ABSTRACT
The global supply of COVID-19 vaccines has been limited, and concerns have arisen about vaccine supply chain disruptions in developing countries. Heterologous prime-boost vaccination, which involves using different vaccines for the first and second doses, has been proposed to enhance the immune response. We aimed to compare the immunogenicity and safety of a heterologous prime-boost vaccination using an inactivated COVID-19 vaccine and AZD1222 vaccine with that of a homologous vaccination using AZD1222. This pilot involved 164 healthy volunteers without prior SARS-CoV-2 infection aged 18 years or older assigned to receive either the heterologous or homologous vaccination. The results showed that the heterologous approach was safe and well-tolerated, although the reactogenicity of the heterologous approach was higher. At 4 weeks after receiving the booster dose, the heterologous approach elicited a non-inferior immune response compared to the homologous approach in neutralizing antibody and cell-mediated immune response. The percentage of inhibition was 83.88 (79.72–88.03) in the heterologous and 79.88 (75.50–84.25) in the homologous group, a mean difference of 4.60 (−1.67–10.88). The geometric mean of interferon-gamma was 1072.53 mIU/mL (799.29–1439.18) in the heterologous group and 867.67 mIU/mL (671.94–1120.40) in the homologous group, a GMR of 1.24 (0.82–1.85). However, the binding antibody test of the heterologous group was inferior to the homologous group. Our findings suggest that the use of heterologous prime-boost vaccination with different types of COVID-19 vaccines is a viable strategy, especially in settings where vaccine supply is limited or where vaccine distribution is challenging.
Introduction
The ongoing COVID-19 pandemic has created an urgent need for effective vaccination strategies to prevent the spread of the virus and reduce the burden on healthcare systems worldwide. Multiple vaccines, including mRNA-based vaccines, inactivated virus vaccines, and viral vector-based vaccines, have been developed and approved for use.Citation1 Most of these vaccines require a two-dose regimen to achieve maximum protection against COVID-19.Citation2–6 However, the global supply of vaccines has been limited, and concerns have arisen about vaccine supply chain disruptions, especially in developing countries.Citation7
The heterologous prime-boost vaccination strategy, which involves the use of different vaccines for the first and second doses, has been proposed to enhance the immune response against SARS-CoV-2. Although the mechanism underlying this enhanced immunogenicity is not fully understood, it is thought to be due to the activation of different arms of the immune system, resulting in a more robust and diverse immune response. In addition, this approach prevents the vector antibody of the initial vaccine in the vector vaccine.Citation8 For example, a heterologous prime-boost regimen using an adenovirus vector vaccine for the first dose and an mRNA vaccine for the second dose has been shown to elicit a strong humoral and cellular immune response against SARS-CoV-2. It is believed that the adenovirus vector vaccine stimulates a strong cellular immune response, while the mRNA vaccine primarily elicits a robust humoral immune response. Combining these different types of vaccines may result in a more comprehensive immune response.Citation9 While studies have demonstrated the immunogenicity of heterologous prime-boost vaccination regimens using mRNA and adenovirus vector vaccines, there is currently limited solid evidence regarding the immunogenicity of a heterologous prime-boost strategy between inactivated and adenovirus vector vaccines in terms of both humoral and cell-mediated immune response.Citation8,Citation10
Therefore, in this pilot study, we aimed to compare the immunogenicity and safety of a heterologous prime-boost vaccination regimen using inactivated and adenoviral vectors with that of a homologous vaccination regimen using adenoviral vector vaccine for both doses. The results of this study could have important implications for the design of COVID-19 vaccination programs, especially in settings where vaccine supply is limited or where vaccine distribution is challenging. By demonstrating the non-inferiority of a heterologous vaccination regimen, this study could help to expand the use of available vaccines and improve vaccination coverage, which is critical to controlling the COVID-19 pandemic.
Materials & methods
Study design and participants
This is a single-center, open-label, non-inferiority, quasi-experimental study designed to demonstrate the immunogenicity, safety, and longitudinal dynamic of a heterologous prime-boost vaccination using inactivated COVID-19 vaccines (CoronaVac, Sinovac Biotech, Beijing, China or BBIBP-CorV, Beijing Bio-Institute of Biological Products, Beijing, China) and an adenoviral vector vaccine (AZD1222, developed by Oxford University and produced by AstraZeneca). The study included two intervention groups: the heterologous and homologous groups. In the heterologous group, the participants received the inactivated COVID-19 vaccine followed by the AZD1222 vaccine at 4-week intervals. In the homologous group, participants received 2 doses of the AZD1222 vaccine at 8-week intervals. The study was conducted at Chulabhorn Hospital, Chulabhorn Royal Academy, Bangkok, Thailand, between August 10 and February 10, 2022. Participants were allowed to choose their vaccine regimen.
The study enrolled healthy participants who were ≥18 years of age and had no history of SARS-CoV-2 vaccination or infection. Patients were excluded if they were pregnant or lactating, had uncontrolled underlying diseases, had respiratory tract infection symptoms or fever 14 days before enrollment, or had received any vaccine within 14 days before enrollment. In addition, participants evaluated their COVID-19 history before receiving the booster vaccine. All participants provided written informed consent before enrollment. The study protocol, consent form, and case records form were reviewed and approved by the Chulabhorn Ethics Committee (reference number: 057/2564), and the trial was registered with thaiclinicaltrials.org (TCTR20210714003).
