ABSTRACT
Persistence of an immunosuppression, affecting both the innate and adaptive arms of the immune system, plays a role in sepsis patients’ morbidity and late mortality pointing to the need for broad and effective immune interventions. MVA-hIL-7-Fc is a non-replicative recombinant Modified Vaccinia virus Ankara encoding the human interleukin-7 fused to human IgG2 Fc fragment. We have shown in murine sepsis models the capacity of this new virotherapy to stimulate both arms of the immune system and increase survival. Herein, an exploratory study in nonhuman primates was performed following a single intravenous injection of the MVA-hIL-7-Fc used at the clinical dose to assess its safety and biological activities. Four cynomolgus macaques were followed for 3 weeks post-injection (p.i), without observed acute adverse reactions. Circulating hIL-7-Fc was detected during the first 3–5 days p.i with a detection peaking at 12 h p.i. IL-7 receptor engagement and downstream signal transduction were detected in T cells demonstrating functionality of the expressed IL-7. Expansion of blood lymphocytes, mainly CD4 and CD8 naïve and central memory T cells, was observed on day 7 p.i. together with a transient increase of Ki67 expression on T lymphocytes. In addition, we observed an increase in circulating B and NK cells as well as monocytes were albeit with different kinetics and levels. This study indicates that a vectorized IL-7-Fc, injected by intravenous route at a relevant clinical dose in a large animal model, is active without adverse reactions supporting the clinical development of this novel virotherapy for treatment of sepsis patients.
Introduction
Interleukin-7 (IL-7) plays a critical role in the development and function of lymphoid cells. This cytokine is involved in regulating the development of T, B, natural killer (NK) and dendritic cells. In addition, it plays a role in homeostasis of mature lymphocyte subsets by inducing cell proliferation and promoting cell survival.Citation1 IL-7 signal is transduced by the IL-7 receptor, a heterodimer composed of two chains of IL-7 Rα (CD127) and the common γ chain of IL-2, IL-9, IL-15 and IL-21.Citation2 Whereas γ chain is expressed by most hematopoietic cells, IL-7 Rα is nearly exclusively expressed on lymphoid cells. IL-7 Rα is expressed on naïve T-cells, downregulated upon activation of IL-7-mediated signaling and re-expressed in memory T-cells.Citation3,Citation4 This leads to activation of JAK1/3, which activates many downstream signaling pathways mainly including STAT5, PI3 and SRC kinases.
Given its ability to prevent lymphocyte apoptosis, induce lymphocyte proliferation and improve lymphocyte function, IL-7 has been tested as a molecular adjuvant in multiple vaccines,Citation5 but also as a host directed therapeutic (HDT) in diseases associated with lymphopenia and lymphocyte exhaustion. Numerous clinical trials have shown that IL-7 therapy is well tolerated with no severe toxicities reported. They have confirmed the capacity of recombinant soluble hIL-7 to restore T lymphocytes in cancer patients,Citation6–8 patients with chronic HIV infection,Citation9,Citation10 bacterial sepsisCitation11,Citation12 or COVID-19.Citation13–15
In preclinical nonhuman primate models, particularly of simian immunodeficiency virus (SIV) infection, recombinant simian and human IL-7 proteins improve immune reconstitution and increase the number of circulating CD4 and CD8 T cells.Citation16–19 IL-7 treatment has been described to counteract IFN-α treatment-induced lymphopenia.Citation20 It enhances CD4 reconstitution following autologous CD34+ selected stem cell transplantation in monkeys.Citation21 The soluble cytokine has also been shown to increase not only peripheral naïve T cells, but also central memory T cells in SIV infected and aged primates.Citation3,Citation22,Citation23
Due to its short circulating half-life, multiple successive injections of high amounts of soluble recombinant IL-7 protein have typically been used in the above-mentioned studies. Viral-based delivery vectors may offer an alternative to the injection of soluble cytokines and bypass some limitations observed with such formulations. Viral vectors have been extensively used in the development of gene therapies and vaccines.Citation24 They can deliver the engineered genes to a variety of organs and related cells, resulting in robust in vivo production of the encoded proteins. Those are directly engineered by the host’s cell machinery typically in a native form. In addition, viral vectors can directly stimulate the immune system by engaging innate immune-pathways and therefore contribute to immune restoration. Some IL-7-encoding viral vectors have been evaluated in preclinical models as prophylactic or therapeutic vaccines.Citation25–27 In these vaccines, the encoded IL-7 is used as an adjuvant to increase vaccine-encoded immunogen-specific immunity. In these settings, dosage of the expressed IL-7 (concentration and duration) and specific pathways engaged by the cytokine as well as effects on immune cell populations have not been described to our knowledge in nonhuman primates. More globally, the use of IL-7-vectors in the development of HDT is not reported in NHP.
To restore immune-homeostasis, particularly in immune-depressed sepsis patients, we have developed a novel IL-7-based virotherapyCitation28 using the non-replicative Modified Vaccinia virus Ankara (MVA). This powerful and safe viral platform harbors an intrinsic capacity to stimulate innate immunity.Citation29–31 The generated recombinant MVA, MVA-hIL-7-Fc, encodes the human IL-7 fused to the human IgG2-Fc to increase in vivo half-life of the cytokine.Citation32 In naïve and sepsis mouse models, we have shown that a single intravenous injection of MVA-hIL-7-Fc results in long-lasting IL-7-Fc detection in blood.Citation28 When compared with the hIL-7-Fc soluble counterpart protein, the MVA-hIL-7-Fc was shown to typically display broader, more robust, and longer-lasting immune activities including a superior increase of total and activated B, T, NK and myeloid cells and of functional T-cells producing multiple cytokines in both spleens and lungs of treated mice.Citation28 The MVA-hIL-7-Fc confers a significant survival advantage in both the cecal-ligation-puncture and Candida albicans sepsis models.Citation28
Here, we evaluated MVA-hIL-7-Fc at a relevant clinical dose in a large animal model. Since human IL-7 is known to be active in monkey,Citation3,Citation16 cynomolgus macaques were treated with a single intravenous (IV) injection of the recombinant MVA and clinical, hematological and biochemical parameters were assessed to obtain preliminary safety data. The pharmacokinetics and functionality of the expressed hIL-7-Fc were analyzed. In addition, effect of MVA-hIL-7-Fc on the absolute circulating lymphocyte count was evaluated, particularly on CD4 and CD8 naïve and memory T cell populations. This exploratory proof-of-activity study represents the first evaluation a vectorized IL-7 in nonhuman primates.
Material and method
Animals and ethics
The study was performed at the Biomedical Primate Research Center (BPRC, Rijswijk, The Netherlands). The BPRC is accredited by the American Association for Accreditation of Laboratory Animal Care (AAALAC) and is compliant with European directive 2010/63/EU for animal experiments which guidelines have been followed. The present study was approved by the central Dutch authorities for animal experiments (Centrale Commissie Dierproeven, CCD), dossier number AVD5020020209404 (CCD.028). The study protocol was approved prior to start by the local animal welfare body of BPRC (Instantie voor Dierwelzijn, IvD), dossier number CCD.028C.
