunisation against infectious diseases’ is used as a benchmark for vaccination policies throughout the UK,Citation4 for registered nursing staff the NMC standards and Code also need to be considered.Citation5 However, while policies are nationwide, regional practices can differ throughout the UK, and ensuring that appropriate policies are followed in local settings can cause confusion. Communication with national team members is a valuable tool in ensuring a concerted approach across the UK and in addressing any issues that may arise. Such issues include the most suitable length of time to allocate for vaccine administration and whether a doctor should be on-site for nurse-led vaccination. When addressing such issues, RCN guidance provides information that can help nurses and midwives justify and support their actions. This includes the guidance for vaccine delivery during COVID-19 ‘Immunization services and large-scale vaccination delivery during COVID-19,Citation6 which is continually updated to offer practical support. In addition, the RCN winter wellbeing resource include information on the annual influenza campaign and ongoing COVID-19 vaccine programme,Citation7 which is also applicable to the delivery of large-scale vaccination. Collectively, the RCN resources provide practical hints and tips, which can be adapted to specific community needs and may prove useful during the pandemic and beyond.
The NMC CodeCitation5 governs registered nurses, and presents the professional standards that nurses, midwives and nursing associates must uphold to be registered to practise in the UK. The NMC Code and standards aim to support nurses by providing key principles to follow, alongside the ethical frameworks that usually guide practice. The NMC Code in Action is another useful resource for nursing staff is centered around three main areas, patient safety and person-centered care, accountability, and professional judgment.Citation8 These resources have been beneficial during the COVID-19 pandemic, during which nurses have often been deployed to positions outside of their usual roles and environments.
Who can administer vaccines?
During the planning phase of the COVID-19 vaccination programme, there was a lack of clarity regarding which personnel could perform each role. Each profession (doctors, pharmacists, nurses) is governed by its professional body, with a requirement across the board to serve a duty of care to the public and uphold the standard of care expected of practitioners performing a particular task or role. In terms of accepting responsibility for a given task, and this includes not just accepting specific tasks but also the task of supervising and delegating to others. Three factors need to be considered, as follows ().
Table 3. Key considerations when selecting appropriate personnel for COVID-19 vaccine delivery.
Ability
Firstly, the level of training/competencies must be considered across all staff grades, ensuring that the individual has the training and skill to perform the task.
Public Health England provides National Minimum Standards (NMS) for immunization training within an agreed standard framework for registered healthcare practitioners (2018)Citation9 and nonregistered practitioners (2015),Citation10 which are both supported by the RCN. These standards are useful in assessing an individual’s ability, skill set and competence. Although the NMS only apply in England and Wales, they provide a valuable framework to support the delivery of immunization training across the UK.
While the necessary knowledge and skills required by an immunizer are dependent on the specific role of the individual, the vaccine and the scope of practice, core immunization training would constitute attendance of virtual, interactive, or face-to-face participatory learning sessions, which meet the curriculum, and assessment of competence through supervised practice using the relevant competent assessment tools.Citation9–11 Supervised practice should be structured and robust and follow the national competency checklist for the role undertaken. There is no guidance on the appropriate number of supervised practice sessions or vaccinations needed to determine competence. However, both the supervisor and immunizer should be confident that the individual has attained the necessary skills and knowledge to provide advice and administer vaccines.
The supervisor also needs the ability to undertake this assessment, notably, this role does not normally require a formal teaching or assessment qualification but individuals should be competent in immunization and also have the skills to assess an individual’s knowledge and skills and be able to arrange further development and learning for new vaccinators as appropriate.
Authority
Secondly, the individual must have the authority to perform the task (i.e., the task is part of their job description or within the policies and protocols of the respective organization).
Accountability
Finally, the individual must be accountable (i.e., they must have agreed to undertake the specific role/task).Citation12 Indeed, healthcare practitioners of all levels must ensure accountability across several domains. To their employer, healthcare practitioners must abide by a contract and job description and be identifiable by their name badge. To the patient, practitioners must be respectful, obtain consent for the delivery of care, and provide appropriate information. To themselves, practitioners must be appropriately trained and experienced and must always follow best practice. Finally, to society, practitioners must be trustworthy and honest.
Decision-making process – delegating and accepting responsibility for vaccine administration
The administration of the any vaccine programme including the COVID-19 vaccine requires a range of different people with varying education and professional experience to work together.
In addition to this all vaccines are prescription-only medicines, and under the Human medicines RegulationsCitation13 it is also essential to remember that appropriate authorization is required before they can be supplied and or administered. This is an important consideration for nursing staff. The regulations are complex and require detailed knowledge and understanding. Full explanation is beyond the scope of this article, but the RCN medicines management resources provide further information.Citation14 Before delegating or accepting responsibility for vaccine administration, the decision-making process should involve asking critical questions regarding ability, authority and accountability, ensuring that all these criteria are satisfied.
Acknowledgments
My Colleague Heather Randle Professional Lead for primary care who helped develop presentation slides.
Declaration of interest statement
None.