Intervention and procedures
The BBIBP-CorV vaccine from Beijing Bio-Institute of Biological Products Co Ltd, China contained 4 μg of SARS-CoV-2 virion and 0.45 mg of aluminum, while the CoronaVac vaccine contained 3 μg of SARS-CoV-2 virion and 0.45 mg/mL of aluminum.Citation5,Citation6,Citation11 The AZD1222 vaccine from SK Bioscience Co Ltd, Republic of Korea, contained 5 × 1010 viral particles.Citation4 All vaccines were administered intramuscularly into the deltoid muscle using the standard dose. These vaccines were approved by Thailand’s regulatory agency and were manufactured and vialled following Good Manufacturing Practices guidelines.
After enrollment, the baseline spike protein anti-receptor binding domain (RBD) antibody levels of all participants were measured, and participants with seropositive status at baseline were excluded from the analysis. Immune responses were measured at two time points, 4 and 4 months after the booster dose. At 4 weeks after the second dose, both the humoral immune and cell-mediated immune responses were assessed. The humoral immune response was measured using the total antibody of the spike protein receptor binding domain (RBD) and surrogate neutralizing antibody (sNT), while the cell-mediated immune response was measured by interferon-gamma releasing assay (IGRA). At 4 months, only the total antibody of the spike protein RBD and sNT were measured.
Sample size calculation
The sample size calculation was based on a non-inferiority trial design, with the hypothesis that immunization with a heterologous prime-boost vaccination is not inferior to a homologous prime-boost vaccination. The geometric mean concentration of antibodies after heterologous prime-boost with CoronaVac and AZD1222 was 736.1 BAU/mL (95%CI, 486.0–1115), while the geometric mean concentration after homologous AZD1222 vaccination was 954.5 BAU/mL (95%CI, 886.5–1028), based on data from healthcare workers in our institute. Non-inferiority was defined as a lower limit of the two-sided 95% confidence interval of geometric mean ratio > 0.67. To achieve a power of 80%, with a significance level of 5% (α = 0.05), and accounting for a 20% participant dropout rate, a total of 154 participants were enrolled, with 77 participants in each of the heterologous and homologous groups.
Statistical analysis
The anti-RBD antibody levels, the percentage of inhibition of sNT, and the interferon-gamma level between the heterologous and homologous groups were compared using a multiple linear regression model. For anti-RBD and interferon-gamma level, before hypothesis testing, the level was log-transformed, and the difference of arithmetic means was then converted to the geometric mean ratio (GMR) by anti-logarithm. The non-inferiority criterion was met if the lower bound of the two-sided 95% confidence interval (CI) of the GMR was greater than 0.67 for anti-RBD and interferon-gamma level.Citation12 For the percentage of inhibition of sNT, the non-inferiority criterion was met if the lower bound of the two-sided 95% confidence interval (CI) of the mean difference was less than 10%. If non-inferiority was established, superiority was then assessed. Summary statistics were presented as mean and standard deviations, medians and interquartile ranges (IQRs), or geometric mean concentrations and 95% CIs as appropriate. The statistical analyses for this study were conducted using IBM SPSS version 26 and GraphPad Prism version 9. A p-value of less than .05 was considered statistically significant.
Endpoints
The primary endpoint was the GMR of anti-RBD concentration between the heterologous and homologous groups at 4 weeks after the booster dose. The secondary endpoints were reactogenicity on 1- and 7-days after each dose of vaccination, as well as the difference in mean percentage of inhibition of sNT at 4 weeks, the GMR of interferon-gamma at 4 weeks, the GMR of anti-RBD at 4 months, and the difference in mean percentage of inhibition of sNT at 4 months.
Safety
All participants were monitored for immediate adverse events for at least 15 minutes after each vaccination at the vaccine clinic. In addition, participants were asked to complete online questionnaires through a short message service to assess reactogenicity at 1- and 7-days post-vaccination. The severity of reactogenicity was graded as mild, moderate, severe, or potentially life-threatening. Mild reactogenicity was defined as not interfering with daily activity or a local reaction less than 5 cm, while moderate reactogenicity was defined as some interference with daily activity or a local reaction of 5.1 to less than 10 cm. Severe reactogenicity was defined as significant interference with daily activity or a local reaction of 10 cm or more. Potentially life-threatening reactogenicity was defined as requiring emergency care or hospitalization or a local reaction including necrosis or exfoliative dermatitis.
