ABSTRACT
Multiple types of SARS-CoV-2 vaccines have been used worldwide, but summarizing their immunologic efficacy post-vaccination remains challenging. The BCR and TCR sequencing based on single-cell sorting makes it possible to evaluate the vaccine-induced immune responses of B or T cells. In this study, we compared the repertoire diversities of B cells and T cells between a whole-virus inactivated vaccine and an S1 protein subunit vaccine in rhesus macaques. We found that the inactivated vaccine could induce a large antigen-specific-BCR repertoire with longer VH CDR3 (21 aa), while the CD3+ TCR α chains of the two vaccine groups showed a similar TCRV/J usage frequency. Detailed analysis of the TCR and BCR repertoires might be of interest for further understanding of the mechanisms of vaccine-induced immune responses.
Introduction
SARS-CoV-2 has spread rapidly around the world, with more than 572 million confirmed cases globally and a cumulative death toll of 6 million.Citation1 The rapid development of vaccines, including inactivated vaccines, mRNA vaccines, viral vector vaccines, and subunit vaccines, has helped reduce the number of severe COVID-19 cases.Citation1–4 However, current research shows that the level of neutralizing antibody titers are decreasing in vaccinated populations and that the number of recorded cases of breakthrough SARS-CoV-2 infection is increasing, especially as we face an increasing number of SARS-CoV-2 variants.Citation5 In this situation, it is very important to explore the immune response mechanism of vaccines to support for the rational design and deployment of vaccines, and to scientifically and effectively control COVID-19.
Different types of vaccines can induce divergent immune response characteristics in vivo.Citation6 However, we cannot obtain enough information on the genetic and functional properties of antigen-specific antibodies from overall serum binding and/or neutralization tests.Citation7 Benefiting from non-human primates (NHPs) investigations of high-affinity, cross-reactive antibodies at the epitope level,Citation7,Citation8 we performed single-cell clonal expansion of memory B cells and T cells repertoire to decode adaptive immune responses in two groups of rhesus monkeys vaccinated with SARS-CoV-2 inactivated vaccine or spike protein subunit vaccine.
Results
Preparation of the experimental SARS-CoV-2 vaccine
The SARS-CoV-2 strain (SARS-CoV-2-KMS1/2020, GenBank No: MT226610.1) used in this study was isolated from sputum collected from an adult COVID-19 patient at the Institute of Medical Biology (IMB), Chinese Academy of Medical Sciences (CAMS).Citation9 Both the inactivated SARS-CoV-2 vaccine and the S1 subunit vaccine were developed by IMB. The viral seed strain for developing the inactivated vaccine was inoculated into a monolayer of Vero cells in BSL3 facility. Inactivation of the viral harvest was performed with formaldehyde (HCHO, 1:4,000, 72 h) treatment, followed by an ultrafiltration-mediated concentration step and purification by column chromatography. The bulk of the inactivated vaccine was emulsified in 0.5 mg/dose Al(OH)3 adjuvant to constitute the final vaccine product at a dosage of 150 U/0.5 ml Citation3(). The viral antigen in one dose of inactivated vaccine contained 5 µg S protein. Meanwhile, the S1 subunit vaccine was designed as full-length S1 domain of spike protein expressed by ferretin-pCDNA3.1+ vector in 293T cell system (). The fusion protein nanoparticles were purified by affinity chromatography using Ni-NTA agarose.Citation10 The subunit vaccine contained a dose of 15 µg S1 antigen (0.5 ml/dose) and 0.5 mg Al(OH)3 (). Antigen protein in both vaccines were verified by western blotting and mass-spectrum analysis.