Four healthy adult female cynomolgus macaques (Macaca fascicularis) with weight over 5 kg were selected from BPRC breeding colonies. Animals were socially housed in pairs, they were offered a daily diet consisting of monkey food pellets (Hope Farms, Woerden, The Netherlands), fruit and vegetables of the season and bread. Drinking water was available ad libitum via automatic water systems. Animal handling and biosampling was performed under a combination of ketamine (ketamine hydrochloride, ketamine 10%; Alfasan Nederland B.V., Woerden, NL, 100 mg/mL, 10 mg/kg) and medetomidine (medetomidine hydrochloride, Sedastart®; AST Farma B.V., Oudewater, The Netherlands, 1 mg/mL, 0.05 mg/kg). After procedure, animals received atipamezole hydrochloride (Sedastop®, ASTFarma B.V., Oudewater, The Netherlands, 5 mg/mL, 0.25 mg/kg) allowing a quick recovery from their sedation.
MVA-hIL-7-Fc treatment
MVA-hIL-7-Fc engineering, production and characterization were previously described.Citation28 Briefly, MVA-hIL-7-Fc is based on the highly attenuated Modified Vaccinia virus Ankara (MVA) encoding for the fusion of the human IL-7 sequence and the sequence of the human IgG2 Fc. The recombinant MVA was generated in primary Chicken Embryo Fibroblasts, purified, and titrated. The maximal expected clinical dose to be used in the future FIM trial is 109 pfu MVA-IL7-Fc. Based on FDA guidelines,Citation33 the animal equivalent dose for monkeys was calculated at 5.107 pfu/kg. Hence, sedated animals received a single intravenous injection via the vena saphena of 5.107 pfu/mL of MVA-hIL7-Fc in buffer (Tris 10 mM, saccharose 5%, Sodium glutamate 10 mM, Sodium chloride 50 mM, pH8).
Evaluation of clinical, hematologic and biochemical parameters
Body temperature, body weight, heart rate and breathing rate were measured at all blood collected time points. Blood was collected in EDTA tubes by venipuncture before MVA injection and 2, 4, 6, 12 hours, 1, 2, 3, 4, 5, 7, 14, 21 days post-injection. Collected blood volume was adapted to the type of analysis at each time point to respect ethical limits.
Clinical chemistry and hematologic analysis were performed on sera collected before and 6 hours, 1, 2, 7, 14, 21 days post MVA injection. Clinical chemistry (ALAT, ASAT, Albumin, Alkaline phosphatase, Bilirubin, Cholesterol, Chloride, Creatinine, α-Glutamyl Transferase, Glucose, LDH, Bicarbonate, Calcium, Sodium, Iron, Potassium, Phosphate, C-reactive protein, Total protein, Urea) was analyzed on a Cobas Integra-400+ (Roche, Almere, The Netherlands). Hematologic analysis (Absolute Leukocyte Counts (White Blood Cell, Lymphocytes, Neutrophils, Monocytes, Eosinophil, Basophil), Erythrocyte count and associated parameters, Mean Corpuscular Volume, Hemoglobin, Hematocrit, Platelets count and associated parameters were conducted on Sysmex Sf-3000 (Goffin Meyvis, The Netherlands).
Determination of hIL-7-Fc serum concentration and pharmacokinetics analysis
For pharmacokinetics analysis, serial blood samples were collected before and 2, 4, 6, 12 hours, 1, 2, 3, 4, 5, 7 days after MVA-hIL-7-Fc injection. The concentration of hIL-7-Fc in frozen sera was determined using the human IL-7 DuoSet® ELISA Development System from R&D Systems according to the provider’s instructions. The hIL-7-Fc concentration of each sample was calculated using a standard curve established with a recombinant hIL-7-Fc protein, the exact counterpart of the MVA-expressed hIL-7-Fc (produced on CHO cells by Geneart (Regensburg, Germany)).Citation28 The area under the serum concentration vs. time curve (AUC) was calculated with Prism 9.0 software (GraphPad Software, LLC) using the trapezoid rules.
Quantification of circulating cytokines and chemokines
Anti-inflammatory and pro-inflammatory cytokines were assessed in frozen sera using the U-PLEX Multiplex electro-chemiluminescence assay from MSD (Meso Scale Discovery) according to the provider’s instructions. The following cytokines were tested: GM-CSF, IFN-α2a, IFN-γ, IL-1β, IL-4, IL-6, IL-8, IL-10, IL-12p70, IP-10, MIP-1α, TNF-α and IL-2. Cytokine concentrations were calculated using standards provided by MSD. Samples were read on a MESO QuickPlex SQ 120 reader.
Immune cell phenotyping using flow cytometry
PBMCs were obtained by density gradient centrifugation of heparinized blood samples with Lymphoprep lymphocyte separation medium (Axis-Shield) and analyzed by flow cytometry. The following mAb staining panel was used for surface staining and establish cell subpopulations: CD3-AF488 (SP34–2; BD), CD4-PerCP-Cy5.5 (L200; BD), CD8-APC-H7 (SK1; BD), CD14-Vioblue (M5E2; Miltenyi), CD16-AF700 (3G8; BD), CD20-BV421 (2H7; BioLegend), CD28-ECD (CD28.2; IOtest), CD45-BUV395 (D058–1283; BD), CD95-BV605 (DX2; BioLegend), CD127-PE (ebioRDR5; Ebioscience), CD159-PE-Cy7 (Z199; Beckman Coulter).
T-cells were gated as follows: Granulocytes/Lymphocytes (by size and granularity), Singlets (by Doublet exclusion), Time gate (proper acquisition), CD45+ population, CD3+/CD14- cells, all before gating of CD4 and CD8 subsets. Naive and memory populations were gated based on CD28/CD95 expression.
Absolute number of subpopulations were calculated based on frequencies measured by flow cytometry and absolute number of lymphocytes measured by hematology analyzer (Sysmex Sf-3000; Goffin Meyvis, The Netherlands).
For pSTAT5 and Ki67 analysis, blood cells were incubated with surface antibodies, before permeabilization with PerFix EXPOSE (PFE, Beckman Coulter, Brea, CA), following the manufacturer’s instructions. Intracellular staining was performed with pSTAT5-AF647 (47/STAT5; BD), Ki67-BV711 (B56; BD) antibodies.