Anti-RBD antibody measurements
The immunogenicity was measured using the Elecsys Anti-SARS-CoV-2 S kit, which is a binding antibody assay designed to detect total antibodies against the spike protein receptor-binding domain (RBD) of wild-type SARS-CoV-2. The manufacturer’s cutoff value for a positive result was >0.8 U/mL. However, the report in this study used the binding antibody units (BAU/mL) based on the WHO international standard for anti-SARS-CoV-2 immunoglobulin concentration, using the manufacturer’s conversion factor (Elecsys-S U = 0.972 × BAU). The linearity range was 0.40–250 U/mL.Citation13 The binding antibody assays correlated well with the neutralizing antibody test, which is a standard method.Citation14
Surrogate virus neutralization test (sNT)
Measuring vaccine-induced protection against viral infections typically requires neutralizing tests, but these traditional methods involve complicated and time-consuming procedures. To overcome these constraints, the study employed a surrogate virus neutralization test (sNT) that has been shown to be highly correlated with traditional neutralizing tests.Citation15 The sNT used in this study was SARS-CoV-2 NeutraLISA (Euroimmun Medizinische Labordiagnostika AG, Lübeck, Germany). The test measures the ability of neutralizing antibodies to block the interaction between the spike protein RBD and recombinant human ACE2. The test is performed on a solid-phase coated with RBD, and biotinylated ACE2 is added to bind the RBD. If neutralizing antibodies are present in the sample, they will bind to the spike protein RBD and prevent the reaction of the recombinant human ACE2. After incubation with the specimen, unbound ACE2 is washed away, and the resulting bound ACE2 is identified using peroxidase-labeled streptavidin. The color intensity generated is inversely proportional to the level of inhibition. According to the manufacturer’s guidelines, a positive outcome is defined as a percentage of inhibition greater than or equal to 35%.
Interferon-gamma releasing assay (IGRA)
The immune response involving T cells is crucial for controlling SARS-CoV-2 infection, and interferon-gamma is a critical cytokine secreted by helper and cytotoxic T cells. This cytokine is a reliable surrogate marker for cell-mediated immune responses.Citation9 To assess the T cell response in this study, an interferon-gamma ELISA was used (Euroimmun Medizinische Labordiagnostika AG, Lübeck, Germany). The assay was used to determine SARS-CoV-2 S1 specific T cell activity using heparinized whole blood as the specimen.
The ELISA kit used in the assay consisted of three reaction tubes, with the first tube serving as the background level of interferon-gamma released and the second tube containing the S1 domain of the SARS-CoV-2 spike protein. The third tube contained mitogen to act as an unspecific antigen control indicating T cell stimulation ability. After incubation for 20–24 hours at 37 ± 1°C, the amount of interferon-gamma released into the plasma was measured by ELISA. The measurement range provided by the manufacturer was 3.88–350 mIU/mL. The interferon-gamma level higher than 350 mIU/mL required further sample dilution.
Results
The study enrolled a total of 164 participants between August and September 2021, with 87 participants in the heterologous group and 77 participants in the homologous group. The baseline demographic and clinical of participants were demonstrated in . Four participants were excluded due to positive serostatus at baseline, three participants in the heterologous group and one participant in the homologous group. These participants were excluded from the immunogenicity analysis. However, they will be included in the safety analysis. There was a significant lower proportion of females in the heterologous group, and the participants in this group trended to be older, although this was not statistically significant. All participants were followed up 4 weeks after receiving the booster dose. However, one-third of the participants (50 out of 160) were lost to follow-up at 4 months. There was no participant diagnosed with COVID-19 during the prime and boost vaccination period. A summarization of the participants’ flow was demonstrated in .
Figure 1. Participants’ flow. Between August and September 2021, a total of 164 participants were assessed for eligibility criteria. The study enrolled 87 participants in the heterologous group and 77 participants in the homologous group. Four participants were excluded due to positive serostatus at baseline, one participant in the homologous group and three participants in the heterologous group. These participants were excluded from the immunogenicity analysis. However, they will be included in the safety analysis. All participants were followed up 1 month after receiving the booster dose. However, one-third of the participants (50 out of 160) 20% were lost to follow-up at 4 months.
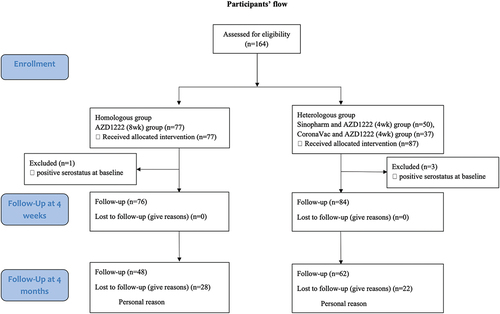
Table 1. Demographic and clinical characteristic data at baseline of all participants in each group.
The study’s primary endpoint was the concentration of anti-RBD antibodies, and the results showed that the homologous group had significantly higher concentrations at both 4 weeks and 4 months after receiving the booster dose. The geometric mean concentration of anti-RBD antibodies at 4 weeks was 452.41 BAU/mL (95% CI, 351.23–582.73) in the heterologous group and 1137.68 BAU/mL (957.30–1352.04) in the homologous group, with a GMR of 0.42 (0.30–0.58). The subgroup analysis showed that the immune response was higher in the CoronaVac/AZD1222 group compared to the BBIBP/AZD1222 group (616.69 vs. 358.61 BAU/mL). The result at 4 months was similar, with the geometric mean concentration of anti-RBD antibodies being 316.62 BAU/mL (258.28–388.13) in the heterologous group and 479.77 BAU/mL (385.60–596.95) in the homologous group, with a GMR of 0.67 (0.50–0.91) ( and ). These results did not meet the non-inferiority criterion, indicating that the homologous booster was superior to the heterologous booster in terms of the anti-RBD antibody response.