References
- Royal College of Nursing. Immunisation. [accessed 2021 Sep]. https://www.rcn.org.uk/clinical-topics/public-health/immunisation
- Royal College of Nursing. Managing childhood immunisation clinics. 2021 [accessed 2021 Sep].https://www.rcn.org.uk/professional-development/publications/managing-childhood-immunisation-clinics-uk-pub-009-860
- Royal College of Nursing. Practical and clinical guidance for vaccine administration. 2021 [accessed 2021 Sep]. https://www.rcn.org.uk/clinical-topics/public-health/immunisation/practical-and-clinical-guidance-for-vaccine-administration
- Public Health England The Green Book. [accessed 2021 Sep]. https://www.gov.uk/government/collections/immunisation-against-infectious-disease-the-green-book
- Nursing and Midwifery Council. The code. [Accessed 2021 Sep]. https://www.nmc.org.uk/standards/code/
- Royal College of Nursing. Immunisation services and large-scale vaccination delivery during COVID-19. 2021 [accessed 2021 Sep]. https://www.rcn.org.uk/clinical-topics/public-health/immunisation/immunisation-services-and-large-scale-vaccination-delivery-during-covid-19
- Royal College of Nursing. Winter wellbeing for 2021. [accessed 2021 Sep]. https://www.rcn.org.uk/get-involved/campaign-with-us/winter-wellbeing
- Nursing and Midwifery Council. NMC caring with confidence: the code in action. [accessed 2021 Sep]. https://www.nmc.org.uk/standards/code/
- Public Health England. Immunisation training standards for healthcare practitioners 2018. [accessed 2021 Sep]. https://www.gov.uk/government/publications/national-minimum-standards-and-core-curriculum-for-immunisation-training-for-registered-healthcare-practitioners
- Public Health England. Immunisation training of healthcare support workers: national minimum standards and core curriculum 2015. [accessed 2021 Sep]. https://www.gov.uk/government/publications/immunisation-training-of-healthcare-support-workers-national-minimum-standards-and-core-curriculum
- Royal College of Nursing. Immunisation knowledge and skills competence assessment tool 2018. [accessed 2021 Sep]. https://www.rcn.org.uk/professional-development/publications/pdf-006943
- Royal College of Nursing. Accountability and delegation. [accessed 2021 Sep]. https://www.rcn.org.uk/professional-development/accountability-and-delegation
- Human Medicines Regulations. 2012. [accessed 2021 Sep]. https://www.legislation.gov.uk/uksi/2012/1916/contents/made
- Royal College of Nursing. Medicines management. [accessed 2021 Sep]. https://www.rcn.org.uk/clinical-topics/medicines-management
Introduction
Shingles (herpes zoster) is caused by the reactivation of a latent varicella zoster virus (VZV) infection, generally decades after the primary infection. Primary VZV infection typically occurs during childhood and causes chickenpox (varicella) but following this primary VZV infection, the virus can enter the sensory nerves and travel along nerves to sensory dorsal root ganglia thus establishing a permanent latent infection. Reactivation of the latent virus may lead to the clinical manifestations of shingles and is associated with immune senescence or suppression of the immune system including immunosuppressive therapy, HIV infection, malignancy and/or increasing age. The annual incidence of shingles for those aged 70 to 79 years is estimated to be around 790 to 880 cases per 100,000 people in England and Wales.Citation1 The first signs of shingles are usually abnormal skin sensations and pain in the affected area of skin. Headache, photophobia, malaise and less commonly fever may occur as part of the prodromal phase. Within days or weeks, a fluid filled blisters typically appears in a dermatomal distribution. The affected area may be intensely painful with associated paresthesia and intense itching is common.Citation2 The rash typically lasts between two and four weeks. Following the rash, persistent pain at the site, known as Post Herpetic Neuralgia (PHN), can develop and is seen more frequently in older people.Citation3
The routine programme for people aged 70 years, using Zostavax® (Merck Sharp & Dohme [MSD], has been in place since 2013. At the same time a catch-up programme was rolled out to those aged 70–79 years in a phased approach. In 2017, Public Health England acknowledged the downward trend in the uptake of the shingles vaccine.Citation4 In the Maassarani Group Practice (Liverpool, UK), this decline was reflected with low attendance for annual reviews and poor patient engagement for preventative care, including screening and vaccinations. There was a marked disparity in attendance for preventive healthcare versus attendance when unwell. This report highlights the successful transition and the pivotal steps taken to facilitate a change in vaccination culture in four Maassarani Group Practice centers, with over 26,500 patients. The practices are in Merseyside and Knowsley, where people’s health is considered to be generally worse than the England average.Citation5
An initiative to increase vaccination uptake
A need for an initiative to increase lifelong patient vaccination uptake was identified, and the following aims were developed. Firstly, to focus on the shingles vaccine as it is a single vaccine with a well-defined cohort. The next aim was to develop a practice-generated framework, as an online search had failed to identify best practice guidelines. Thirdly to ensure that the framework was transferable, as the model would be used for all vaccinations, screening programmes and health promotion messages. Following this, the aim was to ensure the framework was inclusive, with a whole practice approach including clinicians, non-clinical staff, and patients to ensure ownership and investment. It was subsequently necessary to ensure the model was sustainable, with the aim of continued health improvements and then to ensure the model’s accessibility and availability for peer education. Finally, the initiative aimed to use open and inclusive communication.
A multidisciplinary steering group was formed by a lead nurse, a performance team manager, a communications manager, and an MSD vaccine implementation lead, focusing on the clinical provision, logistical improvements, and patient engagement.
Staff survey and training
A baseline vaccine-related knowledge survey was distributed among clinical and non-clinical staff to address knowledge gaps via adjusted training. The administrative staff proved reluctant regarding vaccine-promotion roles; therefore, a sales and marketing training approach was adopted. The staff must be familiar with the product (i.e., Zostavax vaccine) and the target patients to confidently challenge the patient’s perception and provide advice and answers when needed. The survey revealed poor knowledge regarding vaccination criteria, live vaccine, cohort, indication, and contraindication particularities, and a lack of clarity regarding health care assistants’ use of Patient Specific Directions (PSDs) and the purpose of vaccination.
The survey outcomes informed a whole practice training programme. The non-clinical staff was video trained on immunology and vaccines to minimize work disruptions and promote an open and secure environment. The staff reported positive feedback, highlighting a better understanding of the vaccination process and self-confidence.
The second video training explained the shingles vaccine’s transmission, symptoms, treatment, and PHN. A discussion on the planned shingles vaccination implementation programme followed. The videos were made available on the practice intranet, along with a question-and-answer wall where staff could post questions anonymously. Additionally, a training update on lifelong vaccinations focused on shingles vaccine, the health economic aspects of infection, particularly PHN and the associated burden of disease, to help prioritize and incorporate opportunistic vaccination into daily practice.
Communication skills were uplifted for all practice staff. The goal was to create a vaccine-confident culture, generate trust in vaccine efficacy and safety, challenge patients’ perceptions, inform and facilitate a positive change. Informative and educational materials were shared with patients via posters, websites, newsletter, and videos in the waiting area. It was agreed that the training investment offered the staff an updated sense of competence to effectively promote vaccines to patients. All staff received a key facts sheet on the shingles vaccine for reference if needed.
Other factors contributing to success
In addition to filling the staff training gaps, several other factors were key to the success of the initiative. Firstly, the frontline staff, who were the first point of contact for patients on the phone, electronically or in person, were often members of the same local community as the practice patients, and as such, patients were familiar with the staff. This factor, coupled with their skill set uplift, enabled nonclinical staff to become confident and persuasive advocates in influencing patients’ behavior. There were many volunteers to become a vaccination champion in each practice, with responsibilities including the distribution of promotional literature to patients and liaison with the patient participation group.
Secondly, the slogan ‘to make every vaccine contact count’ was adopted as an important tool in achieving our opportunistic vaccination culture goal. Approved desktop vaccine storage bags were placed in each clinician’s room to ensure minimal disruption and adequate time to vaccinate during regular consultations. Bags were filled, emptied, and monitored by a nominated administrative staff member. The vaccination champion further facilitated vaccine opportunities by identifying eligible patients from the appointment lists and ensuring PSDs were signed in readiness, where appropriate. Eligible patients were also signposted for vaccination by in-house and external pharmacy staff and by visiting clinicians.
The performance team also reviewed and improved their vaccination audit process as part of the vaccination drive. Audits were undertaken for all practices to identify and target eligible patients. Patients were contacted by telephone, and non-responders were followed up with a second telephone call and then by letter. Invitations to attend for vaccination were sent in the form of an MSD 70th birthday card. Importantly, all patients who declined or did not attend a vaccine appointment but remained eligible for vaccination were followed up and re-invited to attend.
