ABSTRACT
This study aims to summarize and visually analyze the current research status in pancreatic cancer immunotherapy during the past two decades by bibliometrics and explore the current research hotspots and future development directions. The literature related to pancreatic cancer immunotherapy from 2002 to 2021 was downloaded from the core database of the Web of Science. VOSviewer and CiteSpace software were used to visualize the included literature. A total of 2528 articles were included. In the past two decades, publications in the pancreatic cancer immunotherapy field have increased almost annually. As the country with the largest publications, the United States has various research institutions dedicated to pancreatic cancer immunotherapy. Jaffee EM and Zheng L from Johns Hopkins University and Vonderheide RH from the University of Pennsylvania have published the most articles in this field. The current research hotspots of pancreatic cancer immunotherapy include the tumor microenvironment, immune cells, immune checkpoint blockade, and combination therapy. The study of novel immunotherapies and combination therapy may become the primary focus of future research on pancreatic cancer immunotherapy. More prospective clinical studies with high evidence levels should be conducted.
Introduction
Pancreatic cancer is among the leading causes of death in cancer patients worldwide.Citation1 From 1990 to 2017, global mortality due to pancreatic cancer rose from approximately 196,000 to 441,000.Citation2 The pancreatic cancer incidence is increasing by nearly 0.5 to 1.0% annually, and the incidence of pancreatic cancer may continue to rise.Citation3 Most pancreatic cancer patients are in the advanced stages when diagnosed and lose the opportunity for surgical treatment because of distant metastasis and invasion of essential vessels.
Immunotherapy is a hotspot of the new generation of cancer therapyCitation4. Immunotherapy based on immune checkpoint blockade has advanced during the past two decades.Citation5 Immune checkpoint inhibitors (ICIs) have further optimized the treatment of various types of cancer. Immune checkpoints are immunological cell-expressed receptors that can dynamically regulate immune homeostasis and are particularly important for T-cell function.Citation6 Programmed cell death 1 (PD-1)programmed cell death 1 ligand 1 (PD-L1), and cytotoxic T lymphocyte-associated protein 4 (CTLA-4) are the most commonly studied immune checkpoints in cancer research.Citation7
Research on immunotherapy related to pancreatic cancer is essential to prolong the patient survival. This study applies bibliometric analysis to analyze the characteristics of published papers in pancreatic cancer immunotherapy from 2002 to 2021, aiming to provide new insights into pancreatic cancer research.
Method
Literature sources
Web of Science is widely recognized as the most comprehensive and reliable database for bibliometric analysis, covers nearly all high-quality and influential journals, and provides a comprehensive set of citation data. To improve data representativeness and accessibility, all of the data were extracted from the Web of Science Core Collection (https://www.webofscience.com/wos/woscc/). The retrieval formula is as follows (from January 1, 2002, to December 31, 2021):
#1 TS= Pancreatic Neoplasms OR Pancreatic Neoplasm OR Pancreas Neoplasms OR Pancreas Neoplasm OR Cancer of Pancreas OR Cancer of the Pancreas OR Pancreatic Cancers OR Pancreatic Cancer OR Pancreas Cancers OR Pancreas Cancer OR Pancreatic ductal adenocarcinoma OR Pancreatic adenocarcinoma OR PDAC.
#2 TS= Immunotherap*.
#3 #1 AND #2.
Study selection
Inclusion criteria: publications on immunotherapy for pancreatic cancer were published between January 1, 2002, and December 31, 2021.
Exclusion criteria: (1) non-English literature; (2) conference abstracts, editorials, letters, book chapters, and other types of literature. The screening flow chart of the inclusion and exclusion criteria is shown in .
Moreover, the data of online literature analysis were downloaded, including the volume of literature published annually, country/region of publication, institution of publication, and citation times.