Figure 1. The study design, neutralizing antibody response and single B/T-cell sorting. All animal experiments were approved by the Institutional Animal Care and Use Committee (IACUC) of IMB, CAMS. Before starting the study, it was confirmed that the all monkeys did not carry anti-SARS-CoV-2 antibodies. Blood samples were collected from the animals at 14 days after the boost immunization and before the primary immunization. The serum and PBMCs isolated from the whole blood samples were stocked for the neutralizing antibody (NAb) titer, ELISpot assays and single T-cell and B-cell sorting experiments. (a) Scheme for immunization of rhesus macaques with two doses of inactivated vaccine or subunit vaccine. (b) the animal serum neutralizing titers were determined by standard microneutralization assays. The SARS-CoV-2 strain (SARS-CoV-2-KMS1/2020, GenBank No: MT226610.1) was used as the challenging virus of the standard microneutralization assays. Briefly, the heat-inactivated serum from macaques was serially diluted and incubated with SARS-CoV-2 live virus (100CCID50) for 2 hours at 37°C. Then, 100 μl of the Vero cell suspension (105 cells/ml) was added to the virus-serum mixture. The 96-well plates contained virus-serum mixture was then incubated at 37°C for 7 days. Neutralization Ab titers of every monkey from subunit vaccine group (n = 7) or inactivated vaccine group (n = 8) were detected after two doses of vaccine-inoculation. The values for the neutralizing antibodies are shown as GMT with 95%CI. The data came from three replicates experiments. (c) Comparison of average NAb titers to SARS-CoV-2 between the subunit vaccine (n = 7) and inactivated vaccine groups(n = 8). The values for the neutralizing antibodies are shown as GMTs with 95%CI. The t test was used for the neutralization titers assay. ****: p < .0001. (d) Antigen-specific IFN-γ response to SARS-CoV-2, as determined by ELISpot assay after two doses of vaccine-inoculation according to the manufactures’ protocols. The stimulated peptide mixtures used in the ELISpot assay covered the S1, S2 and N regions. The spot-forming cells (SFCs) per 10^6 PBMCs are counted by an ELISpot plate reader. The value of each column come from three monkeys with highest neutralizing antibody titers in each vaccine group. The Blank represents the PBMC isolated from these three monkeys before the primary immunization. The values are represented by mean±sd. The t test was used for the ELISpot assay. *:p < .05, **: p < .01,****: p < .0001. (e) for single cell-sorting, we labeled the SARS-CoV-2 S or N protein as SARS-CoV-2 S/N-PE antibody according to the PE Lightning-Link Conjugation Kit instructions. Then, PBMCs were stained with 100 μl of PBS containing a mixture of antibodies against anti-CD20-FITC, anti-CD27-APC, and SARS-CoV-2 N/S-PE for SARS-CoV-2-specific memory B cells sorting, or stained with a mixture of antibodies against anti-CD3-BV421 and SARS-CoV-2 N/S-PE for SARS-CoV-2-specific CD3+T cells sorting. After incubation for 30 minutes at room temperature in the dark. Finally, the SARS-CoV-2 memory B cells (CD20+CD27+ S/N protein+) (left panel)and SARS-CoV-2-specific CD3+ T cells (CD3+ S/N protein+)(right panel) were sorted as single cells by a BD FACSJazz cell sorter into 96-well plates containing 20 µl of cell lysis buffer. D0: The day before the primary immunization. D142st: 14 days after the boost immunization.