Finally, as the start of the project coincided with the 70th anniversary of the NHS, a 1940s-themed event named ‘Mingle for Shingles’ was planned to launch the immunization initiative. The aim was to create community collaboration in a fun, inclusive and entertaining environment, which combined health promotion, disease prevention, a celebration of healthcare and, importantly, administration of the shingles vaccine. The event received overwhelming community support, and 34 shingles vaccinations were administered. Patients who did not attend were contacted, and 66% were vaccinated within the following 3 months. General practitioners, nurses and a senior practice pharmacist also attended the event and mingled informally with patients, which challenged perceptions and barriers and further connected practices with the community.
Finally, a revised system was subsequently developed for the call and recall team, which are central to managing patient bookings. The new system included an immunization lead responsible for operational management and performance monitoring; improved identification of patients who require vaccination, a systematic and targeted process for call and recall; administrative protocols built into the clinical system to ensure processes and pathways are adhered to, the use of targeted communication campaigns; methods to ensure accountability and ownership regarding vaccination and immunizations; and weekly and monthly targets for the number of vaccine-eligible patients receiving intervention.
The initiative led to improved vaccine uptake
The initial shingles vaccination campaign triggered effective change management in the practices, and the culture of immunization is now firmly anchored in practice behaviors and policy. This led to vastly improved vaccine uptake ().
Table 4. Shingles vaccine uptake among patients in the Maassarani Group Practice from 1 April 2017–31 March 2018 and 1 April to 31 March 2019.
Following the initiative’s success, the desktop vaccination cool bags remain in daily use and are routinely taken on home visits and to outreach clinics. All clinicians, including locums, are expected to vaccinate opportunistically. Vaccination training and awareness is included in the staff induction programme for both new clinical and non-clinical staff.
Summary
By taking a whole-practice approach and empowering all staff, the immunization initiative has facilitated an effective and sustainable way to improve and maintain vaccination uptake. Positive change was realized through investing in staff and the implementation of best practice techniques. The project has continued to evolve and has proved to be transferable to all vaccinations and other health promotion and health screening programmes throughout the practice.
Acknowledgments
My thanks go to Gemma Ray, Communications Manager, who played a crucial role in the success of this project by creating effective communication, information and health promotion strategies to create a vaccine confident culture amongst staff and patients. Also to Lee Panter, Managing Partner of Primary Care Knowsley, who has a forward-thinking management style and a commitment to continuously improving care. This meant I knew I could depend on Lee to provide 100% support for this project which proved to be invaluable, he enabled a whole practice approach, which was an essential factor to the positive outcomes. I was based at Cornerways Medical Center, Liverpool, where the non-clinical staff were the first participants in the training programmes, I really appreciated their patience (no pun intended) and their continuous support along with their enthusiasm for the vaccination cultural shift.
Acknowledgments
This is based in part upon a presentation at UK National Immunization Conference in December 2020. Writing and editorial assistance provided by the National Immunization Conference.
Declaration of interest
None.
CONTACT Yvonne Gibney [email protected]
References
- van Hoek AJ, Gay N, Melegaro A, Opstelten W, Edmunds WJ. Estimating the cost-effectiveness of vaccination against herpes zoster in England and Wales. Vaccine. 2009;27(9):1454–67. doi:10.1016/j.vaccine.2008.12.024.
- Gilden DH, Dueland AN, Cohrs R, Martin JR, Kleinschmidt-DeMasters BK, Mahalingam R. Preherpetic neuralgia. Neurology. 1991;41(8):1215–18. doi:10.1212/WNL.41.8.1215.
- Oxman MN, Levin MJ, Johnson GR, Schmader KE, Straus SE, Gelb LD, Arbeit RD, Simberkoff MS, Gershon AA, Davis LE, et al. Shingles Prevention Study Group. A vaccine to prevent herpes zoster and postherpetic neuralgia in older adults. N Engl J Med. 2005;352(22):2271–84. doi:10.1056/NEJMoa051016.
- Public Health England. Herpes zoster (shingles) immunisation programme 2017 to 2018: evaluation report. [Accessed 2021 Nov]. https://assets.publishing.service.gov.uk/government/uploads/system/uploads/attachment_data/file/758888/hpr4218_shngls_vc.pdf
- Public Health England. Knowsley Local Authority Health Profile. [accessed 2021 Nov]. https://psnc.org.uk/halton-st-helens-and-knowsley-lpc/wp-content/uploads/sites/45/2018/12/Knowsley-Health-Profile-2018.pdf
Meningococcal Disease
Although rare, meningococcal disease is associated with rapid onset within 48 hours, a 10–15% fatality rate and a high frequency of survivors (25%) are left with life-long sequelae, some of which are devastating. The main pathogens implicated in bacterial meningitis and/or sepsis are Haemophilus influenzae type b (Hib), Streptococcus pneumoniae, Group B streptococcus (GBS) and the meningococcus (Neisseria meningitidis). Neisseria meningitidis is the leading cause of bacterial meningitis, with serogroups A, B, C, W and Y causing the majority of disease.
Vaccine Development
The principle of using capsular polysaccharides and their conjugates as a vaccine antigen to induce an immune response has been used in the development of vaccines against the meningococcus serogroups A, B, C, W and Y. A 1992 study showed that conjugate vaccines for Meningococcal C induced high levels of bactericidal antibodies in infants, and that a plain polysaccharide vaccine was a poor immunogen. Since conjugate Meningococcal C (MenC) vaccines were introduced in the UK in 1999, they have significantly decreased disease.Citation1 In total, MenC vaccine has prevented greater than 20,000 cases, 2,000 deaths and 4,000 permanent sequelae.
Although conjugation of the capsular polysaccharide proved to be a highly successful strategy in the development of vaccines against Men A, C, W and Y disease, conjugation of the capsular polysaccharide is unsuitable against Meningococcal B (MenB) disease because the MenB polysaccharide capsule is generally unsuitable for vaccine development due to low immunogenicity and the potential risk for autoimmunity given the structural similarity of the capsule to certain abundant human glycoproteins.Citation2 Many alternative approaches to develop vaccines that protect against invasive diseases including strains of MenB have been undertaken ().Citation3
Figure 1. Vaccine technology.