Data analysis and visualization
VOSviewer (Version 1.6.18), CiteSpace software (Version 6.1 R3), and the Charticulator online platform were used for visual analysis. VOSviewer is a software developed for building and viewing bibliometric maps. VOSviewer prioritizes the graphical display of bibliometric mapping.Citation8 CiteSpace is a Java application that visually maps rows of highly cited literature and research topics through visualization methods to detect and visualize trends and patterns in the scientific literature.Citation9 Charticulator is an interactive authoring tool that creates customized and reusable chart layouts.Citation10 The web address of Charticulator is https://charticulator.com.
VOSviewer software was used to extract and cluster the countries/regions, institutions, authors, and keywords included in all studies; the main visualization methods were Network, Overlay, and Density. The CiteSpace software set the time slice value as one year and used the Burst Detection algorithm in the software to detect the keywords’ emergence.
Results
The trend of publication outputs
A total of 2528 English studies on immunotherapy for pancreatic cancer were obtained, and the annual publications are shown in . The results showed that from 2002 to 2021, the articles published on pancreatic cancer immunotherapy increased annually.
The top three journals with the most published articles were “Cancers,” “Clinical Cancer Research,” and “Frontiers in Immunology,” with 241 articles (9.534%) in total. The basic information of the top 10 journals with the number of published articles is summarized in .
Table 1. The top 10 journals related to pancreatic cancer immunotherapy from 2002 to 2021.
Countries and institutions
From 2002 to 2021, 74 countries/regions published studies related to pancreatic cancer immunotherapy, among which the United States had the largest number of publications (1117 articles, 44.19%), followed by China (584 articles, 23.10%) and Germany (247 articles, 9.77%). The top three institutions were Johns Hopkins University (123 articles), the University of Texas (107 articles), and the University of California (86 articles). The top 10 institutions are mainly located in the United States and Germany. The top 10 countries and institutions are shown in .
Figure 3. Top 10 countries/regions and institutions related to pancreatic cancer immunotherapy from 2002 to 2021.
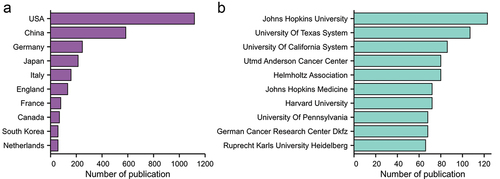
Clustering analysis was performed using VOSviewer software to obtain visualization results, and country/region cooperation was performed on the Charticulator online platform ().
Author
Density visualization with VOSviewer software was used to obtain the density analysis diagram of the researchers from the published literature (). Jaffee EM and Zheng L from Johns Hopkins University and Vonderheide RH from the University of Pennsylvania are leading research contributors worldwide in pancreatic cancer immunotherapy.
Research hotspots and keywords
As of August 17, 2022, there were 100 highly cited papers among the 2,528 studies. The title, number of citations, number of publication years, and journal information of the top 10 papers with citation frequency are shown in . The results showed that the highly cited literature in the research field was mainly related to clinical trials, immune checkpoint inhibitors, immune cells, and the tumor microenvironment.
Table 2. The top 10 cited articles related to pancreatic cancer immunotherapy from 2002 to 2021.
A total of 8107 keywords were extracted and statistically analyzed, and 228 keywords appeared at least 20 times. The top 10 most frequently used keywords were: “immunotherapy,” “pancreatic cancer,” “cancer,” “pancreatic cancer,” “expression,” “gemcitabine,” “dendritic cells,” “t-cells,” “chemotherapy,” and “therapy.” Keywords with higher frequency (≥20) were selected for cluster analysis, and three clusters were obtained. The keyword co-occurrence network diagram is shown in . The results showed that in the early stage of pancreatic cancer immunotherapy, dendritic cells, colony-stimulating factors, and in vitro experiments were the main research directions. In recent years, the tumor microenvironment, blockers, and clinical trials have been the main research directions ().