Anti-hIL-7-Fc neutralization assay
PB-1 cells, murine pre-B cell line dependent on IL-7 for growth, are classically used in IL-7 proliferation-based bioassays to estimate the concentration of anti-IL-7 neutralizing antibodies in serum and plasma samples.Citation34 Serial dilutions of decomplemented NHP sera were mixed with a recombinant, soluble hIL-7-Fc (exact counterpart of the MVA-expressed hIL-7-Fc, Geneart, Regensburg, Germany).Citation28 Thereafter, PB-1 cells were added and incubated for 3 days at 37°C. As controls, cells were also incubated in complete medium alone to determine the background (blank) or with rhIL-7-Fc without serum to determine the 100% proliferation level (uninhibited control). Cell proliferation was assessed with MTS/PMS CellTiter 96® Aqueous Non-Radioactive (Promega) and optic density was measured at 490 nm with a plate reader (Tecan, Spark). For each serum, the neutralization titer was defined as the dilution allowing to neutralize 50% (ND50) or 90% (ND90) of the signal obtained with uninhibited control.
Results
Intravenous injection of MVA-hIL-7-Fc induces an early transient inflammation
Our previous study performed in mice had established that the IV route resulted in the most favorable pharmacokinetics and immune activities of the MVA-hIL-7-Fc when compared with the more classical intramuscular and subcutaneous routes.Citation28 To investigate the safety of the MVA-hIL-7-Fc when injected once by the IV route at the animal equivalent dose based on the foreseen clinical dose, we first analyzed the evolution of clinical, hematological and biochemical parameters in blood. No major adverse reaction was reported, no flu-like syndrome was detected. Body temperature, weight, heart and breathing rates stayed overall either stable or displayed minor increases which remained in the range of normal values observed in healthy animals (Supp. Figure S1). A transient increase of heart rate was observed only at the first time point and was attributed to sedation procedure.Citation35 Globally, cellular homeostasis was not modified following injection of MVA-hIL-7-Fc (). Compared to baseline levels, a small increase of white and red blood cell counts was observed during the first 24 h p.i. (<1,75 and 1.15 folds in mean, respectively). Numbers return to baseline within 48 hours and remained within normal reference range over the experiment. A limited increase of platelet count was observed (2- to 3-fold) 2 weeks p.i. but remained in normal range except for one animal at 1 time point, in agreement with what has been reported in the literature following any viral infection.
Figure 1. MVA-hIL-7-Fc induces an early transient inflammation.
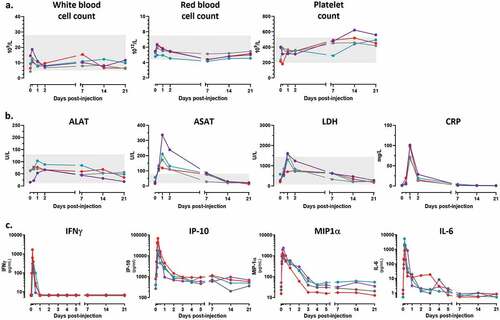
To document the early inflammatory response induced by injection of MVA-hIL-7-Fc, levels of biochemical markers and inflammatory cytokines were measured in blood at different time points p.i. Conventional biomarkers associated with inflammation such as transaminase (ASAT but not ALAT), lactate dehydrogenase (LDH) and C-reactive protein (CRP) showed a sharp transient rise in the first 24 hours p.i in all 4 animals although levels rapidly returned to baseline levels (). Levels of GM-CSF, IFN-α2a, IFN-γ, IL-1β, IL-2, IL-4, IL-6, IL-8, IL-10, IL-12p70, IP-10, MIP-1α and TNF-α were measured using a multiplex assay. Only IFN-γ, IP-10, MIP-1α and IL-6 were detected (). During the first hours p.i., elevated levels of these five inflammatory cytokines were observed but resumed rapidly. At 4 h p.i., a mean of 675 pg/mL of IFN-γ and 2530 pg/mL of IL-6 were measured, and concentrations rapidly decreased thereafter to return to baseline levels within 24–48 hr p.i. Similarly, the peak of IP-10 and MIP-1α was reached 6 h p.i. (35100 and 1337 pg/mL, respectively) and levels rapidly declined during the following 24-72 h. Overall, all measured inflammatory cytokines and biochemical markers were rapidly induced following MVA-hIL-7-Fc injection but detected levels promptly returned to baseline within 1 to 2 days. These data indicate that an intravenous injection of the hIL-7-Fc-armed MVA induces an early transient inflammation as reported for post-MVA administration or following viral infections.Citation36,Citation37
MVA-hIL7-Fc results in production of functional hIL-7-Fc
Pharmacokinetics of circulating hIL-7-Fc was assessed in blood at different time points after injection of MVA-hIL-7-Fc. The hIL-7-Fc was rapidly produced with a peak concentration observed at 12 h p.i. (). The mean peak concentration was calculated at 52,5 ng/mL (ranging from 50,9 to 67,2 ng/mL). The cytokine remained detectable up to 3–4 days p.i. and the mean area under curve (AUC) was calculated at 1256 h*ng/mL.
Figure 2. MVA-hIL-7-Fc induces the production of functional hIL-7-Fc.
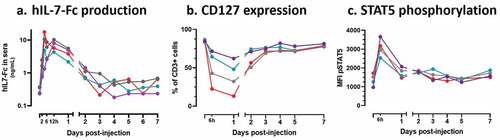
It is known that IL-7 recognition by its receptor (CD127) downregulates the surface expression of CD127.Citation38 We evaluated the expression of CD127 at the surface of blood T cells (). From 6 h and up to 24 h post injection, the percentage of CD127 expressing T cells decreased from 85% on D0 to 39% on D1 (mean decreases). This percentage returned to baseline 3 days post-injection. These variations correspond to the timing of circulating IL-7-Fc detection and strongly suggest that the produced hIL-7-Fc is capable to engage with its receptor.
Binding of hIL7-Fc to its receptor on T cells results in phosphorylation of STAT5 (pSTAT5). This was measured by cytometry over time in blood cells. We observed that the level of STAT5 phosphorylation peaked 6 h p.i. in CD3+ T cells and returned to baseline levels in the following days depending on the animal ().
Overall, these observations indicate that the injection of the MVA-hIL-7-Fc induces a readily detectable circulating hIL7-Fc, capable to recognize its receptor and to induce downstream signaling.
MVA-hIL-7-Fc increases circulating T lymphocyte counts together with other blood immune cells (B lymphocytes, NK cells and monocytes)
We further investigated the role of hIL-7-Fc in stimulating lymphocyte expansion after MVA-hIL-7-Fc injection. After an initial decrease for 2 days representing a priori the migration of T cells out of the blood compartment as previously described,Citation6,Citation18,Citation39 a marked increase of the absolute lymphocyte counts (ALC) was detected for all 4 animals (). An ALC of 2,7 to 4,4.109 cell/L was observed at day 7 p.i., representing 2- to 4-fold increase compared with baseline values. Number of lymphocytes normalized 2 weeks post injection as expected due to homeostatic control, but the ALC remained still superior (mean increase of 49%) at the end of the experiment compared with counts measured before MVA injection.
Figure 3. MVA-hIL-7-Fc increases circulating T lymphocytes together with other blood immune cells (B lymphocytes, NK cells and monocytes).