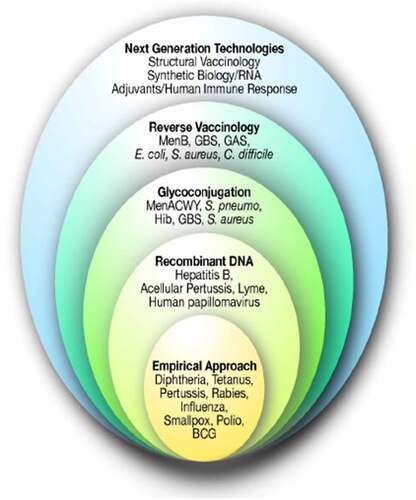
Reverse Vaccinology Use in Meningococcus B Vaccine Development
The discovery of vaccine antigens via whole genome sequencing (WGS) rather than the conventional laboratory-based analysis of microbes to identify components which may elicit protective immunity is termed reverse vaccinology. Use of reverse vaccinology was a change in scientific direction that led to the discovery of new vaccine antigens, many of which had not been discovered before the use of WGS because they were outer membrane proteins that had relatively low levels of surface expression.Citation3
A reverse vaccinology approach was utilized in the development of a MenB vaccine ().Citation3 Proteins located in the cytosol are generally poor immunological targets, whereas surface-associated structures are more likely to induce an immune response as they are more accessible. An in silico bioinformatics approach was used to identify novel antigens for vaccine development. The complete genome sequence of an isolate of Neisseria meningitidis serogroup B (MenB) was systematically screened to identify proteins predicted to be secreted or exported to the outer membrane that were localized in the periplasm or inner membrane. This led to the identification of 600 potential vaccine candidates.Citation3 Of the 600 predicted surface-exposed proteins, 350 were successfully expressed in Escherichia coli and purified as recombinant proteins. The antisera of these 350 recombinant antigens were analyzed, and 91 were proven to be surface-exposed, with 28 able to elicit a bactericidal response. The identification of 28 new potential vaccine candidates represented a real breakthrough.Citation3
Four-component MenB (4CMenB) Vaccine Development
Testing of the candidate vaccine antigens indicated that no single component would be sufficient to induce broad coverage and that a “universal” vaccine should contain multiple antigens. The final choice of antigens to be included was based on cross-protective ability, and maximum coverage of the extensive antigenic variability of MenB strains.Citation3 The resulting multivalent vaccine formulation selected consisted of three main Neisseria protein recombinant antigens; Neisserial Heparin Binding Antigen (NHBA), Factor H binding protein (fHbp) and Neisseria Adhesin A (NadA). To improve immunogenicity and potential strain coverage, PorA (Porin A, the immunodominant antigen of the outer membrane vesicle [OMV]) obtained from a serogroup B strain, NZ98/254, successfully used during an epidemic in New Zealand in 2004–2008 was added to create a four-component vaccine, called 4CMenB.Citation3 The 4CMenB vaccine was introduced into the UK National Infant Immunization programme on September 1st, 2015.Citation4
4CMenB vaccine efficacy
During the first three years of the 4CMenB immunization program, there was a 75% decrease in MenB disease in the UK.Citation5 Vaccine efficacy was assessed by comparing the observed efficacy in fully eligible cohorts irrespective of vaccination status or predicted MenB strain coverage compared to the predicted number of cases in the absence of vaccination (based on observed trends among unvaccinated childhood cohorts over the same period). Cases of MenB continued to fall in the fourth year and have continued to do so every year since the program was implemented, thus protecting more birth cohorts.Citation6 Vaccine effectiveness was calculated using screening methods and, further, using a Poisson regression model to improve precision ().Citation5,Citation7
Table 5. Vaccine efficacy of 4CMenB evaluated by two different techniques.
The effectiveness of 4CMenB is supported by data from real-world experience across multiple settings:
UK national immunization program – 75% decrease in MenB diseaseCitation6
Canada (Saguenay-Lac-Saint-Jean region) outbreak setting − 96% decrease in MenB diseaseCitation8
USA university outbreak setting – no cases in vaccinated individualsCitation9,Citation10
Portugal endemic setting – 79% effectivenessCitation11
Italy national immunization program – effectiveness of 91% in Veneto and 93.6% in TuscanyCitation12
South Australia state-wide study in adolescents − no cases in vaccinated individualsCitation13
Cross-reactive immunity of 4CMenB
Use of 4CMenB has also demonstrated cross-reactive immunity against other non-serogroup B meningococcal serogroups (C, W, Y).Citation14 A study evaluated the ability of antibodies raised by 4CMenB immunization to induce serum bactericidal activity on non-MenB strains by collecting and analyzing meningococcal disease isolates in several European countries and Brazil.Citation15 The Euro-3 panel countries consisted of England and Wales, Germany and France with a total of 227 non-MenB isolates collected between July 1st, 2007 and June 30th, 2008. In the Brazilian panel, 41 non-MenB isolates were collected during 2012. All strains were characterized by serogroup, multi-locus sequence typing and 4CMenB antigenic sequence (fHbp, NHBA and PorA) and the presence of the NadA gene. 4CMenB infant sera killed >70% of representative strains for serogroups C, W and Y of the non-B strains tested. The percentage of non-B isolates killed using human complement serum bactericidal activity assay (hBSA) at bactericidal titers ≥4 in the Euro-3 panel countries classified by serogroup were: MenC (n = 76): 66%, MenW (n = 28): 75%; MenY (n = 23): 91%; all (n = 127): 72%.
The laboratory-based findings that 4CMenB may offer additional protection against MenW have been confirmed in a real-world setting.Citation15 Poisson model estimates were used to compare meningococcal group W (MenW) cases confirmed during the 4 years before and 4 years after implementation of the 4CMenB programme in England. There were 69% fewer MenW cases in fully immunized children receiving 4CMenB than predicted. In total, there were 138 MenW cases in infants and children up to 5 years old. 4CMenB directly prevented 98 (95%CI, 34 − 201) cases. Vaccine effectiveness was 88% but with wide confidence intervals because of small case numbers. Disease severity was similar in 4CMen B immunized and non-immunized children.
Neisseria – Can we kill two birds with one stone?
The Neisseria genus comprises many species isolates, with the three main ones being Neisseria meningitidis, Neisseria gonorrhoeae and Neisseria lactamica. Both N. meningitidis and N. gonorrhoeae can be pathogenic whilst N. lactamica is a commensal. Notably, 80–90% genome identity is shared between meningococcus and gonococcus. However, extensively drug resistant (XDR) strains of N. gonorrhoeae are emerging globally. The World Health Organization (WHO) warns that an untreatable gonorrhea ‘superbug’ is spreading around the world.Citation16,Citation17 Antibiotic resistance is a growing concern and WHO tells of a ‘very serious situation’ after confirming three known cases where all antibiotics used in treatment proved to be ineffective.
Surveillance data suggested that the OMV meningococcal group B vaccine that was used successfully in the vaccination campaign to eliminate the meningococcus B epidemic in New Zealand in infants and children, also affected the incidence of gonorrhea. A retrospective case control study in New Zealand was carried out and demonstrated 31% vaccine effectiveness in protection against gonococcus.Citation18 Another retrospective case-controlled study in the USA in 2020 reported 18,369 gonorrhea infection cases, with an estimated multicomponent MenB (MenB-4C) vaccine effectiveness against gonorrhea of 40% (95% CI, 25 − 53).Citation19
Conclusion
The development of a vaccine to protect against MenB disease has been a major milestone in the fight to eliminate meningococcal meningitis. Successes seen with the use of reverse vaccinology to develop meningitis vaccines may also help in the fight to conquer gonococcus.
Acknowledgments
This is based in part upon a presentation at UK National Immunization Conference in December 2020. Writing and editorial assistance provided by the National Immunization Conference.
Declaration of interest
None.
References
- Campbell H, Borrow R, Salisbury D, Miller E. Meningococcal C conjugate vaccine: the experience in England and Wales. Vaccine. 2009;27(Suppl 2):B20–9. doi:10.1016/j.vaccine.2009.04.067.