CiteSpace was used for keyword burst analysis (). The results indicated that “tumor microenvironment” was the most prominent and that “colony-stimulating factor” was the longest topic in the pancreatic cancer immunotherapy literature over the past 20 years. The terms “nivolumab,” “epithelial-mesenchymal transition,” “tumor microenvironment,” “CTLA 4 inhibition,” and “open-label” have emerged in recent years. In the future, these keywords may become a research hotspot and disciplinary breakthrough in pancreatic cancer immunotherapy.
Discussion
Immunotherapy is a novel method of cancer treatment, along with surgery, radiotherapy, chemotherapy, and targeted therapy. Immunotherapy has also advanced the thorough diagnosis and treatment of pancreatic cancer in recent years.Citation11,Citation12 In recent decades, studying the interaction between the human immune system and cancer has led to the development of cancer immunotherapy. The research established in tumor immunology is now providing evidence for clinical cancer treatment. In this study, bibliometrics was used to analyze and elaborate on the hotspots of the literature in the field of pancreatic cancer immunotherapy from 2002 to 2021. Bibliometrics is a quantitative analysis technique that employs mathematical and statistical tools to characterize, analyze, and predict the research status and development trend of a certain topic by utilizing the quantitative properties of the literature.Citation13 Bibliometrics is undertaken using extensive data analysis and statistical approaches, which minimizes the subjective bias of traditional literature retrieval and artificial induction and summary to some extent, yielding more objective and reliable results. The main results of this paper show that the number of publications in the field of pancreatic cancer immunotherapy has increased annually in the past 20 years. The United States, being the country with the most publications, has many research facilities devoted to pancreatic cancer immunotherapy, which gives it substantial advantages in the field. Furthermore, through the analysis of the keywords, it can be found that the primary research hotspots in the field of pancreatic cancer immunotherapy have also focused on the tumor microenvironment, immune cells, immune checkpoint inhibitors, and other issues in recent years.
Based on the keyword clustering analysis and the specific contents of some critical studies in recent years, the research hotspots in the field of pancreatic cancer immunotherapy mainly focus on (1) the tumor microenvironment; (2) immunity regulation of pancreatic cancer; and (3) combination therapy of pancreatic cancer. The following analysis is performed with each topic’s internal high-frequency words and highly referenced literature.
Tumor microenvironment
The tumor microenvironment is a complex and constantly changing environment for tumor cells to survive.Citation14 Studies have shown that pancreatic cancer development is closely related to the tumor microenvironment.Citation15 In recent years, in-depth research on tumor microenvironment-targeting cells, molecules, and extracellular matrix has been a hot topic in cancer diagnostics and the development of new therapeutics (). Various components of the tumor microenvironment are vital in regulating tumor cell growth, metastasis, and drug resistance and lead to the formation of an immunosuppressive microenvironment. Tumor microenvironment of pancreatic cancer are immunosuppressed due to the presence of various immunosuppressive cells. Immunosuppressive cells in pancreatic cancer include myeloid-derived suppressor cells (MDSCs), regulatory T cells (Treg), M2-type macrophages, pancreatic stellate cells (PSCs), cancer-associated fibroblasts (CAFs) and gamma/delta T-cells (γδ T cells).
Treg cells are a subset of T lymphocytes that can influence tumor growth by acting directly on cancer cells or inhibiting immune cells.Citation16 Treg cells can also form networks with other immunosuppressive cells (such as MDSC), enhancing their immunosuppressive ability against tumors and their resistance to immunotherapy. IL-2α receptor CD25 is a Treg Cell intervention target.Citation17 In a mouse xenograft model of pancreatic cancer Pan02 cell line, CD25 monoclonal antibody, toll-like receptor 9 (TLR9) agonist CpG and pancreatic cancer cell-specific antigen immune stimulatory complex (ISCOM) vaccine reduced the Tregs infiltration in tumor tissue inhibited tumor growth, induced Th1 immune response and activated immune cells, and finally inhibit tumor progression through CD8+ T cells.Citation18 Furthermore, Soares et al.Citation19 found that in a mouse pancreatic cancer model, the combination of transforming growth factor-β (TGF-β) blocking antibody and granulocyte-macrophage colony-stimulating factor (GM-CSF) gene-modified tumor vaccine GVAX can reduce Treg infiltration in the tumor microenvironment, significantly increase antitumor specific CD8+ T cell infiltration, increase IFN-γ secretion, and prolong the survival time of pancreatic cancer mice.