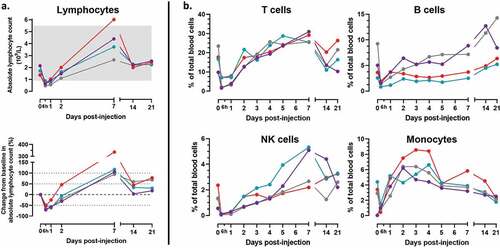
Increase in ALC was mostly due to increase in T-cells (). Concomitant with the ALC increase, the percentage of CD3+ T cells initially decreased before peaking at D7 representing then between 25% and 31% of blood cells (corresponding to increases ranging from +10% to +215% compared with baseline values). An increase of NK cells was also observed following a similar kinetics in all 4 animals (i.e. at first a transient reduction followed by an increase) but most significantly in 2 of them. For these 2 animals, the percent of NK cells sharply increased thereafter (up to 8-fold at D7 p.i.) and decreased continuously up to D21 at which time point values remain still above baselines. The effect of MVA-hIL-7-Fc on B cells was more variable and delayed. After an initial transient reduction B cell percentage slowly normalized within 1 week and increased continuously until the end of follow up (+34% in mean compared with baseline values). For all animals, after a transient decrease observed at 6 h p.i., the percent of monocytes continuously increased up to D4-D5 p.i., before slowly diminishing thereafter up to D21 to mostly return to baseline levels.
Overall, these data indicate that a single injection of MVA-hIL-7-Fc increases numbers and/or percentages of different circulating immune cells albeit with distinct kinetics. For some cell types (B and NK cells) and for some animals, the observed increase lasted up to the end of follow-up (D21 p.i.).
MVA-hIL7-Fc increases absolute counts of CD4 and CD8 T cells, both naïve and central memory populations, and triggered an increase of T cell proliferation
We further analyzed which of the CD4 or CD8 subpopulation of T cells was increased following injection of MVA-hIL-7-Fc. As shown in , both CD4 and CD8 T cells increased overall to a similar level albeit increases tended to be higher for the CD8 population. The peak increase was seen 7 days p.i., at which time the number of CD4 and CD8 lymphocytes reached 1,25.109 and 1,1.109 cells/L, respectively, corresponding to 2,61-fold for CD4 and 2,76-fold for CD8 T cells increases compared with baseline values. The ratio CD4/CD8 did not change at any of the analyzed time-points.
Figure 4. MVA-hIL7-Fc induces T-cell proliferation and increases number of circulating naïve and memory CD4 and CD8 cells.
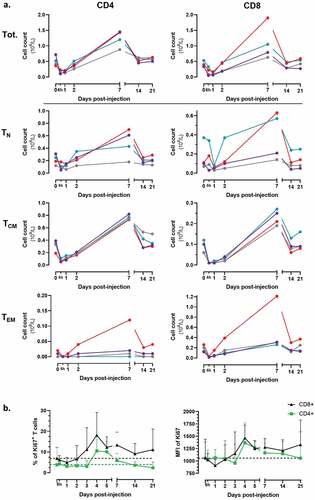
Analysis of the evolution of naïve and memory T cell subpopulations within the CD4 or CD8 T cell compartment revealed that the number of all CD4 and CD8 subpopulations transiently decreased during the first 24 h post injection but the memory compartment was more impacted than the naïve one. Specifically, at this early time point, percentages of decrease compared to baseline values were for the CD4 cell compartment 63% for TCM, 73% for TEM, 43% for TN and, respectively, of 85%, 66%, 59% for the CD8 T cell compartment. Thereafter, cell number started to increase typically from D2 and to D7 at which time the peak increase was seen for all cell populations (with some variation according to cell types and/or animals). Specifically, at 7 days p.i. the mean number of naïve and central memory CD4 T cells reached up-to 4.108/L and 8.108/L, respectively (representing increases of 121% and 145%, respectively, compared with D0 values). Even if effector memory CD4 T cells reached only a mean concentration of 0,1.108/L in mean for 3:4 animals, this level represents an increase of 220% compared with D0 values. The last animal displayed a sharp increase of EM CD4 T cells reaching 1,1.108/L (390% compared with D0 values). Like CD4 T cells, peak increases of all CD8 cell subpopulations were observed at D7, with mean numbers of 3,8.108, 2,2.108 and 4,5.108/L, respectively, for the naïve, central memory and effector populations (representing, respectively, increases of 137%, 167% and 193% compared with D0 values). The 4th animal (same as described above) displayed an important increase of EM CD8 T cells up to 12,1.108/L (362% compared with D0 values).
The increase of T cell numbers was associated with proliferation of the cells. We measured the well-known proliferation marker Ki67 by flow cytometry (). An increase in both the percentage of Ki67 positive T cells and the level of Ki67 expression was observed for both CD4 and CD8 T cells 4 days after injection of MVA-hIL7-Fc. Specifically, increases of 18% for CD8 and 11% for CD4 T cells expressing Ki67 were observed.
Discussion
This exploratory proof-of-concept study demonstrates that a single intravenous injection of the non-replicative recombinant MVA expressing the human IL-7-Fc cytokine used at a relevant clinical dose in a large animal model, is active without adverse reactions. Consistent with the results obtained in murine models,Citation28 a significant expression of hIL-7-Fc was detected in blood which was associated with an increase of both circulating CD4 and CD8 T cells. Beyond T lymphocytes, increase of additional immune cells (B and NK cells, monocytes) was also observed.
To our knowledge our study is the first one reporting early safety data and biological activities of an IL-7 encoded by a viral vector in nonhuman primates. IL-7 has typically been used as adjuvant to enhance and extend vaccine immunogenicity and efficacy. Numerous pre-clinical studies have shown that IL-7 can enhance vaccine protection by improving immunogen-specific cellular and/or humoral immune responses.Citation5 While most of these studies have used recombinant soluble IL-7, only a handful of recombinant vaccines directly encoding the IL-7 cytokine have been described.Citation5 For example, the murine IL-7 gene has been inserted into adenovirus,Citation26 nonlytic Newcastle disease virus,Citation25 attenuated rabies virus,Citation27 or into recombinant M. bovis BCG.Citation40 In these mouse studies, the expected role of the encoded IL-7 to increase vaccine-immunogen specific immunity and efficacy in an adjuvant-like fashion was successfully demonstrated. These studies did not document the pharmacokinetics of the produced IL-7 nor its bioactivity (e.g. impact on immune cell number and activities). We did not identify published studies in nonhuman primates with these IL-7 recombinant vaccines.
