ABSTRACT
This study assessed the immunogenicity and safety of the BNT162b2 mRNA vaccine in lung cancer patients receiving anticancer treatment. We enrolled lung cancer patients receiving anticancer treatment and non-cancer patients; all participants were fully vaccinated with the BNT162b2 vaccine. Blood samples were collected before the first and second vaccinations and 4 ± 1 weeks after the second vaccination. Anti-severe respiratory syndrome coronavirus-2 (SARS-CoV-2) spike protein S1 subunit receptor-binding domain antibody titers were measured using the Architect SARS-CoV-2 IgG II Quant and Elecsys Anti-SARS-CoV-2 S assays. Fifty-five lung cancer patients and 38 non-cancer patients were included in the immunogenicity analysis. Lung cancer patients showed significant increase in the geometric mean antibody concentration, which was significantly lower than that in the non-cancer patients after the first (30 vs. 121 AU/mL, p < .001 on Architect; 4.0 vs 1.2 U/mL, p < .001 on Elecsys) and second vaccinations (1632 vs. 3472 AU/mL, p = .005 on Architect; 213 vs 573 A/mL, p = .002 on Elecsys). The adjusted odds ratio (aOR) for seroprotection was significantly lower (p < .05) in lung cancer patients than that in non-cancer patients. Analysis of the anticancer treatment types showed that the aOR for seroprotection was significantly lower (p < .05) in lung cancer patients receiving cytotoxic agents. They showed no increase in adverse reactions. BNT162b2 vaccination in lung cancer patients undergoing anticancer treatment significantly increased (p < .05) antibody titers and showed acceptable safety. Immunogenicity in these patients could be inadequate compared with that in non-cancer patients.
Introduction
Coronavirus disease (COVID-19), which originated in China in December 2019, spread globally with an unprecedented impact,Citation1 affecting the management of cancer patients in various ways.Citation2 Considering the poor outcome of COVID-19, cancer patients are particularly at risk from the disease,Citation2 since most cancer patients are elderly and tend to have other underlying diseases along with immunosuppression owing to anticancer treatment.Citation2 Thus, cancer patients are vulnerable to infection and at an increased risk of developing serious complications from COVID-19, which in turn could lead to hospitalization, admission to intensive care units, and/or death.Citation3–7 Additionally, further anticancer treatment is often postponed due to the need to prioritize treatment for COVID-19.2 Therefore, prevention of COVID-19 in cancer patients is crucial.
Vaccines are the primary means to prevent COVID-19; moreover, several formulations have been launched since November 2020.Citation8–10 Cancer patients are being prioritized for COVID-19 vaccinations globally, considering their poor clinical outcomes when infected.Citation11 A prospective study evaluated the immunogenicity of COVID-19 vaccine in patients with solid tumor cancer using the SARS-CoV-2-IgG II Quant assay (Abbott laboratories). This study reported the results of anti-severe respiratory syndrome coronavirus-2 (SARS-CoV-2) receptor-binding domain spike protein IgG (anti-RBD) titers of 102 patients with solid tumor cancers (29 patients with gastrointestinal cancer, 26 with lung cancer, 18 with breast cancer, 9 with brain cancer, 8 with genitourinary cancer, and the remaining 12 with other types of cancer) along with 78 healthy subjects.Citation12 After two doses of mRNA-BNT162b2 vaccine, the median IgG titer of patients with solid tumor cancer was significantly lower than that of healthy controls (1931 AU/mL vs. 7160 AU/mL; p < .001).Citation12 Nevertheless, 90% of patients with solid tumor cancers had a positive antibody response when IgG titer of 50 AU/mL or higher was defined as positive, which was considered adequate.Citation12 Moreover, the vaccination was well tolerated in a large prospective cohort study of 816 patients with solid tumor cancer (120 with melanoma, 54 with sarcoma, and 250 with breast cancer, 168 with lung cancer, 70 with gastrointestinal cancer, 46 with gynecologic cancer, 89 with genitourinary cancer, 9 with head-neck cancer, 3 with cerebral cancer, and 7 with neuroendocrine tumor).Citation13 In this study, approximately 3.5% and 1.3% of the cancer patients suffered severe adverse events after the first and second vaccinations, whereas total adverse events occurred in 44% and 38.3% of patients after the first and second dose, respectively.Citation13 However, studies on the immunogenicity and safety of the COVID-19 vaccine specific to lung cancer patients undergoing chemotherapy are limited; a prospective study of 306 patients with thoracic malignancies (including 282 patients with lung cancer, 13 patients with pleural mesothelioma, and 11 with other thoracic malignancies), using the SARS-CoV-2-IgG II Quant assay (Abbott laboratories) reported that 93.7% patients with thoracic cancer had a positive antibody response after two doses when indexed at ≥ 50 AU/mL, although serum anti-RBD IgG titers were lower in cancer patients than in healthy controls.Citation14 Additionally, variations in immunogenicity based on the type of anticancer drug administered to patients remain unclear. Finally, the correlation between the anti-RBD titers measured using two different immunoassays in lung cancer patients are unknown.
Therefore, we undertook a prospective, multicenter cohort study to evaluate the immunogenicity and safety of the BNT162b2 vaccine in lung cancer patients undergoing anticancer treatment. Non-cancer patients with chronic pulmonary diseases represented the control group. Two different immunoassays were used to measure both the cancer patients’ and controls’ anti-RBD titers.
Materials and methods
Study participants
Lung cancer patients receiving anticancer treatment and non-cancer patients with chronic diseases at the pulmonology departments of two tertiary hospitals in Japan (Kameda Medical Center, Chiba and Chikamori Hospital, Kochi) were invited to participate in the study from May 2021 to September 2021. Patients were included upon fulfilling the following criteria at the time of enrollment: 1) lung cancer patients currently undergoing anticancer treatment aged ≥50 years (lung cancer group), or non-cancer patients with chronic diseases aged ≥50 years (non-cancer group); 2) patients who voluntarily received the COVID-19 vaccine; 3) patients who provided informed written consent to participate in the study. Patients meeting any of the following criteria at the time of enrollment were excluded: 1) patients with contraindications to vaccination; 2) those with a history of COVID-19 infection or previous COVID-19 vaccination (including participation in clinical trials or clinical studies); 3) those receiving systemic steroids or immunosuppressive drugs (except for administration as an antiemetic for anticancer drugs); 4) those with autoimmune diseases under active treatment; 5) those who experienced an acute illness requiring antimicrobial agents or steroids within a month of enrollment; 6) those with fever or acute severe diseases at the time of vaccination; 7) those judged by the principal investigator/sub-investigator to be unsuitable as research subjects. The study protocol was approved by the Hakata Clinic Institutional Review Board (no O-50) and was performed in accordance with the Declaration of Helsinki. This study was registered with the Japan Registry of Clinical Trials, trial number: jRCT1071210024.