Figure 2. Anti-RBD concentration during study period. AZ/AZ: homologous AZD1222 group. IV/AZ: heterologous group. RBD: receptor binding domain. BAU: binding antibody unit. IV: inactivated vaccine. AZ: AZD1222 vaccine (adenoviral vector vaccine). D0: day0 (for prime dose of vaccine). M1: 1 month after booster dose. M4: 4 months after booster dose. *: p-value< .05. ****: p-value < .0001.
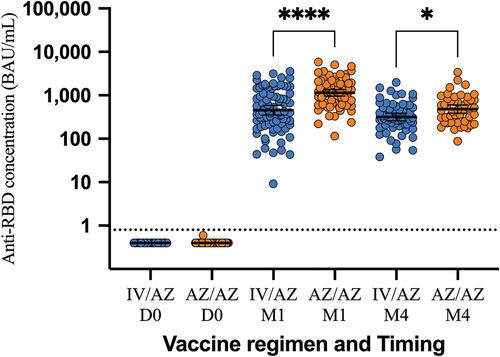
Table 2. The immune response follows both a heterologous and a homologous prime-boost vaccination.
Interestingly, for the surrogate neutralization test, the results showed that the immune response in the heterologous group was not inferior to the homologous group at 4 weeks after receiving the booster dose. The percentage of inhibition was 83.88 (79.72–88.03) in the heterologous group and 79.88 (75.50–84.25) in the homologous group, with a mean difference of 4.60 (−1.67–10.88) ( and ). This result met the non-inferiority criterion. Similar to the binding antibody test for anti-RBD antibodies, the subgroup analysis showed that the percentage of inhibition was higher in the CoronaVac/AZD1222 group compared to the BBIBP/AZD1222 group (87.28% IH vs. 81.32% IH). Interestingly, this response was superior to that of the homologous group. The result at 4 months showed a similar trend with the 4 weeks, although it was not met the non-inferiority criteria.
Figure 3. Surrogate neutralization test during study period. AZ/AZ: homologous AZD1222 group. IV/AZ: heterologous group. sNT: surrogate neutralization test. IV: inactivated vaccine. AZ: AZD1222 vaccine (adenoviral vector vaccine). M1: 1 month after booster dose. M4: 4 months after booster dose. ns: not significant.
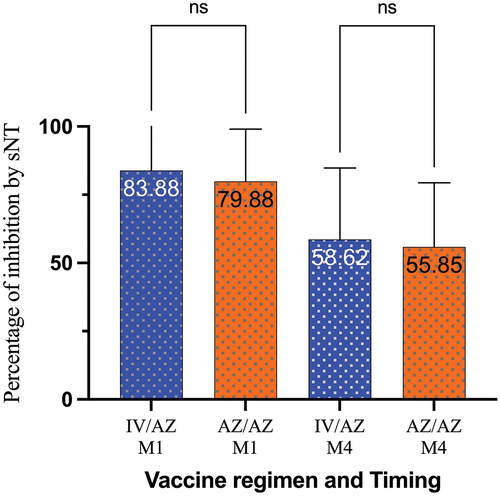
The rate of decline of anti-RBD antibodies in the heterologous group seems to be slower than in the homologous group. At 4 months after the booster vaccination, the concentration of anti-RBD antibodies was about 70% (316.62/452.41 BAU/mL) compared to 42% (479.77/1137.68 BAU/mL) in the homologous group. However, this effect was not observed in the surrogate neutralization test.
The cell-mediated immune response also showed that the heterologous approach was non-inferior to the homologous approach. The geometric mean of interferon-gamma at 4 weeks after receiving the booster dose was 1072.53 mIU/mL (799.29–1439.18) in the heterologous group and 867.67 mIU/mL (671.94–1120.40) in the homologous group, with a GMR of 1.24 (0.82–1.85). The subgroup analysis also supports this response, although the CoronaVac/AZD1222 group had a slightly higher response compared to the BBIBP/AZD1222 group (1133.70 vs. 996.07 mIU/mL) ( and ).
Figure 4. Interferon-gamma level 1 month after booster dose. AZ/AZ: homologous AZD1222 group. IV/AZ: heterologous group. IV: inactivated vaccine. AZ: AZD1222 vaccine (adenoviral vector vaccine). ns: not significant.