- Holst J, Oster P, Arnold R, Tatley M, Næss L, Aaberge I, Galloway Y, McNicholas A, O’Hallahan J, Rosenqvist E, et al. Vaccines against meningococcal serogroup B disease containing outer membrane vesicles (OMV): lessons from past programs and implications for the future. Hum Vaccin Immunother. 2013;9(6):1241–53. doi:10.4161/hv.24129.
- Masignani V, Pizza M, Moxon ER. The development of a vaccine against meningococcus B using reverse vaccinology. Front Immunol. 2019;10:751. doi:10.3389/fimmu.2019.00751.
- Ladhani SN, Ramsay M, Borrow R, Riordan A, Watson JM, Pollard AJ. Enter B and W: two new meningococcal vaccine programmes launched. Arch Dis Child. 2016;101(1):91–95. doi:10.1136/archdischild-2015-308928.
- Isitt C, Cosgrove CA, Ramsay ME, Ladhani SN. Success of 4CMenB in preventing meningococcal disease: evidence from real-world experience. Arch Dis Child. 2020 Aug;105(8):784–90. doi:10.1136/archdischild-2019-318047.
- Ladhani SN, Andrews N, Parikh SR, Campbell H, White J, Edelstein M, Bai X, Lucidarme J, Borrow R, Ramsay ME, et al. Vaccination of infants with meningococcal Group B vaccine (4CMenB) in England. N Engl J Med. 2020 Jan;382(4):309–17. doi:10.1056/NEJMoa1901229.
- Orenstein WA, Bernier RH, Dondero TJ, Hinman AR, Marks JS, Bart KJ, Sirotkin B. Field evaluation of vaccine efficacy. Bull World Health Organ. 1985;63:1055–68.
- De Wals P, Deceuninck G, Lefebvre B, Tsang R, Law D, De Serres G, Gilca V, Gilca R, Boulianne N. Impact of an immunization campaign to control an increased incidence of serogroup B meningococcal disease in one region of Quebec, Canada. Clin Infect Dis. 2017;64(9):1263–67. doi:10.1093/cid/cix154.
- Basta NE, Mahmoud AAF, Wolfson J, Ploss A, Heller BL, Hanna S, Johnsen P, Izzo R, Grenfell BT, Findlow J, et al. Immunogenicity of a meningococcal b vaccine during a University outbreak. New Eng J Med. 2016;375(3):220–28. doi:10.1056/NEJMoa1514866.
- Grogan J, Roos K. Serogroup B meningococcus outbreaks, prevalence, and the case for standard vaccination. Curr Infect Dis Rep. 2017;19(9):30. doi:10.1007/s11908-017-0587-4.
- Rodrigues FMP, Marlow R, Simões MJ, Danon L, Ladhani S, Finn A. Association of use of a meningococcus Group B vaccine with Group B invasive meningococcal disease among children in Portugal. JAMA. 2020;324(21):2187–94. doi:10.1001/jama.2020.20449.
- Azzari C, Moriondo M, Nieddu F, Guarnieri V, Lodi L, Canessa C, Indolfi G, Giovannini M, Napoletano G, Russo F, et al. Effectiveness and impact of the 4CMenB vaccine against Group B meningococcal disease in two Italian regions using different vaccination schedules: a five-year retrospective observational study (2014-2018). Vaccines (Basel). 2020;8(3):469. doi:10.3390/vaccines8030469.
- Marshall HS, McMillan M, Koehler AP, Lawrence A, Sullivan TR, MacLennan JM, Maiden MCJ, Ladhani SN, Ramsay ME, Trotter C, et al. Meningococcal B vaccine and meningococcal carriage in adolescents in Australia. N Engl J Med. 2020;382(4):318–27. doi:10.1056/NEJMoa1900236.
- Biolchi A, De Angelis G, Moschioni M, Tomei S, Brunelli B, Giuliani M, Bambini S, Borrow R, Claus H, Gorla MCO, et al. Multicomponent meningococcal serogroup B vaccination elicits cross-reactive immunity in infants against genetically diverse serogroup C, W and Y invasive disease isolates. Vaccine. 2020;38(47):7542–50. doi:10.1016/j.vaccine.2020.09.050.
- Ladhani SN, et al. First real world evidence of meningococcal group B vaccine, 4CMenB, protection against meningococcal group W disease; prospective enhanced national surveillance, England. Clin Infect Dis. 2020;ciaa1244.
- World Health Organization. Multi-drug resistant gonorrhoea. 2020. [accessed 17th 2020 Aug. https://www.who.int/news-room/fact-sheets/detail/multi-drug-resistant-gonorrhoea
- World Health Organization. Emergence of multi-drug resistant Neisseria gonorrhoeae – threat of global rise in untreatable sexually transmitted infections. 2012.[accessed 2021 Nov].https://www.who.int/publications/i/item/emergence-of-multi-drug-resistant-neisseria-gonorrhoeae—threat-of-global-rise-in-untreatable-sexually-transmitted-infections
- Petousis-Harris H, Paynter J, Morgan J, Saxton P, McArdle B, Goodyear-Smith F, Black S. Effectiveness of a group B outer membrane vesicle meningococcal vaccine against gonorrhoea in New Zealand: a retrospective case-control study. Lancet. 2017;390(10102):1603–10. doi:10.1016/S0140-6736(17)31449-6.
- 2020 STD Prevention Conference September 14–24, 2020: erratum. Sex Transm Dis. 2021 February;48(2):e34. doi:10.1097/OLQ.0000000000001346.
Introduction
Meningitis and neonatal sepsis combined are estimated to be the second-largest infectious cause of death in children under 5 years old.Citation1 Despite significant progress over the last two decades, meningitis remains a much-feared disease globally. The main reasons behind this are the high case fatality rate and the propensity to cause epidemics which present a major challenge for health systems and economies. To help reduce preventable child deaths, the World Health Organization (WHO) produced the first-ever roadmap to help defeat meningitis by 2030. The roadmap involved hundreds of experts, Member States representatives, partners, Civil Society Organization as well as private sector representatives, through multidisciplinary, iterative and comprehensive consultations. The defeating meningitis by 2030 global road map was approved by the seventy-third session of the World Health Assembly in November 2020 (resolution WHA73.9).Citation2
The global road map encompasses five key interconnected pillars, as follows:
Prevention and epidemic control focused on the development of new affordable vaccines, achievement of high immunization coverage, improvement of prevention strategies and response to epidemics;
Diagnosis and treatment, focused on speedy confirmation of meningitis and optimal management;
Disease surveillance to guide meningitis prevention and control;
Care and support of those affected by meningitis, focusing on early recognition and improved access care and support for after-effects from meningitis; and
Advocacy and engagement, to ensure high awareness of meningitis, consideration into countries plans, and increase the right to prevention, care and after-care services.
Although the road map on defeating meningitis addresses all cases of meningitis regardless of cause, it mainly focuses on the main causes of acute bacterial meningitis which are meningococcal, pneumococcal, Haemophilus influenzae and group B streptococci.