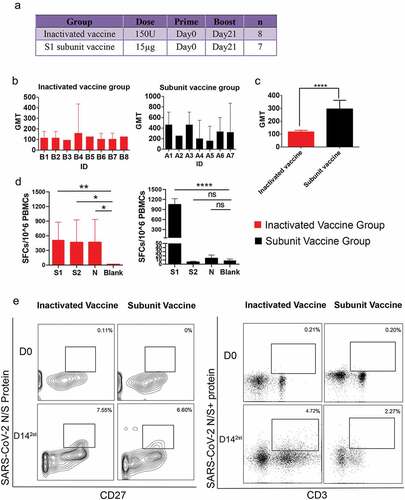
The immune response to the experimental vaccine and SARS-CoV-2-specific T-cell and B-cell sorting
In our study, 15 healthy male Chinese rhesus monkeys (Macaca mulatta) (age: 6 month; weight: 2–3 kg) were randomly divided into two groups: an inactivated vaccine group(n = 8) and a SARS-CoV-2 spike S1 subunit vaccine group (n = 7). The two groups of animals were both immunized intramuscularly on days 0 and 21 (). The results showed that both groups achieved good neutralizing antibody (NAb) levels on 14th day post after two doses of inactivated vaccine or subunit vaccine, with geometric mean titers (GMTs) of 96~161.3 and 161.3~465.2 against the original SARS-CoV-2 strain (). The GMT of NAb titers of subunit vaccine group is higher than the inactivated vaccine group (). Based on the NAb response level, we selected the three monkeys with the higher GMT from each group for T/B-cell receptor repertoire analysis, including monkeys with GMTs of 161.3 (B4), 128 (B5), 128 (B8) for the inactivated vaccine () and monkeys with GMTs of 465.2 (A1), 465.2 (A3), and 335.5 (A6) of the subunit vaccine group (). To evaluate the SARS-CoV-2-specific cellular response, we performed an IFN-γ ELISpot assay on PBMC samples collected from two groups of three vaccine-immunized rhesus monkeys with highest neutralizing antibody titers (). We found specific T-cell IFN-γ response in inactivated vaccine-immunized monkeys could be elicited by the SARS-CoV-2 S1, S2, and N structural proteins. While in subunit-immunized group, only the SARS-CoV-2 S1 peptides effectively simulated a specific T-cell IFN-γ response (). The above results suggest that the inactivated vaccine can induce a broader antigen-specific T cell response than the subunit vaccine.
Then, we sorted PBMC pools from three donor monkeys of each vaccine group by fluorescence activated cell sorting (FACS). The SARS-CoV-2 S and N protein mixtures were used to screen SARS-CoV-2-specific B cells and antigen-specific CD3+ T cells. At 14 days after the boost immunization, a population of memory B cells (CD20+CD27+N/S protein+) was clearly identified as 7.55% and 6.60% of the total CD20+ B cells in the inactivated vaccine group and subunit vaccine group, respectively (). At the same time, a population of T cells (CD3+N/S protein+) was clearly identified as 4.72% and 2.27% of the total PBMC cells in the inactivated vaccine group and subunit vaccine group, respectively (). These results indicated both types of vaccine can elicit humoral and cellular immune responses after two dose immunization.
Sequence analysis of vaccine-induced B-cell and T-cell repertoire
The B-cell repertoire (BCR) immunoglobin heavy chain (IGH) genes of SARS-CoV-2 specific B sorted from different vaccines were amplified from the cDNA by 2 rounds of nested PCR according to a previously established method.Citation11,Citation12 The PCR products were evaluated on 2% agarose gels, and the positives (bands 300~500 bp for IGH gene) were sequenced. The IGH VDJ genes and the CDR3 length were analyzed using IgBLAST (https://www.ncbi.nlm.nih.gov/igblast/). We obtained 59/192 wells of positives in inactivated vaccine group, while 43/242 wells of positives in subunit vaccine group. To evaluate the change in IGH gene frequency of the COVID-19 vaccine-immune rhesus macaques, we calculated the distribution of the IGHV, IGHD, IGHJ, and VH complementarity-determining-region 3(CDR3)gene subgroups in the two groups. We observed that IGHV1-69 * 06 accounted for 25.4% of the total subgroups, followed by IGHV3-21 * 06 (13.6%), IGHV4-59 * 12 (11.9%), and IGHV4-38-2 * 01 (10.2%) in the inactivated vaccine group, while the top IGHV genes were IGHV1-69 * 06 (41.9%), IGHV4-59 * 12 (14.0%), IGHV4-34 * 10 (11.6%), and IGHV3-38 * 01 (9.3%) in the subunit vaccine group (). Our data showed a high level of IGHV1 and IGHV4 expression in both vaccine groups. Compared with the nine of IGHV genes in the subunit vaccine group, the inactivated-vaccine group possessed more kinds of IGHV genes (n = 18) (). For the IGHD and IGHJ distribution, the IGHD5-12 * 01 and IGHJ4 * 03 is the representative germline in the inactivated vaccine group (). However, the IGHD1-14 * 01 and IGHJ6 * 02 is the represented germline in the subunit vaccine group (). Further analysis showed that the top IGHV/J gene pair, IGHV1-69 * 06/IGHJ4 * 03 and IGHV1-69 * 06/IGHJ6 * 02 was present in inactivated vaccine group and subunit vaccine group, respectively (). In addition, the heavy-chain CDR3 sequences of inactivated-vaccine groups contained 9–21 aa with a peak of 37.29% cells at 18 aa and 1.69% of long VH CDR3 (21aa) (); however, the CDR3 lengths of the subunit vaccine groups were no more than 19 aa ().