During registration, the study participants were asked to fill out a self-administered questionnaire with the following information: date of birth, sex, age, and allergy history. Additionally, the attending physicians collected the following information from the participants on the standardized questionnaire: sex, age, underlying diseases, and Eastern Cooperative Oncology Group performance status. The attending physician gathered the following information for lung cancer patients: histological type of the lung cancer, cancer stage, and details of anticancer treatment.
COVID-19 vaccination
All study participants were administered the BNT162b2 mRNA COVID-19 vaccine (COMIRNATY® intramuscular injection, Pfizer, New York, NY, USA). In Japan, mass vaccination was undertaken for BNT162b2 vaccines, which were stored and prepared according to the package insert. Each person received two vaccination doses, 3–4 weeks apart.
Measurement of antibody titers
Blood samples for pre-vaccination antibody titer measurements were collected from study participants within, or at most, 14 days before the first COVID-19 vaccination (S0); those for post-vaccination antibody titer measurements were collected within, or at most, 7 days before the second vaccination (S1) and 4 ± 1 weeks (21–35 days) after the second vaccination (S2). The collected serum samples were stored at − 20°C in the laboratory of the respective hospitals.
Titers of the SARS-CoV-2 anti-RBD and nucleocapsid protein (anti-N) antibodies were measured from the collected blood samples using the following assays: Architect SARS-CoV-2 IgG II Quant and SARS-CoV-2 IgG (Abbott Laboratories, Abbott Park, Il, USA) and Elecsys Anti-SARS-CoV-2 S and Anti-SARS-CoV-2 (Roche Diagnostics, Basel, Switzerland).Citation15,Citation16 Architect SARS-CoV-2 IgG II Quant is an automated, two-step immunoassay for the qualitative and semi-quantitative detection of anti-RBD antibodies against SARS-CoV-2 in human serum and plasma using chemiluminescent microparticle immunoassay technology.Citation15 The Elecsys Anti-SARS-CoV-2 S uses a recombinant protein representing the RBD of the spike antigen in a double-antigen sandwich assay format.Citation16 For the Architect assays, the cutoff values for a positive anti-RBD (quantitative range: 0–40,000 AU/mL) and anti-N test result were ≥50 AU/mL and ≥1.4 index, respectively.Citation12,Citation15 For the Elecsys assays, the cutoff values for a positive anti-RBD (quantitative range: 0.4–2,500 U/mL) and anti-N test result were ≥0.8 U/mL and ≥1.0 COI, respectively.Citation16 Seropositivity was defined as anti-RBD titers equal to or above the positivity cutoff per assay.
Safety
Adverse events associated with COVID-19 vaccination were monitored. Within 48 h after the first and second vaccinations, the focus was on ocular and respiratory symptoms referring to the safety evaluation of influenza vaccines.Citation17,Citation18 Within, or at most, 7 days after the first and second vaccinations, the focus was on local reactions at the injection site as well as other systemic reactions. The symptoms were documented by the participants completing case cards.
Statistical analysis
Since this study aims to report descriptive data on immunogenicity and safety, we did not perform statistical hypothesis testing in the sample size calculation. Generally, 50 subjects are required in a group according to the international standard for evaluating the immunogenicity of influenza vaccines.Citation19 Therefore, we set an approximate sample size of 100 patients (50 lung cancer and 50 non-cancer patients).
Study participants positive for anti-N antibodies (i.e., those who acquired COVID-19) in blood samples before (S0) and after the first (S1) and second (S2) vaccination were excluded from all analyses. The immunogenicity of the COVID-19 vaccine was measured using the geometric mean antibody concentration (GMC) and GMC ratio of the anti-RBD antibody titer. We set the following cutoff points for seroprotection according to the previous studies: 1) ≥165 BAU/mL, based on the 70% vaccine efficacy threshold against symptomatic COVID19 infection estimated from a prior clinical trial of ChAdOx1 nCoV-19 adenoviral vector vaccine,Citation20 and 2) ≥775 BAU/mL, based on the 90% vaccine efficacy threshold against symptomatic COVID-19 reported in a prior clinical trial of mRNA vaccine.Citation21 Although ≥775 BAU/mL is the accepted cutoff value,Citation21 165 BAU/mL was also adopted from a previous studyCitation20 since the present study included elderly patients with or without cancer, and the geometric mean of the antibody titer of these populations was considered relatively low. According to the international standard for anti-SARS-CoV-2 immunoglobulin released by the World health Organization,Citation22 manufacturers provided an assay-specific conversion factor for BAU/mL, which enables cross-comparison among different assays.Citation23 Based on the conversion factor suggested by the manufacturers,Citation24 the value of 165 BAU/mL was converted to 1,162 AU/mL (BAU/mL × 7.042) and 160 U/mL (BAU/mL × 0.971), and that of 775 BAU/mL to 5,458 AU/mL (BAU/mL × 7.042) and 753 U/mL (BAU/mL × 0.971) for the Architect and Elecsys assays, respectively. During data processing, anti-RBD antibody titer of 0 AU/mL was regarded as 0.1 AU/mL for the Architect assay. Anti-RBD antibody titer of <0.4 AU/mL and >2,500 was regarded as 0.4 and 2,500 for the Elecsys assay, respectively. Reciprocal antibody titers were analyzed after logarithmic transformation, and the results are presented on the original scale by calculating the antilogarithm. Stratified analyses were performed to examine the effects of the following potential confounders: age at vaccination (<70, 70–74, and ≥75 years), sex, group (lung cancer and non-cancer groups), and type of anticancer treatment. The significance of the fold-rise within a category was assessed using the Wilcoxon signed-rank test. Inter-category comparisons were made using the Wilcoxon rank-sum test or the Kruskal–Wallis test. When appropriate, the following tests were performed: Student’s t, Fisher’s exact, Jonckheere–Terpstra, and Cochran–Armitage tests. For example, the Student’s t-test was used to compare the age between lung cancer and non-cancer patients in , the Jonckheere–Terpstra test was used to evaluate trends in geometric mean antibody concentrations in age categories in , whereas the Cochran–Armitage test was used to evaluate the trend of the proportions of seropositivity and seroprotection in the age categories in . Additionally, the independent effects of potential confounders on antibody induction were assessed using logistic regression analysis. Models were constructed considering seropositivity or seroprotection as a dependent variable and the abovementioned potential confounders as explanatory variables. Odds ratios (ORs) and 95% confidence intervals (CIs) were calculated. All tests were two-sided, and all analyses were performed using R version 4.1.2 (R Foundation for Statistical Computing; http://www.r-project.org). Differences were considered significant at p < .05.