The Meningitis Progress Tracker – methods and progress to date
The Meningitis Progress Tracker (MPT) is an interactive visualization tool helping to track and facilitate this initiative. Developed by the Meningitis Research Foundation, it brings multiple global sources of estimates of disease burden and data on meningitis together into one place for the first time,Citation3 it represents the first ever visualization of the story of meningitis, with a stated aim to track progress toward defeating meningitis. Source of estimates used in the MPT are the Institute of Health Metrics and Evaluation, or IHME, the WHO Global Health Estimates group, and the WHO and the Johns Hopkins Bloomberg School of Public Health’s Maternal and Child Epidemiology Estimation group’s Child Mortality Estimation.Citation4–7
The MPT is structured around the five pillars of the WHO Defeating Meningitis by 2030 roadmap and provides users with simple, accessible visualizations of meningitis related data. This is of primary importance as the data on the global meningitis burden are hard to interpret, in part due to there being multiple causes, and because the data come from several different sources. While monitoring the progress of the WHO initiative is its primary aim, the MPT can also be used as a learning and development tool.
The pilot study with the prototype MPT 1.0 was launched on World Meningitis Day 2019 (Wednesday 24th April) and to date has attracted over 33,000 users from over 70 countries. MPT 1.0 provided users with estimated numbers of cases and deaths from neonatal sepsis and meningitis by syndrome and pathogenic cause in children under 5 years of age.Citation1
A major update, MPT 2.0, was launched in September 2020 expanded on MPT 1.0 to include new visualization tools such as the disease burden landing page, which has been expanded to include data for all age groups and incidence and mortality rates for meningitis by syndrome, pathogenic cause, and neonatal sepsis. A year slider helps visualize how disease burden has changed since 2000. Additionally, a page aligned to the prevention and epidemic control pillar of the roadmap shows information on vaccine targets, types of vaccine, age groups and different country sets, and for a specific country can determine vaccination schedules and use. The tracking progress page also shows which countries have the most cases or greatest number of deaths and allows data comparison from different countries, and a country profiles tab allows users to easily access country-specific progress and monitor their status regarding specific indicators relevant to the roadmap.
Globally, routine vaccination programmes against meningococcal meningitis have been added to the existing data on global coverage of pneumococcal and Hib vaccines, with detailed information on the control of meningococcal A (MenA) in the meningitis belt.Citation8 For the first time, WHO Inter-country Support Teams surveillance data have been incorporated from the African meningitis belt, and a country profiles tab has been added to summarize country-specific progress and status with regards to specific indicators relevant to the roadmap. The platform can be used to ascertain information about disease types (meningitis and neonatal sepsis) and causes (meningococcal, pneumococcal and Haemophilus influenzae type b [Hib]) in particular countries, regions, and age groups. Users of the MPT can interact with the data on different pages of the MPT, filtering by age, pathogen (meningococcal, pneumococcal and Hib), estimation source and geography to produce visuals of most significant relevance. shows a screen shot of the MPT taken in September 2021, indicating 462,452 estimated total deaths and 8,427,054 estimated total cases of meningitis and neonatal sepsis combined globally.
Summary
Reducing cases and deaths from vaccine-preventable bacterial meningitis is integral to the WHO Defeating Meningitis by 2030 roadmap vision. Meningitis and neonatal sepsis together are within the top three most important infectious syndromes.Citation7 By far the biggest burden of meningitis is estimated to occur in countries with low quality or no death registration data thus data relies heavily on extrapolating from verbal autopsy studies.Citation7 The MPT visualizes multiple sources of global estimates of disease burden together in one place, bringing together indicators for prevention, treatment and support for meningitis and making it easier for civil society groups and other stakeholders to advocate for the implementation of the roadmap in their own countries. The MPT will be further developed to add more worldwide real-time surveillance data, and new indicators of progress for diagnosis, treatment and aftercare for meningitis. Future plans also include the addition of data on group B Streptococcus. More work is required to provide credible meningitis burden estimates for measuring progress. More data on cause of death in regions where child mortality rates are the highest are becoming available through the use for example of minimally invasive tissue sampling.Citation9
Acknowledgments
This is based in part upon a presentation at UK National Immunization Conference in December 2020. Writing and editorial assistance provided by the National Immunization Conference.
Declaration of interest
None
References
- Meningitis Research Foundation. Meningitis progress tracker. [accessed 2021 Sep]. https://www.meningitis.org/mpt
- World Health Assembly. Resolution WHA73.9. Global road map on defeating meningitis by 2030. 2020 Nov [accessed 2021 Sep]. https://apps.who.int/gb/ebwha/pdf_files/WHA73/A73_R9-en.pdf
- Meningitis Progress Tracker. 2021. [accessed 2021 Sep]. https://www.meningitis.org/mpt
- World Health Organization. Disease burden and mortality estimates. Child causes of death, 2000–2017. [accessed 2021 Sep]. https://www.who.int/healthinfo/global_burden_disease/estimates/en/index2.html
- Global Burden of Disease Collaborative Network. Global Burden of Disease Study 2017 (GBD 2017) results. Seattle (United States): Institute for Health Metrics and Evaluation (IHME); 2018. [accessed 2021 Sep]. http://ghdx.healthdata.org/gbd-results-tool
- Wahl B, O’Brien KL, Greenbaum A, Majumder A, Liu L, Chu Y, Lukšić I, Nair H, McAllister DA, Campbell H, et al. Burden of Streptococcus pneumoniae and Haemophilus influenzae type b disease in children in the era of conjugate vaccines: global, regional, and national estimates for 2000-15. Lancet Glob Health. 2018;6(7):e744–e757. doi:10.1016/S2214-109X(18)30247-X.
- Wright C, Blake N, Glennie L, Smith V, Bender R, Kyu H, Wunrow HY, Liu L, Yeung D, Knoll MD, et al. The global burden of meningitis in children: challenges with interpreting global health estimates. Microorganisms. 2021;9(2):377. doi:10.3390/microorganisms9020377.
- Global vaccine market model – demand module, Linksbridge SPC, last major update for 2019 MI4A meningococcal vaccine market study [accessed 2021 Sep].
- Bassat Q, Ordi J, Vila J, Ismail MR, Carrilho C, Lacerda M, Munguambe K, Odhiambo F, Lell B, Sow S, et al. Development of a post-mortem procedure to reduce the uncertainty regarding causes of death in developing countries. Lancet Glob Health. 2013;1(3):e125–6. doi:10.1016/S2214-109X(13)70037-8.