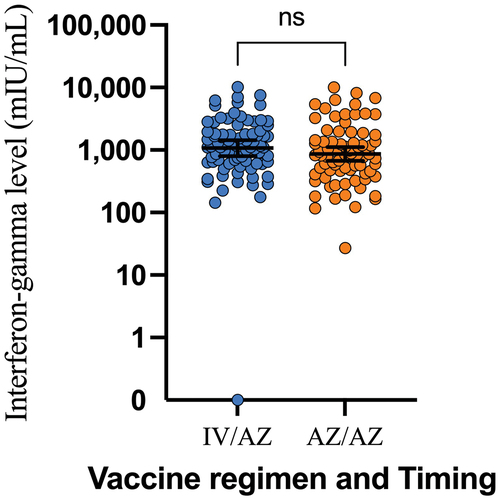
The reactogenicity observed after the prime vaccination with the inactivated vaccine was lower than that of the AZD1222 vaccine (4.6% vs. 22.1%), and most participants recovered within 7 days. Both systemic and local reactogenicity were low after the prime vaccination with the inactivated vaccine. On the other hand, the most common systemic reactogenicities on day-1 after the prime vaccination with AZD1222 were fever (20.8%), headache (18.2%), and drowsiness (18.2%), with the local reactogenicity being about 10.4%.
Interestingly, the reactogenicity observed after the booster dose was higher in the heterologous group than in the homologous group (48.3% vs. 22.1%). The most common systemic reactogenicities after the booster dose in the heterologous group were fever (46.0%), headache (46.0%), and myalgia (44.8%), with the local reactogenicity being about 25.3%. Conversely, the most common systemic reactogenicities in the homologous group after the booster dose were myalgia (20.8%), headache (18.2%), and fatigue (18.2%), with the local reactogenicity being 16.0%. No life-threatening reactogenicity was reported in this study ().
Discussion
Based on the study results, the use of a homologous prime-boost of AZD1222 vaccine results in a higher anti-RBD antibody response compared to a heterologous prime-boost of inactivated and AZD1222 vaccine. However, the surrogate neutralization test showed that the immune response in the heterologous group was not inferior to the homologous group, and the cell-mediated immune response was non-inferior in the heterologous group compared to the homologous group. The humoral immune response in the CoronaVac/AZD1222 group seems to be more robust compared to the BBIBP/AZD1222 group. These findings suggest that a heterologous approach could be considered, particularly in cases where the use of an adenoviral vector vaccine may be limited.
Regarding reactogenicity, the study found that the inactivated vaccine had lower reactogenicity after the prime vaccination than the AZD1222 vaccine. However, the reactogenicity after the booster dose was higher in the heterologous group compared to the homologous group. Nonetheless, no life-threatening reactogenicity was reported in the study.
The authors decided to use an 8-week interval between prime and boost vaccinations in the homologous AZD1222 group instead of a 4-week interval due to a more robust immune response. Voysey M. et al. found that longer prime-boost intervals (>12 weeks) after vaccination with the AZD1222 vaccine result in higher vaccine efficacy compared to shorter prime-boost intervals (< 6 weeks).Citation16
It is possible that the discordance of the results between the binding antibody test and surrogate neutralization test is due to the different functions of the antibodies produced by different vaccines. Binding antibodies may bind to an RBD, but not all the antibodies block its entry into cells.Citation17 The binding antibody test measures the total level of antibodies that recognize an RBD, whereas the surrogate neutralization test measures the ability of the antibodies to block viral entry into cells.Citation14,Citation15 Furthermore, it should be noted that while multifunctional antibodies were observed after the initial dose of the AZD1222 vaccine, their levels were significantly enhanced following the second dose. These multifunctional antibodies comprise anti-spike neutralizing antibodies and Fc-mediated functional antibodies, including antibody-dependent neutrophil/monocyte phagocytosis, complement activation, and natural killer cell activation.Citation18 Conversely, the inactivated COVID-19 vaccine was found to induce relatively low levels of Fc-mediated functional antibodies compared to other vaccine platforms.Citation19 The significance of non-neutralizing antibodies cannot be overstated, particularly in the face of the variant of concern pandemic.Citation20,Citation21 However, due to the study by Lee W.S. et al. showed that Fc-mediated functional antibodies had a longer half-life compared to neutralizing antibodies,Citation22 this explanation cannot account for the result that the rate of decline of anti-RBD antibodies in the homologous group is more rapid than in the heterologous group. Further investigation is necessary to better comprehend the relationship between various antibody types and their efficacy in providing protection against infection in the context of homologous and heterologous vaccination.
Our study results for binding antibody testing differ from those of Wanlapakorn N et al., who found that the level of total RBD antibody was similar between participants receiving heterologous inactivated vaccine and AZD1222 vaccine. They also found that anti-RBD IgG was higher in the heterologous group compared to the homologous group.Citation10 However, the participants in the homologous group of Wanlapakorn N et al. were older, with a mean age of 47.6 (19.0–85.0) compared to 41.1 (18.0–64.0) in the heterologous group. As we know, the immune response of older individuals is generally lower after vaccination.Citation23 This factor may explain the differences between our study and that of Wanlapakorn N et al.