Table 1. Characteristics of study patients included in the immunogenicity analysis.
Table 2. Comparison of the geometric mean concentration (GMC) and GMC ratio of the anti-RBD antibodies among study participants stratified by age, sex, group, and the type of anticancer treatment.
Table 3. Seropositivity and seroprotection among study participants stratified by age, sex, group, and the type of anticancer treatment.
Results
shows the patient selection flow. In total, we enrolled 93 patients (55 lung cancer and 38 non-cancer patients) in this study. One lung cancer patient dropped out; another was positive for anti-N antibodies on the Architect assay, suggesting a previous infection before the first vaccination. After excluding these two patients, 91 patients completed the three blood collections up to 4 weeks after the second vaccination, and were evaluated for immunogenicity and safety as they were negative for anti-N antibodies in both assays in S0, S1, and S2. All study participants were completely vaccinated with the BNT162b2 COVID-19 vaccine (two doses administered 3–4 weeks apart).
lists a comparison of the characteristics between lung cancer patients and non-cancer patients included in the immunogenicity analysis. There was no difference between the lung cancer patients and non-cancer patients with chronic diseases in terms of their mean age. No differences existed in the proportion of men, history of allergies, or Eastern Cooperative Oncology Group performance status between lung cancer patients and non-cancer patients. The most frequent underlying diseases were respiratory disease, hypertension, and dyslipidemia in lung cancer and non-cancer patients. No significant differences (p ≥ .05) in underlying diseases existed between the two groups. Adenocarcinoma was the most common histological type of lung cancer. The most common anticancer therapy comprised tyrosine kinase inhibitors (TKIs), followed by immune-checkpoint inhibitors (ICIs).
lists the GMC and GMC ratio according to the age, sex, group, and type of anticancer treatment. In all subjects, the GMC of the BNT162b2 vaccine was 54 AU/mL (p < .001) on Architect and 2.0 U/mL (p < .001) on Elecsys after the first vaccination and 2,237 (p < .001) AU/mL on Architect and 322 U/mL (p < .001) on Elecsys after the second vaccination, indicating a significant increase. In the stratified analysis considering underlying disease, the GMCs of lung cancer patients were significantly lower than those of the non-cancer patients after the first (30 vs. 121 AU/mL, p < .001 on Architect; 4.0 vs 1.2 U/mL, p < .001, on Elecsys) and second vaccination (1632 vs. 3472 AU/mL, p = .005, on Architect; 213 vs 573 A/mL, p = .002, on Elecsys). depicts changes in the anti-RBD antibody titer before vaccination (S0), after first vaccination (S1), and after second vaccination (S2) between non-cancer and lung cancer patients. Supplementary Figure S1 shows the changes in the anti-RBD antibody titer when converted to BAU/mL. Regarding types of anticancer treatments, in lung cancer patients, a significant increase (p < .05) in GMC was observed after each of the two doses of the BNT162b2 vaccine for all treatment types on both Architect and Elecsys. After the second vaccination, the GMCs varied significantly (p < .05) for various treatments on both Architect and Elecsys; in particular, lung cancer patients receiving cytotoxic agents showed low values of 818 AU/mL and 72 U/mL for Architect and Elecsys, respectively. The GMC ratio of S2/S0 was significantly different (p = .002) among the types of anticancer treatment on Elecsys, whereas no significant difference (p = .145) on Architect was observed. The GMC in the Architect assay measured after the second vaccination (S2) showed a 4.2-fold difference (3472 [AU/mL]/818 [AU/mL]) between non-cancer patients and patients with lung cancer receiving cytotoxic drugs. However, when tested by the Elecsys assay, the difference was further increased by 8-fold (573 [U/mL]/72 [U/mL]). Reduced immunogenicity in lung cancer patients was more notable with the Elecsys assay compared with the Architect assay. shows changes in the anti-RBD antibody titer before vaccination (S0), and after the first (S1) and second (S2) vaccinations based on the types of anticancer treatment. Supplementary Figure S2 shows the changes in the anti-RBD antibody titer converted to BAU/mL A reduction in the anti-RBD titers in lung cancer patients receiving cytotoxic agents after the second vaccination (S2) was more pronounced in the results of the Elecsys assay than in those of the Architect assay based on the cut off for detection.
Figure 2. Changes in the anti-RBD antibody titer before vaccination (S0), after first vaccination (S1), and after second vaccination (S2) between non-cancer and lung cancer patients. A red triangle in each box shows the geometric mean titers, whereas the black horizontal line in the middle shows the median of the log-transformed antibody titers. A. Anti-RBD titer on Architect. B. Anti-RBD titer on Elecsys. The GMCs of lung cancer patients were significantly lower than those of the non-cancer patients after the first vaccination (30 vs. 121 AU/mL, p < .001 on Architect; 4.0 vs 1.2 U/mL, p < .001 on Elecsys) and second vaccination (1,632 vs. 3,472 AU/mL, p = .005 on Architect; 213 vs 573 A/mL, p = .002 on Elecsys).
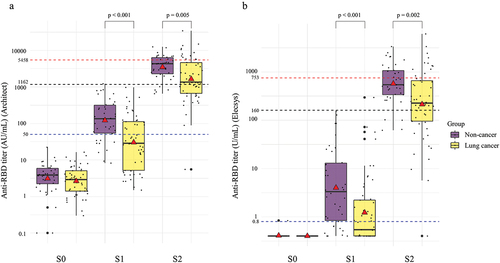
Figure 3. Changes in the anti-RBD antibody titer before vaccination (S0), after first vaccination (S1), and after second vaccination (S2) based on types of anticancer treatment. A red triangle in each box shows the geometric mean titers, whereas the black horizontal line in the middle shows the median of the log-transformed antibody titers. A. Anti-RBD titer on Architect. B. Anti-RBD titer on Elecsys.