MDSCs are immature bone marrow cells with immunosuppressive function. MDSCs can produce high levels of nitric oxide (NO) and reactive oxygen species, which inhibit T cell proliferation and function.Citation20,Citation21 MDSCs can also boost the Treg function.Citation22 In pancreatic cancer, migration, and recruitment of MDSCs to the tumor microenvironment are mediated by CD11b/CD18 integrin heterodimer. GB1275 is a CD11b regulator. After treatment with GB1275 in a mouse pancreatic cancer model, it was discovered that reducing the number of MDSCs simultaneously increases the number of activated CD103+ dendritic cells (DCs) and CD8+ T cells. After combined treatment with anti-PD-1 antibody and GB1275 in the mouse Pancreatic ductal adenocarcinoma (PDAC) model, the mice survival in the combined treatment group was significantly prolonged compared with the control group, which is worthy of further clinical study.Citation23
Tumor-associated macrophages (TAMs) include the M1 type, which inhibits tumor growth, and the M2 type, which promotes tumor invasion. TAMs often appear as M2-types in the tumor microenvironment, forming the immunosuppressive microenvironment of PDAC. Increased arginase-1, CD206, and interleukin-10 expression in M2-type macrophages may play a role in tissue healing and angiogenesis.Citation24 M2-type macrophages are essential in pancreatic cancer. In 2020, Lu et al.Citation25 found that pancreatic cancer patients with high IL-20 expression had a poor prognosis. Using KPC and in situ PDAC models, they found that targeting IL-20 could reduce the infiltration of M2-type TAMs in the in situ PDAC model. Combined treatment with IL-20 antagonist 7E and anti-PD-1 antibody can reduce the infiltration of M2 and increase the infiltration of CD8+ T cells, weaken the immune suppression of the tumor microenvironment, inhibit tumor growth, and significantly prolong the survival time of mice. Kaneda et al.Citation26 found that PI3Kγ inhibition could inhibit the polarization of M2-type tumor-associated macrophages, restore the antitumor function of T cells, and improve the responsiveness of the pancreatic cancer mouse model to chemotherapy.
Many CAFs are activated in the pancreatic cancer stroma; when CAFs in the tumor microenvironment are activated, the extracellular matrix can be generated to form a barrier that prevents antitumor drugs and immune cells.Citation27 Fibroblast activation protein (FAP), an activation marker of CAFs, is overexpressed in pancreatic tumor TMEs and is associated with poor prognosis in patients. In a mouse pancreatic cancer model, the combination of FAP inhibitor and anti-PD-L1 antibody reduced the inhibitory factor CXCL12 production, thereby slowing PDAC progression and prolonging the survival of tumor-bearing mice.Citation28 Focal adhesion kinase (FAK) is a nonreceptor tyrosine-protein kinase. Jiang et al.Citation29found that FAK is overexpressed in human pancreatic cancer and vital in the immunosuppressant microenvironment. The FAK inhibitor VS-4718, gemcitabine, and anti-PD-1 antibody were combined in the KPC mouse model. The results showed that FAK inhibitors inhibited pancreatic interstitial fibrosis, promoted pancreatic cancer response to immunotherapy, significantly inhibited tumor growth, and prolonged the survival of tumor-bearing mice. Symeonides et al.Citation30 further combined the FAK inhibitor, gemcitabine, and anti-PD-1 antibody in the PDAC mouse model and showed a robust antitumor effect, significantly prolonging the survival of mice, and found that inhibition of FAK can reduce the extracellular matrix, thereby improving the status of pancreatic cancer TME.