In terms of neutralization testing, our study results are consistent with previous research which has shown that heterologous prime-boost was non-inferior to the homologous prime-boost. Specifically, it is generally known that the mRNA vaccine generates a stronger neutralizing antibody response compared to the adenoviral vector vaccine.Citation24 However, the Com-COV study demonstrated that individuals who received an initial dose of the AZD1222 vaccine followed by the BNT162b2 vaccine with a 28-day interval were able to generate neutralizing antibodies comparable to those produced through a homologous prime-boost with the BNT162b2 vaccine. The NT50 of pseudovirus neutralizing antibody was 515 (430–617) in the heterologous group, compared to 574 (475–694) in the homologous group, with a geometric mean ratio of 0.9.Citation9 In addition, the neutralizing antibody correlates well with the vaccine efficacy.Citation24
When considering the cell-mediated immune response, studies have suggested that the adenoviral vector vaccine may be more robust compared to other vaccine types.Citation9,Citation16 The cell-mediated immune response is crucial in preventing COVID-19 patients from progressing to severe disease,Citation25 and therefore, this aspect of the immune response is of utmost importance. The cell-mediated immune response was robustly stimulated after the initial dose of the AZD1222 vaccine, peaking at day 14 and then declining by day 28. After the second dose with a 28-day interval, the cell-mediated immune response slightly increased, although the level was lower than that observed at day 14 after the initial dose. However, the cell-mediated immune response is more robust if the initial vaccination is done with the AZD1222 vaccine and is followed by a boost with the BNT162b2 vaccine.Citation9 This effect may be due to the vector antibody of the initial vaccine.Citation26 This may explain the result of our study, which showed non-inferiority of the cell-mediated immune response after initial vaccination with the inactivated vaccine and boost with AZD1222 vaccine compared with the homologous prime-boost with AZD1222 vaccine. Although the inactivated COVID-19 vaccine was found to stimulate the cell-mediated immune response less than other vaccine platforms,Citation17 a booster with a potent vaccine such as AZD1222 in participants receiving initial vaccination with the inactivated vaccine can stimulate a robust cell-mediated immune response compared to the homologous prime-boost with AZD1222, which may be affected by the initial vector antibody.
A nationwide case-control study in Thailand during the delta variant pandemic found that heterologous prime-boost vaccination with inactivated vaccine and AZD1222 vaccine had comparable effectiveness to homologous prime-boost with AZD1222 vaccine. The study included 1,460,458 individuals from September to December 2021. Between day 15–29 after the booster dose, vaccine effectiveness was 57.8% (56.3–59.2) for the heterologous group, whereas it was 61.4% (59.6–63.2) for the homologous group. In addition, the results were comparable between the two groups from day 30–89 after the booster dose.Citation27
One of the primary strengths of this study is its comprehensive approach to evaluating the immunogenicity of different vaccination regimens through multiple endpoints, including anti-RBD antibody levels, sNT titers, and interferon-gamma releasing assays. This approach provides a more complete understanding of the immune response to different vaccination strategies, enabling a more informed assessment of their effectiveness.
However, the study’s main limitation is that it was not a randomized controlled trial. Participants were allowed to choose their own regimen due to vaccine hesitation issues during the study period, resulting in baseline differences that were adjusted using regression modeling. Although the surrogate virus neutralization test correlates well with live virus-based or pseudovirus-based assays in determining neutralizing activity, it is not the gold standard. Additionally, the study was conducted in a single center in Thailand, which may limit the generalizability of the findings to other populations and settings. Furthermore, the study only evaluated short-term immunogenicity and safety of the vaccine regimens, and long-term follow-up data are needed to determine the durability of the immune response and the long-term safety profile of the vaccine regimens. There was no laboratory evaluation of SARS-CoV-2 infection during the study period. Although we evaluated the participants’ history of COVID-19, some may have had asymptomatic infections which could interfere with the result evaluation. Finally, the study did not assess the effectiveness of the vaccine regimens in preventing COVID-19 infection or disease.
Conclusion
In conclusion, our study provides evidence that a heterologous prime-boost vaccination strategy using inactivated COVID-19 and AZD1222 vaccines is safe and elicits a non-inferior immune response in terms of neutralizing antibody and cell-mediated immune response compared to a homologous vaccination regimen with AZD1222. These findings are important, especially in settings where vaccine supply is limited or where vaccine distribution is challenging. However, further randomized controlled trials with a larger sample size are needed to confirm the long-term efficacy and safety of a heterologous prime-boost vaccination strategy.
Acknowledgments
We thank the clinical research management unit for managing this project. We also thank the central laboratory of Chulabhorn Hospital for the laboratory testing and gratefully acknowledge funding from Chulabhorn Royal Academy and National Vaccine Institute, Thailand.
Disclosure statement
No potential conflict of interest was reported by the author(s).
Additional information
Funding
References
- World Health Organization. Status of COVID-19 vaccines within WHO EUL/PQ evaluation process 2023. [accessed 2023 Feb 14]. https://www.who.int/teams/regulation-prequalification/eul/covid-19.
- Polack FP, Thomas SJ, Kitchin N, Absalon J, Gurtman A, Lockhart S, Perez JL, Pérez Marc G, Moreira ED, Zerbini C, et al. Safety and efficacy of the BNT162b2 mRNA Covid-19 vaccine. N Engl J Med. 2020;383(27):1–10. doi:10.1056/NEJMoa2034577.
- Baden LR, El Sahly HM, Essink B, Kotloff K, Frey S, Novak R, Diemert D, Spector SA, Rouphael N, Creech CB, et al. Efficacy and safety of the mRNA-1273 SARS-CoV-2 vaccine. N Engl J Med. 2021;384(5):403–16. doi:10.1056/NEJMoa2035389.