We observed here that the MVA-encoded hIL-7-Fc was rapidly produced and detected in blood up to 3–4 days after a single injection. A few studies using soluble IL-7 have been described in NHP but in a setting where the cytokine was injected multiple times hence precluding comparison with our study.Citation16,Citation18,Citation19 In contrast and in some human trials, the pharmacokinetics and specific biological activities have been reported following a single injection of a soluble IL-7. In these studies, IL-7 was detected 24-30 h p.i. in serum.Citation6,Citation9,Citation11,Citation41,Citation42 Recently, a hIL-7 fused to the IgD/IgG4 immunoglobulin domain was injected once intramuscularly in healthy volunteers. Lee et al. report the detection of the hIL-7-HyFc in blood until 7 days p.i. when the highest dose of the cytokine was used.Citation39 However, despite the very high amount injected (60 µg/kg), only a moderate concentration of IL-7 was measured at the peak (1,2 ng/mL 4 h p.i.) while very low levels persisted up to 7 days p.i. (0,2 ng/mL). Interestingly, in their study, authors report the induction of partially neutralizing anti-IL-7-HyFc antibodies.Citation39 In our study, we measured the presence of neutralizing anti-hIL-7-Fc antibodies in plasma of MVA-hIL-7-Fc injected primates but could not detect them up to 21 days p.i. (last time point of the study) (Supp. Figure S2).
The combination of three elements may have contributed to the level and persistence of detection of the hIL-7-Fc produced after injection of the MVA-hIL-7-Fc in primates. First, the role of the Fc domain which can be recycled due to its interaction with the neonatal Fc receptor.Citation32 This recycling mechanism is expected to protect the hIL7-Fc from lysosomal degradation and thereby prolong its persistence. Second, the large molecular size of hIL-7-Fc (43 kDa for a monomer, 100kDa molecular weight for the glycosylated dimer)Citation28 makes it less susceptible to systemic clearance through the kidneys.Citation43 Third and importantly, the use of a vector as delivery platform was anticipated to play a key and original role in the pharmacokinetics of the expressed cytokine. Indeed, following an IV injection of the MVA virus, different cell types of multiple organs will be infected and express the engineered payload.Citation44,Citation45 Hence, the multiple cells targeted by the MVA are expected to result in a wide and prolonged cytokine expression directly in vivo.
It is well known that IL-7 signaling downregulates CD127 expression on mature T cells, limiting uncontrolled T-cell proliferation.Citation4 We have observed in the present study a rapid declining expression of CD127 on T cells before returning to baseline 72 h after injection of MVA-hIL-7-Fc. This observation confirms the capacity of the in vivo produced hIL-7-Fc to be recognized by its receptor. The delay necessary for CD127 expression to return to baseline level (3 days p.i.) suggests, at least in the NHP model, that to be effective and stimulate T cells again a second injection of the MVA-hIL-7-Fc should be contemplated at Day 3 or later post-primary injection. One may question the relevance of daily injections of soluble IL-7 reported in some studies, in which lymphocyte expression of CD127 remains low during the entire course of treatment (lasting in some instances several weeks).Citation3,Citation7,Citation17
Preclinical studies have demonstrated the influence of multiple injections of recombinant soluble IL-7 on lymphocyte count in healthy NHP.Citation16,Citation18,Citation19 In our study, a single injection of MVA-hIL-7-Fc markedly modified the absolute lymphocyte count. The initial decrease in count during the first 48 h, attributed to early cell trafficking out of the circulation into tissues and peripheral lymphoid organs, was expected and previously reported in primate and human.Citation6,Citation18,Citation39 Consistent with observations in murine models obtained with MVA-hIL-7-Fc, we confirmed here in a larger animal species an important increase of ALC. Although not formally comparable, it is interesting to note that this increase reaches same range or higher than that reported after multiple injections of recombinant IL-7s.Citation6,Citation18,Citation39,Citation46 Importantly, the effect of MVA-hIL-7-Fc persists in time. The ALC increase was mostly due to increase of T cells, both CD4 and CD8 lymphocytes and mostly naïve and TCM cells. Increase was associated with an increase of Ki67-positive T cell proportion as previously reported.Citation3,Citation19,Citation46,Citation47 The number of B lymphocytes also increased albeit at later times p.i. Earlier studies in NHP have described a limited increase of B cell number following multiple injections of soluble of IL-7.Citation18,Citation21 In human trials, recombinant IL-7 treatment increased the B lymphocyte blood count in some patients.Citation6,Citation39 IL-7 is known to be a key regulator of the B lymphopoiesis, promoting the proliferation, survival and maturation during the pro-B to pre-B cell transition.Citation1,Citation48 IL-7 also promotes B survival by modulating pro- and anti-apoptotic factor production and by inducing B regulatory factor expression at the surface of peripheral T cells.Citation1,Citation49 It would be interesting to further study the effect of the hIL-7-Fc encoded by the MVA on B-cell subpopulations number and functions.
Recombinant MVAs in the clinic have typically been administered by intramuscular, subcutaneous, or intradermic routes. The intravenous route has recently been documented by DeMaria et al.Citation50 who report on good tolerance of the MVA-BN-brachyury-TRICOM vaccine injected by this route in advance solid tumor indications. Another preventive MVA-based vaccine against malaria using the IV route in healthy volunteers is currently under evaluation (NCT03707353, NCT03084289, NCT04009096). While a handful of preclinical studies on recombinant MVAs injected IV in mouse models have been reported,Citation44,Citation45 evaluations in nonhuman primates have not been described. Our study documents for the first time the evolution of clinical, hematological, and biochemical parameters after intravenous injection of an armed MVA in cynomolgus primates. Only a moderate and transient inflammation was observed during the first 48 h post injection. We detected an increase of the circulating pro-inflammatory cytokines (IFNγ, IP-10, MIP-1α, IL-6, IL-8) and inflammation associated biochemical markers (ASAT, LDH, CRP) within the first 6-24 h post MVA injection (). The MVA vector is known to be a strong inducer of innate immunity, via interferon signaling and inflammasome pathways. Studies have described that these proinflammatory cytokines are produced in vitro and in vivo in response to MVA in whole blood, PBMCs, primary human monocytes, and macrophages.Citation29,Citation30,Citation36,Citation51 Similar expression of these pro-inflammatory cytokines has also been observed in mice after MVA-hIL-7-Fc injection.Citation28 In our study, we clearly observed, in addition to T and B lymphocytes, an increase of cells from the innate lineage particularly NK cells and monocytes likely due to the capacity of the MVA vector itself to stimulate the innate system.Citation30 The transient increase of monocytes observed during the first 3 days p.i. reflects the classical response to inflammation caused by the intravenous injection of the viral vector.Citation52 MVA is also known to activate and induce proliferation of NK cells in mice and NHP.Citation53,Citation54 In addition, IL-7 may also contribute to the maturation and the survival of NK cells.Citation1,Citation55 Additional studies including an experimental group treated by the empty MVA are warranted to precisely elucidate the role of the MVA backbone on the effects observed on these cell populations.
Overall, the MVA-hIL-7-Fc represents an innovative and safe immunotherapy capable to stimulate both the innate and adaptive immune systems. The present study underlines particularly the capacity of the MVA-expressed hIL-7-Fc to increase numbers of circulating naïve and memory CD4 and CD8 T-lymphocytes. It confirms in a large animal model earlier studies conducted in mice,Citation28 and in ex vivo surrogate human models using PBMC from bacterial sepsis and COVID-19 patients in ICU as well as from immunosenescent hip fractured patients.Citation56,Citation57 Taken together, these studies support the clinical development of MVA-hIL-7-Fc in immunosuppressed situations.