Figure 2. The gene characteristics of BCR IGH in the two vaccine groups. (a,b) IGH-V, IGH-D and IGH-J domain gene distributions for single SARS-CoV-2-specific memory B cells in the (a) inactivated vaccine group and (b) subunit vaccine group. (c) the usage frequencies of all IGH-V genes (y axis) and IGH-J genes (× axis) amplified from single viral antigen-specific memory B cells from the inactivated vaccine group (red dots) or subunit vaccine group (black dots). Point size shows the frequency (100%) of a V-J pairing. (d) The CDR3 AA length distribution of VH for the two vaccine groups.
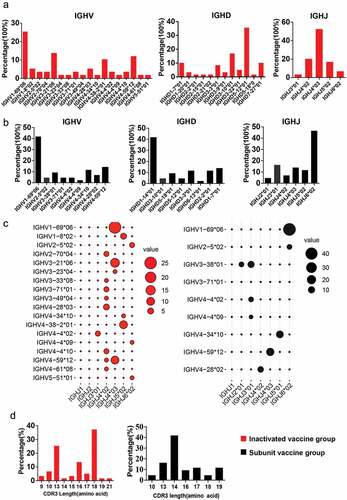
The T-cell repertoire (TCR) αβ genes were amplified by two rounds of nested PCR, and the sequences of positives (bands 200~500 bp),Citation13,Citation14 including TRAV, TRAJ or TRBV, TRBD, and TRBJ genes and CDR3 length, were analyzed using IMGT (http://www.imgt.org/ IMGT_vquest/analysis). We found 46/364 wells TCR α- or β-chain gene amplifications in inactivated vaccine group. The TCR α chain accounted for 28 wells and β chain accounted for 18 wells. In subunit vaccine group, 75/288 wells were positive for TCR α- or β-chain gene amplification, in which α chain accounted for 46 wells, and β chain accounted for 29 wells. The V-(D)-J and CDR3 sequences obtained from the TCR α/β chains were further investigated to interpret the T-cell immune characteristics of immunized monkeys. From the CDR3 length of TCRα, we observed 32% of cells with 11 aa, 28% of cells with 12 aa, and 8% of cells with 14 aa in the inactivated-vaccine group (distributing of 8~14 aa). The subunit vaccine group displayed a CDR3 length of 7 to 14 aa, with 56.8% of cells with 12 aa (). For the TCRβ chain, 62.5% of CDR3 length and 75% of CDR3 length were approximately 12 to 13 aa in inactivated-vaccine group and subunit vaccine group, respectively, while a 10aa length of CDR3 was found more frequency in the inactivated vaccine group (). The TRAV6 gene segment and TRAJ42 and CDR3 (ALIDGGSRGNLI) gene segment pairings exhibited higher usage in the TCRα chains of both inactivated vaccine and subunit vaccine donors (), while there was no obvious bias of the TRBV-J-CDR3 gene segment pairings in the TCRβ chains of the two vaccine groups ().