- Falsey AR, Sobieszczyk ME, Hirsch I, Sproule S, Robb ML, Corey L, Neuzil KM, Hahn W, Hunt J, Mulligan MJ, et al. Phase 3 safety and efficacy of AZD1222 (ChAdox1 nCoV-19) Covid-19 vaccine. N Engl J Med. 2021;385(25):2348–60. doi:10.1056/NEJMoa2105290.
- Tanriover MD, Doganay HL, Akova M, Guner HR, Azap A, Akhan S, Köse Ş, Erdinç FŞ, Akalın EH, Tabak ÖF, et al. Efficacy and safety of an inactivated whole-virion SARS-CoV-2 vaccine (CoronaVac): interim results of a double-blind, randomised, placebo-controlled, phase 3 trial in Turkey. Lancet. 2021;398(10296):213–22. doi:10.1016/S0140-6736(21)01429-X.
- Al Kaabi N, Zhang Y, Xia S, Yang Y, Al Qahtani MM, Abdulrazzaq N, Al Nusair M, Hassany M, Jawad JS, Abdalla J, et al. Effect of 2 inactivated SARS-CoV-2 vaccines on symptomatic COVID-19 infection in adults: a randomized clinical trial. JAMA. 2021;326(1):35–45. doi:10.1001/jama.2021.8565.
- World Health Organization. Coronavirus (COVID-19) dashboard 2023. [accessed 2023 Feb 14]. https://covid19.who.int.
- Cohen G, Jungsomsri P, Sangwongwanich J, Tawinprai K, Siripongboonsitti T, Porntharukchareon T, Wittayasak K, Thonwirak N, Soonklang K, Sornsamdang G, et al. Immunogenicity and reactogenicity after heterologous prime-boost vaccination with CoronaVac and ChAdox1 nCov-19 (AZD1222) vaccines. Human Vacc Immunother. 2022;18(5):2052525. doi:10.1080/21645515.2022.2052525.
- Liu X, Shaw RH, Stuart ASV, Greenland M, Aley PK, Andrews NJ, Cameron JC, Charlton S, Clutterbuck EA, Collins AM, et al. Safety and immunogenicity of heterologous versus homologous prime-boost schedules with an adenoviral vectored and mRNA COVID-19 vaccine (Com-COV): a single-blind, randomised, non-inferiority trial. Lancet. 2021;398(10303):856–69. doi:10.1016/S0140-6736(21)01694-9.
- Wanlapakorn N, Suntronwong N, Phowatthanasathian H, Yorsaeng R, Vichaiwattana P, Thongmee T, Auphimai C, Srimuan D, Thatsanatorn T, Assawakosri S, et al. Safety and immunogenicity of heterologous and homologous inactivated and adenoviral-vectored COVID-19 vaccine regimens in healthy adults: a prospective cohort study. Human Vacc Immunother. 2022;18(1):2029111. doi:10.1080/21645515.2022.2029111.
- Tawinprai K, Siripongboonsitti T, Porntharukchareon T, Wittayasak K, Thonwirak N, Soonklang K, Sornsamdang G, Auewarakul C, Mahanonda N. Reactogenicity, immunogenicity, and humoral immune response dynamics after the third dose of heterologous COVID-19 vaccines in participants fully vaccinated with inactivated vaccine. Expert Rev Vaccines. 2022 Dec;21(12):1873–81. doi:10.1080/14760584.2022.2099380.
- Frenck RW Jr., Klein NP, Kitchin N, Gurtman A, Absalon J, Lockhart S, Perez JL, Walter EB, Senders S, Bailey R, et al. Safety, immunogenicity, and efficacy of the BNT162b2 Covid-19 vaccine in adolescents. N Engl J Med. 2021;385(3):239–50. doi:10.1056/NEJMoa2107456.
- Infantino M, Pieri M, Nuccetelli M, Grossi V, Lari B, Tomassetti F, Calugi G, Pancani S, Benucci M, Casprini P, et al. The WHO international standard for COVID-19 serological tests: towards harmonization of anti-spike assays. Int Immunopharmacol. 2021 Nov;100:108095. doi:10.1016/j.intimp.2021.108095.
- Rus KR, Korva M, Knap N, Avsic Zupanc T, Poljak M. Performance of the rapid high-throughput automated electrochemiluminescence immunoassay targeting total antibodies to the SARS-CoV-2 spike protein receptor binding domain in comparison to the neutralization assay. J Clin Virol. 2021;139:104820. doi:10.1016/j.jcv.2021.104820.
- Tan CW, Chia WN, Qin X, Liu P, Chen MI, Tiu C, Hu Z, Chen VCW, Young BE, Sia WR, et al. A SARS-CoV-2 surrogate virus neutralization test based on antibody-mediated blockage of ACE2–spike protein–protein interaction. Nat Biotechnol. 2020;38(9):1073–8. doi:10.1038/s41587-020-0631-z.