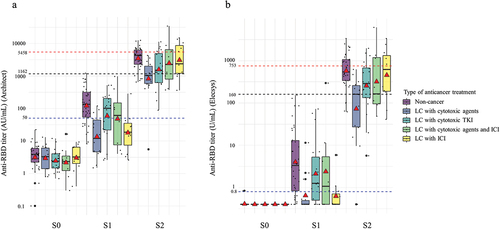
lists the seropositivity and seroprotection according to age, sex, group, and the type of anticancer treatment. Among all subjects, seropositivity on Architect and Elecsys was 54% and 58% after the first vaccination and 99% and 99% after the second vaccination, respectively. Regarding underlying diseases, after the first vaccination (S1), the lung cancer patients showed significantly lower rates of seropositivity compared with non-cancer patients (38% vs 76%, p < .001 on Architect; 45% vs 76%, p = .005 on Elecsys). After the second vaccine dose, the percentages of lung cancer patients and non-cancer patients with positive seropositivity showed no difference (98% vs 100%, p = 1.000 on Architect; 98% and 100%, p = 1.000 on Elecsys). However, the percentage of seroprotection in lung cancer patients was lower than that in non-cancer patients for the cutoff points ≥1,162 AU/mL (62% vs 87%, p = .016) on Architect and ≥160 U/mL (64% and 90%, p = .007) on Elecsys. In addition, there were significant differences (p < .05) in the percentages of seroprotection after the second vaccination among the types of anticancer treatments. Notably, the percentages of seroprotection in lung cancer patients with cytotoxic agents were low (43% for ≥1,162 AU/mL, 0% for ≥5,458 AU/mL on Architect; 50% for ≥160 U/mL, 0% for ≥753 U/mL on Elecsys), whereas those in lung cancer patients receiving ICIs were high (73% for ≥1,162 AU/mL, 46% for ≥5,458 AU/mL on Architect; 82% for ≥160 U/mL, 46% for ≥753 U/mL on Elecsys), which were similar to those in non-cancer patients.
lists the adjusted ORs for seropositivity and seroprotection after vaccination with the BNT162b2 vaccine. The ORs for seropositivity in lung cancer patients were significantly lower (p < .05) than those in non-cancer patients (0.20, 95% CI, 0.08–0.55 on Architect; 0.29, 95% CI, 0.11–0.75 on Elecsys) after the first vaccination. The ORs for seroprotection were significantly reduced (p < .05) in lung cancer patients after the second vaccination for the cutoff value of ≥1,162 AU/mL on Architect and ≥160 U/mL on Elecsys showing 0.22 (95% CI 0.07–0.69) and 0.20 (95% CI 0.06–0.68), respectively. Among the anticancer treatment types, the ORs for seropositivity in lung cancer patients receiving cytotoxic agents were reduced: 0.07 (95% CI: 0.01–0.37) for Architect and 0.09 (95% CI 0.02–0.44) for Elecsys after the first vaccination and 0.07 (95% CI: 0.01–0.40) for ≥1,162 AU/mL on Architect and 0.11 (95% CI 0.02–0.57) for ≥160 U/mL on Elecsys after the second vaccination. In contrast, the OR in lung cancer patients receiving ICIs after the second vaccination was 0.39 (0.06–2.28) for ≥1,162 U/mL on Architect and 0.59 (95% CI 0.08–4.23) for ≥160 U/mL on Elecsys, showing no significant difference (p ≥ .05) compared with that in non-cancer patients.
Table 4. Adjusted odds ratios for seropositivity and seroprotection after COVID-19 vaccination in non-cancer patients and lung cancer patients.
lists the adverse reactions to the BNT162b2 COVID-19 vaccination. There were no differences (p ≥ .05) in ocular or respiratory symptoms within 48 h, local reactions (within 48 h, and 48 h to 1 week), or systemic reactions (within 48 h, and 48 h to 1 week) between lung cancer patients and non-cancer patients after the first vaccination. Local reactions within 48 h post-vaccination were observed in 79% of lung cancer patients and 81% of non-cancer patients (p = 1.000). The most frequent local reaction within 48 h after the first vaccination was pain (72% in lung cancer patients and 78% in non-cancer patients, p = .624). Forty-two percent of lung cancer patients and 47% of non-cancer patients had systemic reactions within 48 h after the first vaccination (p = .666). No significant differences (p ≥ .05) in adverse reactions were noted after the second vaccination, except for itching being more common (p = .034) within 48 h in non-cancer patients. Local reactions within 48 h after the second vaccination increased compared with that after the first vaccination (83% in lung cancer patients and 84% in non-cancer patients, p = 1.000). Systemic reactions within 48 h after the second vaccination also increased compared with that after the first vaccination (65% of lung cancer patients and 68% of non-cancer patients, p = 1.000). The frequencies of fever (27% vs. 19%, p = .453), fatigue (36% vs. 43%, p = .516), and myalgia (26% vs. 38%, p = .259) in lung cancer patients vs. non-cancer patients, respectively, were particularly high. Most cases were mild and did not affect daily life.
Table 5. Adverse reactions to the BNT162b2 COVID-19 vaccination.
Discussion
Herein, we evaluated the immunogenicity and safety of the BNT162b2 COVID-19 vaccine after the first and second vaccinations in lung cancer patients and compared them with those in non-cancer patients. Lung cancer patients showed a significant increase in the GMC; however, the GMC was significantly lower in these patients than in non-cancer patients. In the multivariate analysis, the adjusted OR for seropositivity and seroprotection (≥1,162 AU/mL for Architect and ≥160 AU/mL for Elecsys) by the BNT162b2 vaccine was significantly lower (p < .05) in lung cancer patients than in non-cancer patients. In the analysis of the anticancer treatment types, the adjusted OR for seropositivity and seroprotection (≥1,162 AU/mL for Architect and ≥160 AU/mL for Elecsys) was significantly lower (p < .05) in lung cancer patients receiving cytotoxic agents than in non-cancer patients. Additionally, there was no increase in the number of adverse reactions in lung cancer patients compared with that in non-cancer patients.