Figure 3. The gene characteristics of TCRαβ chains in the two vaccine groups. (a,b) the CDR3 AA length distribution of TCRαβ chains for the two vaccine groups. (c) The diagram shows COVID-19-specific TCRα chain V-J gene pairs in the two vaccine groups. The first, second and third columns represent TRAV, TRAJ, and CDR3, respectively. (d) The first to fourth columns represent the TRBV, TRBD, TRBJ, and CDR3, respectively. The area of the colors of each column (from left to right) was proportional to the frequency of the V, D, J or CDR3 gene sequence in all TCR clones from the two vaccine groups. The paired V-(D)-J with CDR3 genes are connected by smooth curves. The thickness of a line shows the frequency (100%) of a V-(D)-J-CDR3 pairing.
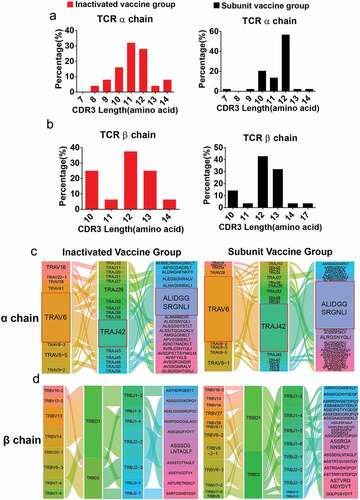
Conclusions and discussion
Recently, TCR and BCR repertoires have been widely applied to evaluate the mechanisms of the immune response to many kinds of vaccines, including the HBV protein vaccine,Citation15 influenza vaccine,Citation16 and SARS-CoV-2 vaccine.Citation17 A recent clinical study defined specific TCR and BCR sequences associated with different COVID-19 disease courses by next-generation sequencing.Citation18 Another study reported the difference in BCR/TCR signal transduction and B/T-cell activation between the protein subunit (PS) vaccine ZF2001 and an mRNA vaccine named RRV by transcriptomic analysis.Citation19 However, there is incomplete knowledge about the nature of variant T-cell and B-cell immune responses induced by SARS-CoV-2 inactivated vaccines and protein subunit vaccines, which have been widely used in China. This knowledge is urgently needed to inform trial design and strategic vaccine development. Since the NHP models are widely used in evaluating the preclinical immune effectiveness of SARS-CoV-2 vaccines,Citation2,Citation3,Citation9,Citation10,Citation20,Citation21 investigation of TCRs and BCRs during vaccination of NHPs can play an important role in the guidance of SARS-CoV-2 vaccine development and serve as a translational bridge to the clinical research.Citation8
IGH germline and TCR germline elicited to SARS-CoV-2 infection could imply the immune response of host recovered from COVID-19. It was reported that the IGHV3 BCR clusters are preferentially expressed in individuals with COVID-19.Citation22 The TCR germline features in convalescent patients and healthy donors were also revealed that TRAV12–1 and TRBV7–9 were used by 71% and 16% of YLQ-specific TCRs, and TRAV13–2 and TRBV6–5 were used by 15% and 25% of RLQ-specific TCRs, respectively, compared with just 3%–4% gene usage in control TCRs.Citation17 In our study on vaccine induced B cell response, we found that IGHV1 and IGHV4 are the major IGH germlines in the subunit vaccine and inactivated vaccine groups. While in TCR analysis, TRAV6 gene segment and TRAJ42 and CDR3 (ALIDGGSRGNLI) gene segment pairings exhibited higher usage in the TCRα chains of both inactivated vaccine and subunit vaccine animals. Whereas differences in the peptide-specific antigen responses induced by the two kinds of vaccines according to the ELISpot assay results, whether germline-encoded features are involved in TCR recognition of these SARS-CoV-2 peptides remains to be further clarified in the future.