Introduction
Neisseria meningitidis is a leading global cause of meningitis and septicemia. Among the six main serogroups (A, B, C, W, X and Y), serogroup B (MenB) is currently the leading cause of invasive meningococcal disease in industrialized nations. Owing to the poor immunogenicity of the MenB capsule, MenB vaccines are based on antigens that are inherently subject to variation. The 4CMenB vaccine (Bexsero, GlaxoSmithKline UK) contains several proteins, notably Porin A (PorA) P1.4, factor H binding protein (fHbp), Neisserial Heparin Binding Antigen (NHBA) and Neisseria adhesin A (NadA).Citation1 In order to be covered by 4CMenB, anisolate must express PorA P1.4 and/or express a sufficiently cross-reactive variant of at least one of the other peptides.Citation2 The correlate of protection for MenB is a titer >1:4 in the serum bactericidal antibody assay using human complement (hSBA).Citation3 Since it is not practical to test all invasive MenB isolates using the hSBA assay, the Meningococcal Antigen Typing System (MATS) assay was developed to assess isolate/strain coverage.Citation2 The MATS assay utilizes sero/genotyping to characterize PorA, and an enzyme-linked immunosorbent assay (ELISA) to assess cross-reactivity and expression of fHbp, NHBA and NadA. If an isolate possesses PorA P1.4 and/or the relative potency (RP; versus a reference strain) of at least one of the other antigens exceeds a protective threshold, the positive bactericidal threshold (PBT), the isolate is considered covered.
The MATS assay is performed by a limited number of laboratories worldwide. Furthermore, MATS requires a viable isolate which is not always available owing to early antibiotic administration. Therefore, relatively accessible schemes, Genetic (g)MATS and the Meningococcal Deduced Vaccine Antigen Reactivity (MenDeVAR) Index, have been developed to predict strain coverage based on genotypic data alone.Citation4,Citation5 gMATS was based on a large MATS dataset (published and unpublished) and MenDeVAR was developed using a relatively limited set of published MATS data. MenDeVAR also enables genotypic analysis of strain coverage by the MenB-fHBP vaccine (Trumenba, Pfizer Limited), based on published hSBA and flow cytometry data. Characteristics of gMATS and MenDeVAR are summarized in .
Table 6. Key similarities/differences between gMATS and MenDeVAR.
The aim of this study was to compare estimated 4CMenB coverage of English MenB isolates according to MATS, gMATS and MenDeVAR.
Methods
The study included all English invasive MenB isolates (n = 792) from epidemiological years (July to June, inclusive) 2014/15 to 2017/18. Genotypic data were obtained from draft genomes hosted on the Meningitis Research Foundation Meningococcus Genome Library (MGL).Citation6. The MATS assay was performed in accordance with Donnelly et al. 2010 (with fHbp PBT = 0.012).Citation2 gMATS was applied in accordance with Muzzi et al., 2019.Citation4 MenDeVAR was applied in accordance with Rodrigues et al., 2020.Citation5
Results
MATS provided a 4CMenB coverage prediction (covered or not covered) for all of the isolates. gMATS provided a coverage prediction for 76.5% (n = 606/792) of isolates with the remainder unpredictable. MenDeVAR provided a coverage estimate for 63.3% (n = 501/792) of isolates with the remainder unpredictable.
According to MATS, 72.3% (n = 573/792) of isolates were covered by 4CMenB and 27.7% (n = 219/792) were not covered (). On an individual basis, gMATS predicted that 62.2% (n = 493/792) of isolates would be covered by 4CMenB and 14.3% (n = 113/792) of isolates would not be covered. Once the unpredictable isolates had been divided equally into covered and not covered, gMATS coverage was similar to that of MATS with 74.0% (n = 586/792) of isolates covered and 26.0% (n = 206/792) not covered. MenDeVAR, which does not divide unpredictable isolates, was more dissimilar with 54.0% (n = 428/792) of isolates covered and 9.2% (n = 73/792) not covered.
Figure 4. 4CMenB coverage of English invasive MenB isolates from 2014/15 to 2017/18 as predicted by MATS, gMATS and MenDeVAR.
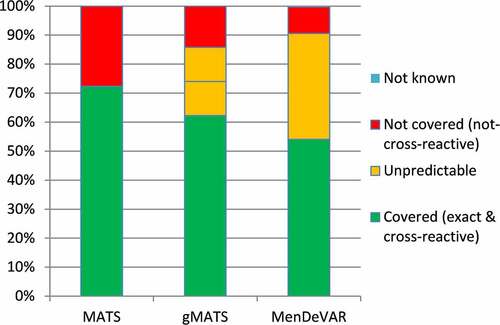
gMATS and MenDeVAR were fully in agreement for 4CMenB coverage of PorA (17.0% covered, n = 135/792) and for 97.5% of isolates for coverage of NadA (n = 772/792). This included non-coverage of nadA− isolates (n = 602, 76.0%) and isolates not expected to express NadA (phase variable off n = 79, 10.0%; frameshifted/insertionally inactivated alleles, n = 13, 1.6%). They were also in agreement for isolates possessing alleles for NadA peptides deemed not to be covered by MenDeVAR (peptides 1, 21 and 100, n = 78, 9.8%, collectively). Since gMATS considers no NadA peptides to be covered, the two schemes were in disagreement for isolates with (i) peptides deemed covered by MenDeVAR (peptides 3, 6 and 8; n = 3 collectively), (ii) peptides deemed indeterminate by MenDeVAR including NadA-1 (n = 3), NadA-2/3 (n = 2) and NadA-4/5 (n = 2) peptides, and (iii) incompletely assembled NadA-4/5 and NadA-6 alleles which were, therefore, also indeterminate for MenDeVAR (n = 10).
For fHbp, gMATS and MenDeVAR were in agreement for 53.0% (n = 63/119) of peptides across 89.5% (n = 709/792) of isolates. Among the 10.5% of isolates where disagreements existed, 73.5% (n = 61/83) were because of variant 2/3 peptides that gMATS considered not covered, and MenDeVAR considered indeterminate. A further four disagreements related to isolates with alleles for peptides 10 and 254 which were considered covered by MenDeVAR but indeterminate by gMATS since a test of proportions was unable to reject 50% coverage as a null hypothesis. The remaining 18 disagreements related to peptides 2, 90, 224, 245, 249 and 510, which were considered covered by gMATS but indeterminate by MenDeVAR, despite >75% of corresponding isolates being covered in the original gMATS dataset.Citation4
For NHBA, gMATS and MenDeVAR were in agreement for 87.8% (n = 72/82) of peptides across 76.9% (n = 609/792) of isolates. Among the 23.1% of isolates where disagreements existed, 80.3% (n = 147/183) were due to peptides (3, 20 and 21) considered covered by gMATS and indeterminate by MenDeVAR. In the original gMATS dataset,Citation4 these were covered in 65.7% (n = 234/356), 64.8% (n = 140/216) and 69.9% (n = 267/382) of isolates, respectively, and so didn’t reach the 75% threshold of MenDeVAR. Disagreements among a further 18.0% (n = 33/183) of isolates were due to peptides (13, 58, 144, 122 and 24) that were considered not covered by gMATS and indeterminate by MenDeVAR. In the original gMATS dataset,Citation4 no isolates with peptides 13 (n = 6), 58 (n = 7), 144 (n = 18) or 122 (n = 14) were covered, while peptide 24 was covered among 34.8% (n = 32/92) of isolates. The remaining disagreements, observed among three isolates (1.6%, n = 3/183), related to peptides 607 and 197 which were covered and not covered, respectively, by MenDeVAR but indeterminate by gMATS having not reached significance in the test of proportions.