Several studies have shown that the immunogenicity of the COVID-19 vaccine is reduced in cancer patients; moreover, inadequate antibody responses have been reported, especially in cancer patients vaccinated with a single dose.Citation12,Citation25,Citation26 A lower seroconversion rate of anti-spike IgG antibody has been reported especially in patients with hematologic malignancies and those receiving the anti-CD20 antibody.Citation25,Citation27,Citation28 Conversely, patients with solid tumors may have a sufficient seroconversion rate after two doses of mRNA vaccination.Citation12,Citation14,Citation25,Citation27 Studies using the same Abbott reagent for anti-RBD antibodies, as in our study, reported that completely vaccinated patients with solid tumors showed 90–98% of seropositivity at a cutoff value ≥50 AU/mL.Citation12,Citation14,Citation27 Here, we found 98% seropositivity at a cutoff value ≥50 AU/mL on Architect in lung cancer patients receiving anticancer treatment. Nevertheless, patients with solid tumors reportedly had a lower titer of anti-spike IgG than healthy subjects, even after complete vaccination.Citation12,Citation14,Citation29 Here, the GMC was significantly lower in lung cancer patients than in non-cancer patients, considering a stratified analysis.
Recent studies have shown an association between antibody titer and vaccine efficacy.Citation20,Citation21,Citation23,Citation30,Citation31 In our study, we adopted two cutoff points for seroprotection according to previous studies.20,Citation21 There was no difference in the percentage of seropositivity between lung cancer patients and non-cancer patients after two doses of vaccination; however, the percentage of seroprotection in lung cancer patients was lower than that in non-cancer patients with cutoff values of ≥1,162 AU/mL and ≥160 AU/mL for Architect and Elecsys, respectively. Furthermore, a significant decrease was observed in the adjusted OR for seroprotection with cutoff values of ≥1,162 AU/mL and ≥160 AU/mL for Architect and Elecsys, respectively. Compared to that in non-cancer patients, the immunogenicity of the COVID-19 vaccine in lung cancer patients undergoing anticancer treatment could be inadequate.
Several studies have reported that cytotoxic agents reduce the immunogenicity of COVID-19 vaccines in patients with solid tumor cancer.Citation14,Citation29,Citation32–34 Consistent with previous findings, in this study, the OR for seroprotection at cutoff values of ≥1,162 AU/mL for Architect and ≥160 U/mL for Elecsys after two vaccination doses was significantly decreased in lung cancer patients undergoing treatment with cytotoxic agents. A cytotoxic agent is designed to destroy rapidly growing tumor cells, but will inevitably also impair hematopoiesis. Moreover, the full functional capacity of immune cells depends on the clonal expansion of antigen specific lymphocytes and is therefore adversely affected.Citation35 Thus, cytotoxic agents may inhibit antibody production. Additionally, there are limited data on whether TKIs affect the immunogenicity of COVID-19 vaccines.Citation14,Citation32 In a study evaluating patients with thoracic cancer using the Abbott reagent used in the present study, TKI treatment was associated with a reduced antibody response to BNT162b2 COVID-19 vaccine compared with that in health controls.Citation14 Similarly, in our study, the adjusted OR for ≥1,162 AU/mL on Architect and ≥160 AU/mL on Elecsys after two vaccination doses tended to decrease in patients treated with TKIs, although not significantly (p ≥ .05). Several studies have reported that ICIs do not decrease the immunogenicity of COVID-19 vaccines.Citation27,Citation36 Notably, the adjusted ORs for seroprotection in patients receiving ICIs were 0.39 (0.06–2.28) for ≥1,162 U/mL on Architect and 0.59 (95% CI 0.08–4.23) for ≥160 U/mL on Elecsys after the second vaccination, which did not decrease as compared with that in non-cancer patients.
The GMC ratio of S2/S0 was significantly different between groups and among types of anticancer treatment on Elecsys, whereas it was insignificant on Architect. In addition, a more exaggerated fold difference in the Elecsys assay antibody titer between non-cancer patients and patients with lung cancer receiving cytotoxic agents was observed after the second vaccination (S2). First, this may reflect the assay-specific handling of values below the limit of detection and setting of the zero-value baseline; the limit of detection per manufacturers’ definitions is set at 0.35 U/mL for the Elecsys and 6.8 AU/mL for the Architect assay. Secondly, it is of note that the Elecsys assay uses a double-antigen sandwich method,Citation16 which in other studies has been shown to better detect antibodies with higher avidity,Citation37,Citation38 compared with the two-step Architect immunoassay.Citation15 The avidity was determined by the colorimetric comparison of optical density values obtained using enzyme-linked immunosorbent assay with and without the addition of a 5.5 M urea treatment step.Citation38 Third, the difference in epitopeCitation39 targeted by the two assays might also have affected the measured titers. A recent study demonstrated the different kinetics of the two immunoassays in healthy adults.Citation40 In the study, the anti-RBD titers after six months from the second vaccination were more attenuated in Architect (Abbott) than in Elecsys (Roche) even though the changes in antibody titers were similar until one month after the second vaccination. The authors speculated that the attenuation of antibodies in Elecsys was less than that in Architect owing to the detection of antibodies with higher avidity by Elecsys because time intervals and additional vaccine doses increase the maturation and activity of antibodies induced against the target antigen.Citation41 In this study, we only evaluated the short-term immunogenicity and safety. High avidity IgG reportedly plays an important role in immunity against SARS-CoV-2.Citation42 Further studies are thus needed to evaluate the kinetics of antibody titers in immunocompromised patients, such as patients with cancer, to determine differences in assays and the longitudinal changes, such as after 6 months post-vaccination.
The short-term safety of the COVID-19 vaccine in cancer patients undergoing treatment is reportedly comparable to that in healthy subjects.Citation29 In a review of seven studies-three including patients with hematologic malignancies, two including those with solid tumors, and two including those with both types of cancer – the authors reported that frequencies of local and systemic reactions did not differ between cancer patients and healthy subjects after partial and full vaccination.Citation29 A large cohort study that evaluated 1,753 patients, including 1,094 with solid tumors and 89 with hematologic malignancies, revealed few differences in the frequency of adverse reactions between cancer and non-cancer patients.Citation43 Here, no increase in adverse reactions was observed in patients with lung cancer after either the first or second vaccination dose.