As essential fibroblast-like cells in the tumor microenvironment of pancreatic cancer, PSCs are capable of secreting galectin-1 and IL-6 and promoting T-cell apoptosis.Citation31 IL-6 is mainly derived from pancreatic stellate cells. In 2020, Kesh et al.Citation32 found that in a mouse model of pancreatic cancer, an IL-6 inhibitor could inhibit pancreatic tumor progression, reduce the proportion of activated M2 macrophage in the pancreatic tumor microenvironment and increase CD8+ infiltration in the tumor microenvironment. It can also lower lactate excretion and modulate pancreatic TME, rendering pancreatic tumors susceptible to anti-PD1 therapy.
The formation of the immunosuppressive microenvironment of pancreatic cancer also seems to be related to the involvement of γδ T cells, which belong to the unconventional innate lymphocyte group and express T cell receptors (TCR) with γ and δ chains on their surface.Citation33 In normal pancreatic tissue or mice, the number of γδ T cells can be almost negligible, but PDAC patients compared with healthy patients, peripheral blood and PDAC tumor itself γδ T cells percentage increased.Citation34 Studies have shown that in pancreatic tumor stroma, γδ T cells are located near the PSC. These γδ T cells are able to induce IL-6 production from the PSC of PDAC, thereby regulating immunosuppressive tumor microenvironment.Citation35 Daley et al.Citation36 observed that pancreatic tumor-infiltrating γδ T cells can promote tumorigenesis by inhibiting αβ T cell infiltration, activation, and Th1 polarization. Therapeutic strategies based on the tumor microenvironment have become a promising approach for cancer treatment, mainly including immunotherapy, anti-angiogenesis, and extracellular matrix therapy.Citation37
Immune cells and tumor immunity
The function of macrophages and T cells in pancreatic cancer and cancer vaccines provided new insight into immune therapy.Citation38–40 Macrophages, T cells, dendritic cells, and other immune cells can directly affect the invasion, metastasis, and angiogenesis of pancreatic cancer and are also associated with antitumor immune activity and tumor immune escape.Citation41 First, cancer vaccines can effectively initiate the body’s antitumor immune response. Pancreatic cancer vaccines such as GVAX and survivin have been proven to stimulate antitumor immune activation.Citation39,Citation42 CD40 agonists can also improve antigen-presenting cells activity and boost immune responses in the tumor microenvironment of pancreatic cancer.Citation43 Studies have shown that the combination of CD-40 agonists is necessary to improve the survival rate of patients with PDAC. CD40 monotherapy improves CD8+T cell infiltration and depletes CD8+ T cells in the tumor microenvironment.Citation44
T-cell-based tumor treatment strategies mainly include chimeric antigen receptor T-cell (CAR-T) and T-cell receptor-engineered T-cell therapy, which has achieved good results in patients with hematological malignancies.Citation45 Currently, CAR-T therapy targets for pancreatic cancer include mesoderm, carcinoembryonic antigen, tyrosine kinase growth factor receptor, and fibroblast activating protein. However, most CAR-T therapy clinical trials in pancreatic cancer are still in the early stage.Citation46
Antagonizing immunosuppressive cells or factors is also one of the methods of immunotherapy. Both multinucleated MDSCs and M2 macrophages can lead to the immune escape of tumor cells.Citation47 The CCL2-CCR2 signaling axis is intimately associated with macrophage recruitment, and blockage of this axis can decrease M2-type macrophage activity in the tumor microenvironment. Specific inhibitors of the CCL2-CCR2 signaling axis, CCX872, and PF-04136309, have been used in clinical trials of pancreatic cancer patients.Citation48,Citation49 In preclinical models of pancreatic cancer, blocking colony-stimulating factor-1 and its receptor pathway can also polarize macrophages to the antitumor M1 phenotype.Citation50
Combination therapy and comprehensive diagnosis and treatment of pancreatic cancer
The combined treatment and comprehensive diagnosis and treatment of pancreatic cancer are also one of the current and future research directions. There have been several large-scale clinical studies on pancreatic cancer immunotherapy in recent years (NCT03404960, NCT03214250). Since the in-depth study of immune checkpoints on the surface of T cells, immune checkpoint blocking, mainly PD-1 and CTLA4, has become a new therapeutic strategy for many cancers.Citation6,Citation51 However, the efficacy of immune checkpoint inhibitors in pancreatic cancer is suboptimal, primarily because the low mutation load of pancreatic cancer leads to weak immunogenicity and cannot induce T-cell activation, and the tumor microenvironment of pancreatic cancer is immunosuppressive, further inhibiting T-cell function and number.Citation47
In recent years, clinical research on pancreatic cancer immunotherapy has gradually focused on combining traditional or targeted therapy with immune checkpoint inhibitors, such as targeting CXCL12 and PD-L1.Citation28 Various models of immune therapy are one of the future research directions.