Discussion
Against a recent panel of English invasive MenB isolates, gMATS performed better than the published version of MenDeVAR in terms of the proportion of isolates given a prediction and its closeness to MATS outcomes. Individual PorA-based predictions were identical between MenDeVAR and gMATS, as expected given identical criteria. NadA-based predictions were similar between gMATS and MenDeVAR but could have been improved if the latter considered NadA-4/5 and NadA-6 peptides de facto not covered, as the literature indicates.Citation1,Citation7
The main causes of discrepancies between gMATS and MenDeVAR were (i) differences in the relative stringencies of the covered/cross-reactive and not covered/ not cross-reactive thresholds, (ii) consideration of fHbp variant 2 and 3 peptides as potentially cross-reactive by MenDeVAR (similar to NadA-4/5 and NadA-6 above), (iii) unavailability of certain MATS data to inform MenDeVAR due to non- (or pending) publication, (iv) the lack of a system in MenDeVAR to divide unpredictable isolates between covered and not-covered when dealing with a national dataset.
The complete and timely publication of MATS data, adjustments to MenDeVAR criteria surrounding well established non-covered variants,Citation1 and development of a system to divide unpredictable isolates in national datasets should be possible and would serve to improve MenDeVAR. Adjustment of the cross-reactive/not cross-reactive thresholds would, however, constitute a big change to the published MenDeVAR criteria. A possible way forward may be to add a second set of ‘low stringency’ thresholds.
It could be argued that the relatively stringent thresholds of MenDeVAR would improve vaccine selection in an outbreak scenario. For example, the USA university cluster referred to in the MenDeVAR publication involved a strain possessing alleles for fHBP peptide 19 and NHBA peptide 20.Citation8 fHbp peptide 19 is not covered by 4CMenB for either scheme, whilst MenDeVAR considers it cross-reactive with MenB-fHBP. NHBA peptide 20 is covered by 4CMenB according to gMATS (65% positivity in original gMATS datasetCitation4) but is indeterminate according to MenDeVAR. The developers of MenDeVAR therefore proposed that when using MenDeVAR “this information could have directed public health specialists to using Trumenba early after IMD cluster definition was met, preventing delays in health protection interventions, including mass vaccination campaigns, frequently required in university settings.” Flow cytometry of the outbreak strain indicated, however, that fHbp expression was ‘relatively low’ and that NHBA binding of antisera raised against NHBA peptide 2 (the vaccine variant) was also ‘low.’Citation8 This highlights the complexity of such decisions, which will invariably benefit from input from national meningococcal reference laboratories.
Given (i) MenDeVAR’s privileged location on the PubMLST platform,Citation9 (ii) its inherently ‘live’ nature, and (iii) its utilization in estimating coverage by both MenB vaccines, it may, in due course, replace gMATS. Therefore, implementing changes similar to the ones suggested above should be considered to align performance to gMATS level. Meanwhile, as new strains and antigenic variants continue to arise, the use of MATS will need to continue in order to inform all such MATS-based genotypic schemes.
CONTACT Jay Lucidarme [email protected] Meningococcal Reference Unit, Manchester Medical Microbiology Partnership, Floor 2 Clinical Sciences Building II, Manchester Royal Infirmary, Oxford Road, Manchester, M13 9WL, UK
Acknowledgments
This publication made use of the Neisseria Multi Locus Sequence Typing website (https://pubmlst.org/organisms/neisseria-spp/) sited at the University of Oxford. The development of this site has been funded by the Wellcome Trust and the European Union.Citation9 It also made use of the Meningitis Research Foundation Meningococcus Genome Library (MGL; http://www.meningitis.org/research/genome) developed by Public Health England, the Wellcome Trust Sanger Institute and the University of Oxford as a collaboration. The MGL project was part funded by Meningitis Research Foundation. Material and funding for the MATS assay used in this study were provided by GlaxoSmithKline Biologicals SA.
Disclosure statement
JL, XB, AL, LW, SAC and RB perform contract research on behalf of the UK Health Security Agency for GlaxoSmithKline, Pfizer, and Sanofi Pasteur.
References
- Serruto D, Bottomley MJ, Ram S, Giuliani MM, Rappuoli R. The new multicomponent vaccine against meningococcal serogroup B, 4CMenB: immunological, functional and structural characterization of the antigens. Vaccine. 2012;30(Suppl 2):B87–97. doi:10.1016/j.vaccine.2012.01.033.
- Donnelly J, Medini D, Boccadifuoco G, Biolchi A, Ward J, Frasch C, Moxon ER, Stella M, Comanducci M, Bambini S, et al. Qualitative and quantitative assessment of meningococcal antigens to evaluate the potential strain coverage of protein-based vaccines. Proc Natl Acad Sci U S A. 2010;107(45):19490–5. doi:10.1073/pnas.1013758107.
- Lucidarme J, et al. Meningococcal serogroup A, B, C, W, X, and Y serum bactericidal antibody assays. Methods Mol Biol. 2019;1969:169–79.
- Muzzi A, Brozzi A, Serino L, Bodini M, Abad R, Caugant D, Comanducci M, Lemos AP, Gorla MC, Křížová P, et al. Genetic Meningococcal Antigen Typing System (gMATS): a genotyping tool that predicts 4CMenB strain coverage worldwide. Vaccine. 2019 Feb 8;37(7):991–1000. doi:10.1016/j.vaccine.2018.12.061.
- Rodrigues CMC, Jolley KA, Smith A, Cameron JC, Feavers IM, Maiden MCJ. Meningococcal Deduced Vaccine Antigen Reactivity (MenDeVAR) index: a rapid and accessible tool that exploits genomic data in public health and clinical microbiology applications. J Clin Microbiol. 2020;59(1). doi:10.1128/JCM.02161-20.
- Hill DM, Lucidarme J, Gray SJ, Newbold LS, Ure R, Brehony C, Harrison OB, Bray JE, Jolley KA, Bratcher HB, et al. Genomic epidemiology of age-associated meningococcal lineages in national surveillance: an observational cohort study. Lancet Infect Dis. 2015;15(12):1420–8. doi:10.1016/S1473-3099(15)00267-4.
- Comanducci M, Bambini S, Caugant DA, Mora M, Brunelli B, Capecchi B, Ciucchi L, Rappuoli R, Pizza M. NadA diversity and carriage in Neisseria meningitidis. Infect Immun. 2004;72(7):4217–23. doi:10.1128/IAI.72.7.4217-4223.2004.
- Soeters HM, et al. Serogroup B meningococcal disease vaccine recommendations at a university, New Jersey, USA, 2016. Emerg Infect Dis. 2016May, 23(5), 867–9.
- Jolley KA, Bray JE, Maiden MCJ. Open-access bacterial population genomics: bIGSdb software, the PubMLST.org website and their applications. Wellcome Open Res. 2018;3:124. doi:10.12688/wellcomeopenres.14826.1.