Furthermore, we observed a significant increase in antibody titers and an acceptable safety level of BNT162b2 COVID-19 vaccination in lung cancer patients undergoing anticancer treatment, which could support the use of COVID-19 vaccination in this patient group. However, compared with that in non-cancer patients, immunogenicity may be inadequate. Further studies are needed to determine whether increased mRNA dosage, vaccine types, mixing vaccine types, vaccination timing, or additional doses enhance immunogenicity in cancer patients under treatment. Cancer patients should continue to be prioritized for vaccination. Studies evaluating the immunogenicity of COVID-19 vaccination in cancer patients are increasing in number; however, only a few have evaluated clinical outcomes such as the incidence of infection, hospitalization, and death.Citation25,Citation44,Citation45 Further studies are needed to evaluate the effectiveness of COVID-19 vaccination in cancer patients considering the various cancer types, administered vaccine, type of anticancer treatment, number of doses, timing of vaccination, mixed vaccination, immunogenicity by assay method, and clinical outcomes such as incidence of COVID-19 and death attributable to COVID-19. Even after vaccination, infection control measures, including universal wearing of masks and social distancing, continue to be important for lung cancer patients undergoing anticancer treatment.
Our study has some limitations. First, the sample size was small. Nevertheless, this was a multicenter study and, to date, only a few have focused on lung cancer patients alone. The sample size of 53 lung cancer patients was relatively large compared with those in previous studies, and we were able to undertake a multivariate analysis considering various types of anticancer treatment. Second, we did not evaluate the cellular immunity associated with the COVID-19 vaccination. However, the anti-RBD antibody titer evaluated here is reportedly correlated with the efficacy of the COVID-19 vaccination.Citation20,Citation21,Citation23 Third, only short-term immunogenicity and safety were evaluated here. However, as this study is ongoing, antibody titers and adverse events, including immune-related events, at 6 months after the second vaccination will be reported in a future paper.
In conclusion, BNT162b2 vaccination in lung cancer patients undergoing anticancer treatment significantly increased the antibody titers with acceptable safety. Compared to non-cancer patients, the COVID-19 RBD immunogenicity in the lung cancer patients could be inadequate. Further studies and infection control measures to protect lung cancer patients from COVID-19 are therefore warranted.
Credit statement
K.N. contributed to conceptualization, data curation, formal analysis, investigation, methodology, project administration, software, validation, visualization, writing – original draft, and writing – review & editing. M.I. contributed to conceptualization, investigation, resources, methodology, project administration, and writing – review & editing. H.M. contributed to conceptualization, data curation, formal analysis, methodology, software, validation, visualization, and writing – review & editing. C.Y., T.N. M.S., H.N., and Y.O contributed to investigation, resources, and writing – review & editing. Y.N. contributed to funding acquisition, investigation, resources, visualization, and writing – review & editing. N.K. and Y.N. contributed to investigation, resources, and writing – review & editing. Y.K. contributed conceptualization, funding acquisition, investigation, resources, visualization, and writing – review & editing. Y.H. contributed to conceptualization, funding acquisition, methodology, supervision, and writing – review & editing. All authors have read and approved the final draft of the manuscript.
Supplemental Material
Download PDF (267.8 KB)Acknowledgments
We thank the staff of the Department of Pulmonary Medicine and Laboratory Medicine at Kameda Medical Center and Chikamori Hospital for their involvement in this study.
Disclosure statement
No potential conflict of interest was reported by the author(s).
Supplementary material
Supplemental data for this article can be accessed on the publisher’s website at https://doi.org/10.1080/21645515.2022.2140549
Additional information
Funding
References
- Zhu N, Zhang D, Wang W, Li X, Yang B, Song J, Zhao X, Huang B, Shi W, Lu R, et al. A novel coronavirus from patients with pneumonia in China, 2019. N Engl J Med. 2020;382(8):1–13. doi:10.1056/NEJMoa2001017.
- The Lancet O. COVID-19: global consequences for oncology. Lancet Oncol. 2020;21:467. doi:10.1016/S1470-2045(20)30175-3.
- Desai A, Gupta R, Advani S, Ouellette L, Kuderer NM, Lyman GH, Li A. Mortality in hospitalized patients with cancer and coronavirus disease 2019: a systematic review and meta‐analysis of cohort studies. Cancer. 2021;127(9):1459–68. doi:10.1002/cncr.33386.
- Liang W, Guan W, Chen R, Wang W, Li J, Xu K, Li C, Ai Q, Lu W, Liang H, et al. Cancer patients in SARS-CoV-2 infection: a nationwide analysis in China. Lancet Oncol. 2020;21(3):335–37. doi:10.1016/S1470-2045(20)30096-6.
- Dai M, Liu D, Liu M, Zhou F, Li G, Chen Z, Zhang Z, You H, Wu M, Zheng Q, et al. Patients with cancer appear more vulnerable to SARS-CoV-2: a multicenter study during the COVID-19 outbreak. Cancer Discov. 2020;10(6):783–91. doi:10.1158/2159-8290.CD-20-0422.
- Wang Q, Berger NA, Xu R. Analyses of risk, racial disparity, and outcomes among US patients with cancer and COVID-19 Infection. JAMA Oncol. 2020;7(2):220. doi:10.1001/jamaoncol.2020.6178.
- Garg S, Patel K, Pham H, Whitaker M, O’Halloran A, Milucky J, Anglin O, Kirley PD, Reingold A, Kawasaki B, et al. Clinical trends among U.S. adults hospitalized with COVID-19, March to December 2020 : a cross-sectional study. Ann Intern Med. 2021;174(10):1409–19. doi:10.7326/M21-1991.
- Polack FP, Thomas SJ, Kitchin N, Absalon J, Gurtman A, Lockhart S, Perez JL, Pérez Marc G, Moreira ED, Zerbini C, et al. Safety and efficacy of the BNT162b2 mRNA Covid-19 vaccine. N Engl J Med. 2020;383(27):2603–15. doi:10.1056/NEJMoa2034577.
- Baden LR, El Sahly HM, Essink B, Kotloff K, Frey S, Novak R, Diemert D, Spector SA, Rouphael N, Creech CB, et al. Efficacy and safety of the mRNA-1273 SARS-CoV-2 vaccine. N Engl J Med. 2021;384(5):403–16. doi:10.1056/NEJMoa2035389.