To date, the SARS-CoV-2 spike protein has been considered as the major immunogen for vaccine development, but many studies are shifting their emphasis to multiple viral antigens for comprehensive immune protection. Typically, in the situation of Variants of Concern (VOC) transmission, spike mutations may abrogate immune protection elicited by S subunit vaccine.Citation5 We believed that whole inactivated virus with an abundance of viral antigens can not only target spike neutralizing antibodies but also maintain potency for inducing the T/B cell responses.Citation5 The diversity of an antigen-specific TCR/BCR repertoire is suggested as an index for T/B cell clonal populations elicited by infection or vaccination.Citation18–Citation23–25 On the basis of the polymorphic receptors expressed by B cells and T cells induced by different immunogens, we found that BCR germline IGHV1-69 * 06 and TCR germline TRAV6-J42 with CDR3 (ALIDGGSRGNLI) were used in the inactivated vaccine and protein subunit vaccine groups. One article showed that human IGHV1–69 B-cell receptors imparted natural affinity for a “universal” vaccine target, enabling rapid bNAb responses in mice that were elicited using a rationally designed influenza immunogen.Citation26 However, whether IGHV1–69 belongs to the public lineage of influenza and SARS-CoV-2 infection remains to be furtherly studied. Notably, the inactivated vaccine can induce an abundant antigen-specific-BCR repertoire compared with the subunit vaccine, with longer CDR3 sequences (19–21 aa in length), may be relevant to Ab specificity and cross-reactivity.Citation27,Citation28 Following IFN-γ ELISpot assay revealed that the cellular immune response elicited by the subunit vaccine was concentrated on the S1 protein. However, the inactivated vaccine could widely elicit S1-, S2- and N-specific cellular immune in limited single-cell sorting samples. Whether this broad cellular immune response can affect the evolution of the TCR lineage is worth furtherly investigating.
Although the conclusion of this study comes from a small single-cell sorting sample, we aimed to provide information and a reference to help understand the TCR/BCR responsiveness to inactivated and subunit vaccines. In this context, we just choose three rhesus macaques with the highest neutralizing antibody titers from each group to analysis. Therefore, our study includes a representative sample. In summary, in addition to neutralizing antibody titers, the frequencies and sequences of specific T/B-cell repertoires are also critical for evaluating the protection induced by candidate vaccines.
Authors’ contributions
HZ and MS conceptualized the study; HZ, MS and SL wrote the manuscript; LQ, YZ, and SL performed the experiments and analyzed the results; BL, HW, HL, JL, and YC helped to perform the animal experiments.
Acknowledgement
We thank the Kunming National High-level Biosafety Primate Research Center for its support.
Disclosure statement
No potential conflict of interest was reported by the author(s).
Correction Statement
This article has been corrected with minor changes. These changes do not impact the academic content of the article.
Additional information
Funding
References
- WHO. WHO Coronavirus (COVID-19) dashboard; 2022 [ accessed 2022 Aug 04]. https://covid19.who.int/.
- Feng L, Wang Q, Shan C, Yang C, Feng Y, Wu J, Liu X, Zhou Y, Jiang R, Hu P, et al. An adenovirus-vectored COVID-19 vaccine confers protection from SARS-COV-2 challenge in rhesus macaques. Nat Commun. 2020;11(1):1. doi:10.1038/s41467-020-18077-5.
- Chen H, Xie Z, Long R, Fan S, Li H, He Z, Xu K, Liao Y, Wang L, Zhang Y, et al. Immunological evaluation of an inactivated SARS-CoV-2 vaccine in rhesus macaques. Mol Ther Methods Clin Dev. 2021;23:108–7. doi:10.1016/j.omtm.2021.08.005.
- Bettini E, Locci M. SARS-CoV-2 mRNA vaccines: immunological mechanism and beyond. Vaccines (Basel). 2021;9. doi:10.3390/vaccines9020147.