Author contributions
C-A.C, P.M. and G.I. designed the study and interpretation of data. C-A.C. and M.B. set up the protocols. M.V. was responsible for the in vivo execution of the experiment. S.H. performed the ex vivo flowcytometry analysis C.D., A.E. and N.K. measured the concentrations of hIL-7-Fc, cytokines and neutralizing anti-IL-7 antibodies in blood. C-A.C. performed analysis. The first draft was written by C-A.C. and G.I. contributed to revised versions of the manuscript. All authors read, reviewed, and approved the final manuscript.
Supplemental Material
Download Zip (250.5 KB)Acknowledgments
Authors would like to thank Jean-Baptiste Marchand, Nathalie Silvestre and Clementine Spring-Giusti for their continuous support.
Disclosure statement
No potential conflict of interest was reported by the authors.
Supplementary material
Supplemental data for this article can be accessed on the publisher’s website at https://doi.org/10.1080/21645515.2022.2133914
Additional information
Funding
References
- Chen D, Tang T-X, Deng H, Yang X-P, Tang Z-H. Interleukin-7 biology and its effects on immune cells: mediator of generation, differentiation, survival, and homeostasis. Front Immunol. 2021;12:1. doi:10.3389/fimmu.2021.747324.
- Barata JT, Durum SK, Seddon B. Flip the coin: iL-7 and IL-7R in health and disease. Nat Immunol. 2019;20(12):1584–10. doi:10.1038/s41590-019-0479-x.
- Fry TJ, Moniuszko M, Creekmore S, Donohue SJ, Douek DC, Giardina S, Hecht TT, Hill BJ, Komschlies K, Tomaszewski J, et al. IL-7 therapy dramatically alters peripheral T-cell homeostasis in normal and SIV-infected nonhuman primates. Blood. 2003;101(6):2294–99. doi:10.1182/blood-2002-07-2297.
- Park J-H, Yu Q, Erman B, Appelbaum JS, Montoya-Durango D, Grimes HL, Singer A. Suppression of IL7Ralpha transcription by IL-7 and other prosurvival cytokines: a novel mechanism for maximizing IL-7-dependent T cell survival. Immunity. 2004;21(2):289–302. doi:10.1016/j.immuni.2004.07.016.
- Huang J, Long Z, Jia R, Wang M, Zhu D, Liu M, Chen S, Zhao X, Yang Q, Wu Y, et al. The broad immunomodulatory effects of IL-7 and its application in vaccines. Front Immunol. 2021;12:680442. doi:10.3389/fimmu.2021.680442.
- Sportès C, Babb RR, Krumlauf MC, Hakim FT, Steinberg SM, Chow CK, Brown MR, Fleisher TA, Noel P, Maric I, et al. Phase I study of recombinant human interleukin-7 administration in subjects with refractory malignancy. Clin Cancer Res. 2010;16(2):727–35. doi:10.1158/1078-0432.CCR-09-1303.
- Sportès C, Hakim FT, Memon SA, Zhang H, Chua KS, Brown MR, Fleisher TA, Krumlauf MC, Babb RR, Chow CK, et al. Administration of rhIL-7 in humans increases in vivo TCR repertoire diversity by preferential expansion of naive T cell subsets. J Exp Med. 2008;205(7):1701–14. doi:10.1084/jem.20071681.
- Trédan O, Ménétrier-Caux C, Ray-Coquard I, Garin G, Cropet C, Verronèse E, Bachelot T, Rebattu P, Heudel PE, Cassier P, et al. ELYPSE-7: a randomized placebo-controlled phase IIa trial with CYT107 exploring the restoration of CD4+ lymphocyte count in lymphopenic metastatic breast cancer patients. Ann Oncol. 2015;26(7):1353–62. doi:10.1093/annonc/mdv173.
- Sereti I, Dunham RM, Spritzler J, Aga E, Proschan MA, Medvik K, Battaglia CA, Landay AL, Pahwa S, Fischl MA, et al. IL-7 administration drives T cell–cycle entry and expansion in HIV-1 infection. Blood. 2009;113(25):6304–14. doi:10.1182/blood-2008-10-186601.
- Lévy Y, Lacabaratz C, Weiss L, Viard J-P, Goujard C, Lelièvre J-D, Boué F, Molina J-M, Rouzioux C, Avettand-Fénoêl V, et al. Enhanced T cell recovery in HIV-1–infected adults through IL-7 treatment. J Clin Invest. 2009;119(4):997–1007. doi:10.1172/JCI38052.
- Francois B, Jeannet R, Daix T, Walton AH, Shotwell MS, Unsinger J, Monneret G, Rimmelé T, Blood T, Morre M, et al. Interleukin-7 restores lymphocytes in septic shock: the IRIS-7 randomized clinical trial. JCI Insight. 2018;3(5):e98960. doi:10.1172/jci.insight.98960.
- Turnbull IR, Mazer MB, Hoofnagle MH, Kirby JP, Leonard JM, Mejia-Chew C, Spec A, Blood J, Miles SM, Ransom EM, et al. IL-7 immunotherapy in a nonimmunocompromised patient with intractable fungal wound sepsis. Open Forum Infect Dis. 2021;8(6):ofab256. doi:10.1093/ofid/ofab256.
- Monneret G, de Marignan D, Coudereau R, Bernet C, Ader F, Frobert E, Gossez M, Viel S, Venet F, Wallet F. Immune monitoring of interleukin-7 compassionate use in a critically ill COVID-19 patient. Cell Mol Immunol. 2020;17(9):1001–03. doi:10.1038/s41423-020-0516-6.
- Mazer MB, Turnbull IR, Miles S, Blood TM, Sadler B, Hess A, Botney MD, Martin RS, Bosanquet JP, Striker DA, et al. Interleukin-7 reverses lymphopenia and improves T-cell function in coronavirus disease 2019 patient with inborn error of toll-like receptor 3: a case report. Crit Care Explor. 2021;3(7):e0500. doi:10.1097/CCE.0000000000000500.
- Laterre PF, François B, Collienne C, Hantson P, Jeannet R, Remy KE, Hotchkiss RS. Association of interleukin 7 immunotherapy with lymphocyte counts among patients with severe coronavirus disease 2019 (COVID-19). JAMA Network Open. 2020;3(7):e2016485. doi:10.1001/jamanetworkopen.2020.16485.
- Nugeyre M-T, Monceaux V, Beq S, Cumont M-C, Ho Tsong Fang R, Chêne L, Morre M, Barré-Sinoussi F, Hurtrel B, Israël N. IL-7 stimulates T cell renewal without increasing viral replication in simian immunodeficiency virus-infected macaques. J Immunol. 2003;171(8):4447–53. doi:10.4049/jimmunol.171.8.4447.