- Voysey M, Clemens SAC, Madhi SA, Weckx LY, Folegatti PM, Aley PK, Angus B, Baillie VL, Barnabas SL, Bhorat QE, et al. Safety and efficacy of the ChAdox1 nCov-19 vaccine (AZD1222) against SARS-CoV-2: an interim analysis of four randomised controlled trials in Brazil, South Africa, and the UK. Lancet. 2021;397(10269):99–111. doi:10.1016/S0140-6736(20)32661-1.
- Ribas A, Sengupta R, Locke T, Zaidi SK, Campbell KM, Carethers JM, Jaffee EM, Wherry EJ, Soria J-C, D’Souza G. Priority COVID-19 vaccination for patients with cancer while vaccine supply is limited. Cancer Discov. 2021;11(2):233–36. doi:10.1158/2159-8290.CD-20-1817.
- Massarweh A, Eliakim-Raz N, Stemmer A, Levy-Barda A, Yust-Katz S, Zer A, Benouaich-Amiel A, Ben-Zvi H, Moskovits N, Brenner B, et al. Evaluation of seropositivity following BNT162b2 messenger RNA vaccination for SARS-CoV-2 in patients undergoing treatment for cancer. JAMA Oncol. 2021;7(8):1133. doi:10.1001/jamaoncol.2021.2155.
- Di Noia V, Pimpinelli F, Renna D, Barberi V, Maccallini MT, Gariazzo L, Pontone M, Monti A, Campo F, Taraborelli E, et al. Immunogenicity and safety of COVID-19 vaccine BNT162b2 for patients with solid cancer: a large cohort prospective study from a single institution. Clin Cancer Res. 2021;27(24):6815–23. doi:10.1158/1078-0432.CCR-21-2439.
- Gounant V, Ferré VM, Soussi G, Charpentier C, Flament H, Fidouh N, Collin G, Namour C, Assoun S, Bizot A, et al. Efficacy of severe acute respiratory syndrome coronavirus-2 vaccine in patients with thoracic cancer: a prospective study supporting a third dose in patients with minimal serologic response after two vaccine doses. J Thorac Oncol. 2022;17(2):239–51. doi:10.1016/j.jtho.2021.10.015.
- Abbott ARCHITECT SARS-CoV-2 IgG II quant reagent -instructions for use- US food and drug administration. [accessed 2022 Sep 23]. https://www.fda.gov/media/146371/download.
- Elecsys anti-CoV-2 S -instructions for use- US food and drug administration. [accessed 2022 May 1]. https://www.fda.gov/media/137605/download.
- Oculo-respiratory syndrome following influenza vaccination: review of post-marketing surveillance through four influenza seasons in Canada. Can Commun Dis Rep. 2005;31(21):217–25.
- National Advisory Committee on I. An Advisory Committee Statement (ACS). National Advisory Committee on Immunization (NACI). Supplementary statement on influenza vaccination: continued use of fluviral influenza vaccine in the 2000-2001 season. Can Commun Dis Rep. 2001;27:1–3.
- Products. ECfPM. Note for guidance on harmonisation of requirements for influenza vaccines (CPMP/BWP/214/96). London: European Agency for the Evaluation of Medical Products; 1997. [accessed 2015 Feb 23]. http://www.ema.europa.eu/docs/en_GB/document_library/Scientific_guideline/2009/09/WC500003945.pdf.
- Feng S, Phillips DJ, White T, Sayal H, Aley PK, Bibi S, Dold C, Fuskova M, Gilbert SC, Hirsch I, et al. Correlates of protection against symptomatic and asymptomatic SARS-CoV-2 infection. Nat Med. 2021;27(11):2032–40. doi:10.1038/s41591-021-01540-1.
- Gilbert PB, Montefiori DC, McDermott AB, Fong Y, Benkeser D, Deng W, Zhou H, Houchens CR, Martins K, Jayashankar L, et al. Immune correlates analysis of the mRNA-1273 COVID-19 vaccine efficacy clinical trial. Sci (New York, NY). 2022;375(6576):43–50. doi:10.1126/science.abm3425.
- First WHO International Standard for anti-SARS-CoV-2 immunoglobulin (human). [accessed 2022 Sep 21]. https://www.nibsc.org/documents/ifu/20-136.pdf.
- Knezevic I, Mattiuzzo G, Page M, Minor P, Griffiths E, Nuebling M, Moorthy V. WHO International Standard for evaluation of the antibody response to COVID-19 vaccines: call for urgent action by the scientific community. Lancet Microbe. 2022;3(3):e235–40. doi:10.1016/S2666-5247(21)00266-4.
- Lukaszuk K, Kiewisz J, Rozanska K, Dabrowska M, Podolak A, Jakiel G, Woclawek-Potocka I, Lukaszuk A, Rabalski L. Usefulness of IVD kits for the assessment of SARS-CoV-2 antibodies to evaluate the humoral response to vaccination. Vaccines. 2021;9(8):840. doi:10.3390/vaccines9080840.
- Becerril-Gaitan A, Vaca-Cartagena BF, Ferrigno AS, Mesa-Chavez F, Barrientos-Gutiérrez T, Tagliamento M, Lambertini M, Villarreal-Garza C. Immunogenicity and risk of SARS-CoV-2 infection after COVID-19 vaccination in patients with cancer: a systematic review and meta-analysis. Eur J Cancer. 2022;163:243–260.
- Barrière J, Chamorey E, Adjtoutah Z, Castelnau O, Mahamat A, Marco S, Petit E, Leysalle A, Raimondi V, Carles M. Impaired immunogenicity of BNT162b2 anti-SARS-CoV-2 vaccine in patients treated for solid tumors. Ann Oncol. 2021;32(8):1053–55. doi:10.1016/j.annonc.2021.04.019.
- Thakkar A, Gonzalez-Lugo JD, Goradia N, Gali R, Shapiro LC, Pradhan K, Rahman S, Kim SY, Ko B, Sica RA, et al. Seroconversion rates following COVID-19 vaccination among patients with cancer. Cancer Cell. 2021;39(8):1081–90.e2. doi:10.1016/j.ccell.2021.06.002.
- Takakuwa T, Nakagama Y, Yasugi M, Maeda T, Matsuo K, Kiritoshi A, Deguchi R, Hagawa N, Shibata W, Oshima K, et al. Discrepant antigen-specific antibody responses causing SARS-CoV-2 persistence in a patient receiving B-cell-targeted therapy with rituximab. Int Med. 2021;60(23):3827–31. doi:10.2169/internalmedicine.7884-21.