- Cromer D, Juno JA, Khoury D, Reynaldi A, Wheatley AK, Kent SJ, Davenport MP. Prospects for durable immune control of SARS-CoV-2 and prevention of reinfection. Nat Rev Immunol. 2021;21(6):395–404. doi:10.1038/s41577-021-00550-x.
- Baxter D. Active and passive immunity, vaccine types, excipients and licensing. Occup Med (Lond). 2007;57(8):552–556. doi:10.1093/occmed/kqm110.
- Sundling C, Phad G, Douagi I, Navis M, Karlsson Hedestam GB. Isolation of antibody V(D)J sequences from single cell sorted rhesus macaque B cells. J Immunol Methods. 2012;386:85–93. doi:10.1016/j.jim.2012.09.003.
- Meng W, Li L, Xiong W, Fan X, Deng H, Bett AJ, Chen Z, Tang A, Cox KS, Joyce JG, et al. Efficient generation of monoclonal antibodies from single rhesus macaque antibody secreting cells. Mabs. 2015;7(4):707–718. doi:10.1080/19420862.2015.1051440.
- Zheng H, Li H, Guo L, Liang Y, Li J, Wang X, Hu Y, Wang L, Liao Y, Yang F, et al. Virulence and pathogenesis of SARS-CoV-2 infection in rhesus macaques: a nonhuman primate model of COVID-19 progression. PLoS Pathog. 2020;16(11):e1008949. doi:10.1371/journal.ppat.1008949.
- Li H, Guo L, Zheng H, Li J, Zhao X, Li J, Liang Y, Yang F, Zhao Y, Yang J, et al. Self-assembling nanoparticle vaccines displaying the receptor binding domain of SARS-CoV-2 elicit robust protective immune responses in Rhesus monkeys. Bioconjug Chem. 2021;32(5):1034–1046. doi:10.1021/acs.bioconjchem.1c00208.
- Zheng H, Yang Z, Li B, Li H, Guo L, Song J, Hou D, Li N, Yang J, Wu Q, et al. Single B cells reveal the antibody responses of rhesus macaques immunized with an inactivated enterovirus D68 vaccine. Arch Virol. 2020;165(8):1777–1789. doi:10.1007/s00705-020-04676-6.
- Zheng H, Wang J, Li B, Guo L, Li H, Song J, Yang Z, Li H, Fan H, Huang X, et al. A novel neutralizing antibody specific to the DE loop of VP1 can inhibit EV-D68 infection in mice. J Immunol. 2018;201(9):2557–2569. doi:10.4049/jimmunol.1800655.
- Dash P, Wang GC, Thomas PG. Single-cell analysis of T-cell receptor alphabeta repertoire. Methods Mol Biol. 2015;1343:181–197.
- Sun X, Saito M, Sato Y, Chikata T, Naruto T, Ozawa T, Kobayashi E, Kishi H, Muraguchi A, Takiguchi M. Unbiased analysis of TCRα/β chains at the single-cell level in human CD8+ T-cell subsets. PLoS One. 2012;7(7):e40386. doi:10.1371/journal.pone.0040386.
- Miyasaka A, Yoshida Y, Wang T, Takikawa Y. Next-generation sequencing analysis of the human T-cell and B-cell receptor repertoire diversity before and after hepatitis B vaccination. Hum Vaccin Immunother. 2019;15(11):2738–2753. doi:10.1080/21645515.2019.1600987.
- Yang X, Chen G, Weng NP, Mariuzza RA. Structural basis for clonal diversity of the human T-cell response to a dominant influenza virus epitope. J Biol Chem. 2017;292(45):18618–18627. doi:10.1074/jbc.M117.810382.
- Shomuradova AS, Vagida MS, Sheetikov SA, Zornikova KV, Kiryukhin D, Titov A, Peshkova IO, Khmelevskaya A, Dianov DV, Malasheva M, et al. SARS-CoV-2 epitopes are recognized by a public and diverse repertoire of human T cell receptors. Immunity 2020, 53:1245–1257 e1245.