- Beq S, Nugeyre M-T, Ho Tsong Fang R, Gautier D, Legrand R, Schmitt N, Estaquier J, Barré-Sinoussi F, Hurtrel B, Cheynier R, et al. IL-7 induces immunological improvement in SIV-infected rhesus macaques under antiviral therapy. J Immunol. 2006;176(2):914–22. doi:10.4049/jimmunol.176.2.914.
- Beq S, Rozlan S, Gautier D, Parker R, Mersseman V, Schilte C, Assouline B, Rancé I, Lavedan P, Morre M, et al. Injection of glycosylated recombinant simian IL-7 provokes rapid and massive T-cell homing in rhesus macaques. Blood. 2009;114(4):816–25. doi:10.1182/blood-2008-11-191288.
- Leone A, Rohankhedkar M, Okoye A, Legasse A, Axthelm MK, Villinger F, Piatak M, Lifson JD, Assouline B, Morre M, et al. Increased CD4+ T cell levels during IL-7 administration of antiretroviral therapy-treated simian immunodeficiency virus-positive macaques are not dependent on strong proliferative responses. J Immunol. 2010;185(3):1650–59. doi:10.4049/jimmunol.0902626.
- Parker R, Dutrieux J, Beq S, Lemercier B, Rozlan S, Fabre-Mersseman V, Rancez M, Gommet C, Assouline B, Rancé I, et al. Interleukin-7 treatment counteracts IFN-α therapy-induced lymphopenia and stimulates SIV-specific cytotoxic T lymphocyte responses in SIV-infected rhesus macaques. Blood. 2010;116(25):5589–99. doi:10.1182/blood-2010-03-276261.
- Storek J, Gillespy T, Lu H, Joseph A, Dawson MA, Gough M, Morris J, Hackman RC, Horn PA, Sale GE, et al. Interleukin-7 improves CD4 T-cell reconstitution after autologous CD34 cell transplantation in monkeys. Blood. 2003;101(10):4209–18. doi:10.1182/blood-2002-08-2671.
- Vassena L, Miao H, Cimbro R, Malnati MS, Cassina G, Proschan MA, Hirsch VM, Lafont BA, Morre M, Fauci AS, et al. Treatment with IL-7 prevents the decline of circulating CD4+ T cells during the acute phase of SIV infection in rhesus macaques. PLoS Pathog. 2012;8(4):e1002636. doi:10.1371/journal.ppat.1002636.
- Okoye AA, Rohankhedkar M, Konfe AL, Abana CO, Reyes MD, Clock JA, Duell DM, Sylwester AW, Sammader P, Legasse AW, et al. Effect of IL-7 therapy on naive and memory T cell homeostasis in aged rhesus macaques. J Immunol. 2015;195(9):4292–305. doi:10.4049/jimmunol.1500609.
- Ura T, Okuda K, Shimada M. Developments in viral vector-based vaccines. Vaccines (Basel). 2014;2(3):624–41. doi:10.3390/vaccines2030624.
- Zhao L, Mei Y, Sun Q, Guo L, Wu Y, Yu X, Hu B, Liu X, Liu H. Autologous tumor vaccine modified with recombinant new castle disease virus expressing IL-7 promotes antitumor immune response. J Immunol. 2014;193(2):735–45. doi:10.4049/jimmunol.1400004.
- Ohs I, Windmann S, Wildner O, Dittmer U, Bayer W. Interleukin-encoding adenoviral vectors as genetic adjuvant for vaccination against retroviral infection. PLoS ONE. 2013;8(12):e82528. doi:10.1371/journal.pone.0082528.
- Li Y, Zhou M, Luo Z, Zhang Y, Cui M, Chen H, Fu ZF, Zhao L, Williams BRG. Overexpression of interleukin-7 extends the humoral immune response induced by rabies vaccination. J Virol. 2017;91(7). doi:10.1128/JVI.02324-16.
- Lélu K, Dubois C, Evlachev A, Crausaz M, Baldazza M, Kehrer N, Brandely R, Schlesinger Y, Silvestre N, Marchand J-B, et al. Viral delivery of IL-7 is a potent immunotherapy stimulating innate and adaptive immunity and confers survival in sepsis models. J Immunol. 2022;209(1):99–117. doi:10.4049/jimmunol.2101145.
- Delaloye J, Roger T, Steiner-Tardivel Q-G, Le Roy D, Knaup Reymond M, Akira S, Petrilli V, Gomez CE, Perdiguero B, Tschopp J, et al. Innate immune sensing of modified vaccinia virus Ankara (MVA) is mediated by TLR2-TLR6, MDA-5 and the NALP3 inflammasome. PLoS Pathog. 2009;5(6):e1000480. doi:10.1371/journal.ppat.1000480.
- Price PJR, Torres-Domínguez LE, Brandmüller C, Sutter G, Lehmann MH. Modified Vaccinia virus Ankara: innate immune activation and induction of cellular signalling. Vaccine. 2013;31(39):4231–34. doi:10.1016/j.vaccine.2013.03.017.
- Verheust C, Goossens M, Pauwels K, Breyer D. Biosafety aspects of modified vaccinia virus Ankara (MVA)-based vectors used for gene therapy or vaccination. Vaccine. 2012;30(16):2623–32. doi:10.1016/j.vaccine.2012.02.016.
- Sockolosky JT, Szoka FC. The neonatal Fc receptor, FcRn, as a target for drug delivery and therapy. Adv Drug Deliv Rev. 2015;91:109–24. doi:10.1016/j.addr.2015.02.005.
- US Food and Drug Administration - Center for Drug Evaluation and Research. 2005. Guidance for Industry - Estimating the Maximum Safe Starting Dose in Initial Clinical Trials for Therapeutics in Adult Healthy Volunteers. https://www.fda.gov/regulatory-information/search-fda-guidance-documents/estimating-maximum-safe-starting-dose-initial-clinical-trials-therapeutics-adult-healthy-volunteers.
- Mire-Sluis AR, Healey L, Griffiths S, Hockley D, Thorpe R. Development of a continuous IL-7-dependent murine pre-B cell line PB-1 suitable for the biological characterisation and assay of human IL-7. J Immunol Methods. 2000;236(1–2):71–76. doi:10.1016/s0022-1759(99)00237-9.
- Richig JW, Sleeper MM. Electrocardiography of nonhuman primates. pp. 57–71. doi:10.1016/B978-0-12-809469-3.00008-6.
- Sanchez J, Gonçalves E, Llano A, Gonzáles P, Fernández-Maldonado M, Vogt A, Soria A, Perez S, Cedeño S, Fernández MA, et al. Immune profiles identification by vaccinomics after MVA immunization in randomized clinical study. Front Immunol. 2020;11:586124. doi:10.3389/fimmu.2020.586124.
- Velazquez-Salinas L, Verdugo-Rodriguez A, Rodriguez LL, Borca MV. The role of interleukin 6 during viral infections. Front Microbiol. 2019;10:1057. doi:10.3389/fmicb.2019.01057.