- Tran S, Truong TH, Narendran A. Evaluation of COVID-19 vaccine response in patients with cancer: an interim analysis. Eur J Cancer. 2021;159:259–74. doi:10.1016/j.ejca.2021.10.013.
- Khoury DS, Cromer D, Reynaldi A, Schlub TE, Wheatley AK, Juno JA, Subbarao K, Kent SJ, Triccas JA, Davenport MP. Neutralizing antibody levels are highly predictive of immune protection from symptomatic SARS-CoV-2 infection. Nat Med. 2021;27(7):1205–11. doi:10.1038/s41591-021-01377-8.
- Goldblatt D, Fiore-Gartland A, Johnson M, Hunt A, Bengt C, Zavadska D, Snipe HD, Brown JS, Workman L, Zar HJ, et al. Towards a population-based threshold of protection for COVID-19 vaccines. Vaccine. 2022;40(2):306–15. doi:10.1016/j.vaccine.2021.12.006.
- Corti C, Antonarelli G, Scotté F, Spano JP, Barrière J, Michot JM, André F, Curigliano G. Seroconversion rate after vaccination against COVID-19 in patients with cancer—a systematic review. Ann Oncol. 2021;33(2):158–68. doi:10.1016/j.annonc.2021.10.014.
- Palich R, Veyri M, Marot S, Vozy A, Gligorov J, Maingon P, Marcelin A-G, Spano J-P. Weak immunogenicity after a single dose of SARS-CoV-2 mRNA vaccine in treated cancer patients. Ann Oncol. 2021;32(8):1051–53. doi:10.1016/j.annonc.2021.04.020.
- Webber TB, Provinciali N, Musso M, Ugolini M, Boitano M, Clavarezza M, D’Amico M, Defferrari C, Gozza A, Briata IM, et al. Predictors of poor seroconversion and adverse events to SARS-CoV-2 mRNA BNT162b2 vaccine in cancer patients on active treatment. Eur J Cancer. 2021;159:105–12. doi:10.1016/j.ejca.2021.09.030.
- Wumkes ML, van der Velden AM, Los M, Leys MB, Beeker A, Nijziel MR, van der Velden AWG, Westerman M, Meerveld-Eggink A, Rimmelzwaan GF, et al. Serum antibody response to influenza virus vaccination during chemotherapy treatment in adult patients with solid tumours. Vaccine. 2013;31(52):6177–84. doi:10.1016/j.vaccine.2013.10.053.
- Ma Y, Liu N, Wang Y, Zeng J, Hu Y-Y, Hao W, Shi H, Zhu P, Lv J, Fan W, et al. Immune checkpoint blocking impact and nomogram prediction of COVID-19 inactivated vaccine seroconversion in patients with cancer: a propensity-score matched analysis. J ImmunoTher Cancer. 2021;9(11):e003712. doi:10.1136/jitc-2021-003712.
- Nakagama Y, Nitahara Y, Kaku N, Tshibangu-Kabamba E, Kido Y. A dual-antigen SARS-CoV-2 serological assay reflects antibody avidity. J Clin Microbiol. 2022;60(2):e0226221. doi:10.1128/jcm.02262-21.
- Pichler D, Baumgartner M, Kimpel J, Rössler A, Riepler L, Bates K, Fleischer V, von Laer D, Borena W, Würzner R. Marked increase in avidity of SARS-CoV-2 antibodies 7–8 months after infection is not diminished in old age. J Infect Dis. 2021;224(5):764–70. doi:10.1093/infdis/jiab300.
- Nitahara Y, Nakagama Y, Kaku N, Candray K, Michimuko Y, Tshibangu-Kabamba E, Kaneko A, Yamamoto H, Mizobata Y, Kakeya H, et al. High-resolution linear epitope mapping of the receptor binding domain of SARS-CoV-2 spike protein in COVID-19 mRNA vaccine recipients. Microbiol Spectr. 2021;9(3):e0096521. doi:10.1128/Spectrum.00965-21.
- Matsuura T, Fukushima W, Nakagama Y, Kido Y, Kase T, Kondo K, Kaku N, Matsumoto K, Suita A, Komiya E, et al. Kinetics of anti-SARS-CoV-2 antibody titer in healthy adults up to 6 months after BNT162b2 vaccination measured by two immunoassays: a prospective cohort study in Japan. Vaccine. 2022;40(38):5631–40. doi:10.1016/j.vaccine.2022.08.018.
- Perez-Saez J, Zaballa M-E, Yerly S, Andrey DO, Meyer B, Eckerle I, Balavoine J-F, Chappuis F, Pittet D, Trono D, et al. Persistence of anti-SARS-CoV-2 antibodies: immunoassay heterogeneity and implications for serosurveillance. Clin Microbiol Infect. 2021;27(11):1695.e7–.e1. doi:10.1016/j.cmi.2021.06.040.
- Bauer G. The potential significance of high avidity immunoglobulin G (IgG) for protective immunity towards SARS-CoV-2. Int J Infect Dis. 2021;106:61–64. doi:10.1016/j.ijid.2021.01.061.
- Shulman RM, Weinberg DS, Ross EA, Ruth K, Rall GF, Olszanski AJ, Helstrom J, Hall MJ, Judd J, Chen DYT, et al. Adverse events reported by patients with cancer after administration of a 2-dose mRNA COVID-19 vaccine. J Natl Compr Canc Net. 2022;20(2):160–66. doi:10.6004/jnccn.2021.7113.
- Marra AR, Kobayashi T, Suzuki H, Alsuhaibani M, Tofaneto BM, Bariani LM, Auler MDA, Salinas JL, Edmond MB, Doll M, et al. Short-term effectiveness of COVID-19 vaccines in immunocompromised patients: a systematic literature review and meta-analysis. J Infect. 2022;84(3):297–310. doi:10.1016/j.jinf.2021.12.035.
- Chodick G, Tene L, Rotem RS, Patalon T, Gazit S, Ben-Tov A, Weil C, Goldshtein I, Twig G, Cohen D, et al. The effectiveness of the two-dose BNT162b2 vaccine: analysis of real-world data. Clin Infect Dis. 2022;74(3):472–78. doi:10.1093/cid/ciab438.