- Cao Y, Su B, Guo X, Sun W, Deng Y, Bao L, Zhu Q, Zhang X, Zheng Y, Geng C, et al. Potent neutralizing antibodies against SARS-CoV-2 identified by high-throughput single-cell sequencing of convalescent patients. B Cells Cell. 2020;182:73–84 e16.
- Wang Q, Song Z, Yang J, He Q, Mao Q, Bai Y, Liu J, An C, Yan X, Cui B, et al. Transcriptomic analysis of the innate immune signatures of a SARS-CoV-2 protein subunit vaccine ZF2001 and an mRNA vaccine RRV. Emerg Microbes Infect. 2022;11(1):1145–1153. doi:10.1080/22221751.2022.2059404.
- Garrido C, Curtis AD, Dennis M, Pathak SH, Gao H, Montefiori D, Tomai M, Fox CB, Kozlowski PA, Scobey T, et al. SARS-CoV-2 vaccines elicit durable immune responses in infant rhesus macaques. Sci Immunol. 2021;6(60). doi:10.1126/sciimmunol.abj3684.
- Zheng H, Chen Y, Li J, Li H, Zhao X, Li J, Yang F, Li Y, Liu C, Qin L, et al. Longitudinal analyses reveal distinct immune response landscapes in lung and intestinal tissues from SARS-CoV-2-infected rhesus macaques. Cell Rep. 2022;39(8):110864. doi:10.1016/j.celrep.2022.110864.
- Schultheiss C, Paschold L, Simnica D, Mohme M, Willscher E, von Wenserski L, Scholz R, Wieters I, Dahlke C, Tolosa E, et al. Next-generation sequencing of T and B cell receptor repertoires from COVID-19 patients showed signatures associated with severity of disease. Immunity. 2020;53(2):442–455 e444. doi:10.1016/j.immuni.2020.06.024.
- Hou D, Chen C, Seely EJ, Chen S, Song Y. High-throughput sequencing-based immune repertoire study during infectious disease. Front Immunol. 2016;7:336. doi:10.3389/fimmu.2016.00336.
- Luo L, Liang W, Pang J, Xu G, Chen Y, Guo X, Wang X, Zhao Y, Lai Y, Liu Y, et al. Dynamics of TCR repertoire and T cell function in COVID-19 convalescent individuals. Cell Discov. 2021;7(1):89. doi:10.1038/s41421-021-00321-x.
- Zollner A, Watschinger C, Rossler A, Farcet MR, Penner A, Bohm V, Kiechl SJ, Stampfel G, Hintenberger R, Tilg H, et al. B and T cell response to SARS-CoV-2 vaccination in health care professionals with and without previous COVID-19. EBioMedicine. 2021;70:103539. doi:10.1016/j.ebiom.2021.103539.
- Sangesland M, Ronsard L, Kazer SW, Bals J, Boyoglu-Barnum S, Yousif AS, Barnes R, Feldman J, Quirindongo-Crespo M, McTamney PM, et al. Germline-encoded affinity for cognate antigen enables vaccine amplification of a human broadly neutralizing response against influenza virus. Immunity. 2019;51(4):735–749 e738. doi:10.1016/j.immuni.2019.09.001.
- D’Angelo S, Ferrara F, Naranjo L, Erasmus MF, Hraber P, Bradbury ARM. Many routes to an antibody heavy-chain CDR3: necessary, yet insufficient, for specific binding. Front Immunol. 2018;9:395. doi:10.3389/fimmu.2018.00395.
- Barrios Y, Jirholt P, Ohlin M. Length of the antibody heavy chain complementarity determining region 3 as a specificity-determining factor. J Mol Recognit. 2004;17(4):332–338. doi:10.1002/jmr.679.