- Voysey M, Costa Clemens SA, Madhi SA, Weckx LY, Folegatti PM, Aley PK, Angus B, Baillie VL, Barnabas SL, Bhorat QE, Oxford COVID Vaccine Trial Group, et al. Single-dose administration and the influence of the timing of the booster dose on immunogenicity and efficacy of ChAdOx1 nCoV-19 (AZD1222) vaccine: a pooled analysis of four randomised trials. Lancet. 2021 Mar 6;397(10277):881–91. doi:10.1016/S0140-6736(21)00432-3. Epub 2021 Feb 19. Erratum in: Lancet. 2021 Mar 6;397(10277):880. PMID: 33617777; PMCID: PMC7894131.
- Sadarangani M, Marchant A, Kollmann TR. Immunological mechanisms of vaccine-induced protection against COVID-19 in humans. Nat Rev Immunol. 2021;21(8):475–84. doi:10.1038/s41577-021-00578-z.
- Barrett JR, Belij-Rammerstorfer S, Dold C, Ewer KJ, Folegatti PM, Gilbride C, Halkerston R, Hill J, Jenkin D, Stockdale L, et al. Phase 1/2 trial of SARS-CoV-2 vaccine ChAdOx1 nCoV-19 with a booster dose induces multifunctional antibody responses. Nat Med. 2021;27(2):279–88. doi:10.1038/s41591-020-01179-4.
- Bartsch YC, Tong X, Kang J, Avendano MJ, Serrano EF, Garcia-Salum T, Pardo-Roa C, Riquelme A, Cai Y, Renzi I, et al. Omicron variant spike-specific antibody binding and Fc activity are preserved in recipients of mRNA or inactivated COVID-19 vaccines. Sci Transl Med. 2022;14(642):eabn9243. doi:10.1126/scitranslmed.abn9243.
- Kaplonek P, Cizmeci D, Fischinger S, Collier AR, Suscovich T, Linde C, Broge T, Mann C, Amanat F, Dayal D, et al. mRNA-1273 and BNT162b2 COVID-19 vaccines elicit antibodies with differences in Fc-mediated effector functions. Sci Transl Med. 2022;14(645):eabm2311. doi:10.1126/scitranslmed.abm2311.
- Kaplonek P, Fischinger S, Cizmeci D, Bartsch YC, Kang J, Burke JS, Shin SA, Dayal D, Martin P, Mann C, et al. mRNA-1273 vaccine-induced antibodies maintain Fc effector functions across SARS-CoV-2 variants of concern. Immunity. 2022;55(2):355–65 e4. doi:10.1016/j.immuni.2022.01.001.
- Lee WS, Selva KJ, Davis SK, Wines BD, Reynaldi A, Esterbauer R, Kelly HG, Haycroft ER, Tan H-X, Juno JA, et al. Decay of Fc-dependent antibody functions after mild to moderate COVID-19. Cell Rep Med. 2021 Jun 15;2(6):100296. doi:10.1016/j.xcrm.2021.100296.
- Tawinprai K, Siripongboonsitti T, Porntharukchareon T, Dechates B, Monprach H, Sornsamdang G, Wittayasak K, Soonklang K, Mahanonda N. Persistence of immunogenicity, contributing factors of an immune response, and reactogenicities after a single dose of the ChAdOx1 (AZD1222) COVID-19 vaccine in the Thai population. Human Vacc Immunother. 2022;18(1):2035573. doi:10.1080/21645515.2022.2035573.
- Khoury DS, Cromer D, Reynaldi A, Schlub TE, Wheatley AK, Juno JA, Subbarao K, Kent SJ, Triccas JA, Davenport MP. Neutralizing antibody levels are highly predictive of immune protection from symptomatic SARS-CoV-2 infection. Nat Med. 2021;27(7):1205–11. doi:10.1038/s41591-021-01377-8.
- Rydyznski Moderbacher C, Ramirez SI, Dan JM, Grifoni A, Hastie KM, Weiskopf D, Belanger S, Abbott RK, Kim C, Choi J, et al. Antigen-specific adaptive immunity to SARS-CoV-2 in acute COVID-19 and associations with age and disease severity. Cell. 2020;183(4):996–1012 e19. doi:10.1016/j.cell.2020.09.038.
- Logunov DY, Dolzhikova IV, Shcheblyakov DV, Tukhvatulin AI, Zubkova OV, Dzharullaeva AS, Kovyrshina AV, Lubenets NL, Grousova DM, Erokhova AS, et al. Safety and efficacy of an rAd26 and rAd5 vector-based heterologous prime-boost COVID-19 vaccine: an interim analysis of a randomised controlled phase 3 trial in Russia. Lancet. 2021;397(10275):671–81. doi:10.1016/S0140-6736(21)00234-8.
- Nittayasoot N, Suphanchaimat R, Thammawijaya P, Jiraphongsa C, Siraprapasiri T, Ploddi K, Pittayawonganon C, Mahasirimongkol S, Tharmaphornpilas P. Real-world effectiveness of COVID-19 vaccines against severe outcomes during the period of omicron predominance in Thailand: a test-negative nationwide case–control study. Vaccines (Basel). 2022;10(12):2123. doi:10.3390/vaccines10122123.