- Ghazawi FM, Faller EM, Sugden SM, Kakal JA, MacPherson PA. IL-7 downregulates IL-7Rα expression in human CD8 T cells by two independent mechanisms. Immunol Cell Biol. 2013;91(2):149–58. doi:10.1038/icb.2012.69.
- Lee SW, Choi D, Heo M, Shin E-C, Park S-H, Kim SJ, Oh Y-K, Lee BH, Yang SH, Sung YC, et al. hIL-7-hyFc, a long-acting IL-7, increased absolute lymphocyte count in healthy subjects. Clin Transl Sci. 2020;13(6):1161–69. doi:10.1111/cts.12800.
- Hatano S, Tamura T, Umemura M, Matsuzaki G, Ohara N, Yoshikai Y. Recombinant mycobacterium bovis bacillus Calmette-Guerin expressing Ag85B-IL-7 fusion protein enhances IL-17A-producing innate γδ T cells. Vaccine. 2016;34(22):2490–95. doi:10.1016/j.vaccine.2016.03.096.
- Lévy Y, Sereti I, Tambussi G, Routy JP, Lelièvre JD, Delfraissy JF, Molina JM, Fischl M, Goujard C, Rodriguez B, et al. Effects of recombinant human interleukin 7 on T-cell recovery and thymic output in HIV-infected patients receiving antiretroviral therapy: results of a phase I/IIa randomized, placebo-controlled, multicenter study. Clin Infect Dis. 2012;55(2):291–300. doi:10.1093/cid/cis383.
- Perales M-A, Goldberg JD, Yuan J, Koehne G, Lechner L, Papadopoulos EB, Young JW, Jakubowski AA, Zaidi B, Gallardo H, et al. Recombinant human interleukin-7 (CYT107) promotes T-cell recovery after allogeneic stem cell transplantation. Blood. 2012;120(24):4882–91. doi:10.1182/blood-2012-06-437236.
- Knauf MJ, Bell DP, Hirtzer P, Luo ZP, Young JD, Katre NV. Relationship of effective molecular size to systemic clearance in rats of recombinant interleukin-2 chemically modified with water-soluble polymers. J Biol Chem. 1988;263(29):15064–70. doi:10.1016/S0021-9258(18)68146-3.
- Fend L, Gatard-Scheikl T, Kintz J, Gantzer M, Schaedler E, Rittner K, Cochin S, Fournel S, Préville X. Intravenous injection of MVA virus targets CD8+ lymphocytes to tumors to control tumor growth upon combinatorial treatment with a TLR9 agonist. Cancer Immunol Res. 2014;2(12):1163–74. doi:10.1158/2326-6066.CIR-14-0050.
- Ramirez JC, Finke D, Esteban M, Kraehenbuhl JP, Acha-Orbea H. Tissue distribution of the Ankara strain of vaccinia virus (MVA) after mucosal or systemic administration. Arch Virol. 2003;148(5):827–39. doi:10.1007/s00705-003-0006-z.
- Moniuszko M, Fry T, Tsai W-P, Morre M, Assouline B, Cortez P, Lewis MG, Cairns S, Mackall C, Franchini G. Recombinant interleukin-7 induces proliferation of naive macaque CD4+ and CD8+ T cells in vivo. J Virol. 2004;78(18):9740–49. doi:10.1128/JVI.78.18.9740-9749.2004.
- Steele AK, Carrasco-Medina L, Sodora DL, Crawley AM. Increased soluble IL-7 receptor concentrations associate with improved IL-7 therapy outcomes in SIV-infected ART-treated rhesus macaques. PLoS ONE. 2017;12(12):e0188427. doi:10.1371/journal.pone.0188427.
- Corfe SA, Paige CJ. The many roles of IL-7 in B cell development; mediator of survival, proliferation and differentiation. Semin Immunol. 2012;24(3):198–208. doi:10.1016/j.smim.2012.02.001.
- Sammicheli S, Ruffin N, Lantto R, Vivar N, Chiodi F, Rethi B. IL-7 modulates B cells survival and activation by inducing BAFF and CD70 expression in T cells. J Autoimmun. 2012;38(4):304–14. doi:10.1016/j.jaut.2012.01.012.
- DeMaria PJ, Lee-Wisdom K, Donahue RN, Madan RA, Karzai F, Schwab A, Palena C, Jochems C, Floudas C, Strauss J, et al. Phase 1 open-label trial of intravenous administration of MVA-BN-brachyury-TRICOM vaccine in patients with advanced cancer. J Immunother Cancer. 2021;9(9):e003238. doi:10.1136/jitc-2021-003238.
- Lehmann MH, Kastenmuller W, Kandemir JD, Brandt F, Suezer Y, Sutter G. Modified vaccinia virus ankara triggers chemotaxis of monocytes and early respiratory immigration of leukocytes by induction of CCL2 expression. J Virol. 2009;83(6):2540–52. doi:10.1128/JVI.01884-08.
- Mangaonkar AA, Tande AJ, Bekele DI. Differential diagnosis and workup of monocytosis: a systematic approach to a common hematologic finding. Curr Hematol Malig Rep. 2021;16(3):267–75. doi:10.1007/s11899-021-00618-4.
- Milo I, Blecher-Gonen R, Barnett-Itzhaki Z, Bar-Ziv R, Tal O, Gurevich I, Feferman T, Drexler I, Amit I, Bousso P, et al. The bone marrow is patrolled by NK cells that are primed and expand in response to systemic viral activation. Eur J Immunol. 2018;48(7):1137–52. doi:10.1002/eji.201747378.
- Palgen J-L, Tchitchek N, Huot N, Elhmouzi-Younes J, Lefebvre C, Rosenbaum P, Dereuddre-Bosquet N, Martinon F, Hocini H, Cosma A, et al. NK cell immune responses differ after prime and boost vaccination. J Leukoc Biol. 2019;105(5):1055–73. doi:10.1002/JLB.4A1018-391RR.
- Michaud A, Dardari R, Charrier E, Cordeiro P, Herblot S, Duval M. IL-7 enhances survival of human CD56bright NK cells. J Immunother (1991). 2010;33(4):382–90. doi:10.1097/CJI.0b013e3181cd872d.
- Marton C, Minaud A, Coupet C-A, Vallet H, Boddaert J, Kehrer N, Inchauspe G, Barraud L, Sauce D. A novel virotherapy encoding human interleukin-7 enhances ex vivo human immune functions in immunosenescent hip fracture trauma patients. Unpublished results.
- Crausaz M, Monneret G, Conti F, Lukaszewicz A-C, Marchand J-B, Martin P, Inchauspé G, Venet F. A novel virotherapy encoding human interleukin-7 improves ex vivo T lymphocyte functions in immunosuppressed patients with septic shock and critically ill COVID-19. Front Immunol. 2022;13. doi:10.3389/fimmu.2022.939899.