More drug combinations can directly inhibit cancer cells and cancer cells sensitive to immunotherapy, activate the endogenous immune response, and drive antitumor immune cell infiltration and a series of effects, mainly immune checkpoint inhibitors combined with chemotherapy drugs, or remove negative regulatory factors.Citation5 In a phase 2 clinical study in seven centers in the United States, pancreatic cancer patients received CD40 agonists, PD-1 inhibitors, and traditional chemotherapy to achieve various effects of inhibiting cancer cell growth, promoting T-cell activation, and preventing T-cell depletion.Citation52 The objective response rate, disease control rate, and median response time of 22 patients treated with a chemokine CXCR4 antagonist in combination with a PD-L1 inhibitor and chemotherapy were 32%, 77%, and 7.8 months, respectively, according to a COMBAT/KEYKEY-202 trial.Citation53 Moreover, combining a PD-1 inhibitor and CTLA-4 inhibitor can prevent T-cell depletion in peripheral tissues and inhibit the immunosuppressive function of regulatory T cells, synergistically activating antitumor immunity and improving the immune response rate of patients.Citation54 Therefore, this combination therapy, including immunotherapy, will become a new treatment strategy for pancreatic cancer patients in the future. At present, the common combined immunotherapy includes dual immunotherapy, immunotherapy combined with chemotherapy, etc.Citation55 Previous studies have shown that the use of PD-1, PD-L1 or CTLA 4 checkpoints inhibitors for the treatment of patients with PDAC did not produce significant clinical improvement, Yamamoto et al.Citation56 found that chloroquine inhibit autophagy synergy with the double ICIs therapy can enhance antitumor immune effects, but a phase 2 clinical trialCitation57 results showed that the combination of the anti-PD-L1 antibody Durvalumab and the anti-CTLA-4 antibody Tremelimumab did not significantly improve the prognosis of patients with metastatic PDAC, indicating that dual ICIs therapy is not effective in patients with metastatic PDAC. Therefore, some scholars have begunto explore ICIs in combination with nonspecific immune regulators but are less effective. Moreover, ICIs in combination with oncolytic viruses or vaccines are also in progress, and the current study shows therapeutic potential, but further studies are still needed to prove their therapeutic efficacy.Citation55 Immunotherapy combined with chemotherapy has shown great promise in the treatment of patients with PDAC. Chemotherapy can enhance the expression of tumor antigens and enhance the sensitivity of tumors to immunotherapy. The use of immunotherapy combined with traditional chemotherapy regimens may further improve the effectiveness of treatment. In a preclinical study,Citation58 gemcitabine potentiated immune responses to anti-PD-1 antibodies in animal models. Vaccine or virus combined with chemotherapy also affects the course of PDAC patients, but no significant effect has been determined so far, and further exploration is needed. summarizes the current clinical trials of combined immunotherapy with nivolumab, pembrolizumab, and ipilimumab for pancreatic cancer.
Table 3. Summary of ongoing clinical trials of combination immunotherapy for pancreatic cancer.
Limitations
(1) This study acquired literature from 2002 to 2021, and with the continuous updating of the WOS core library, the retrieval results of this study are slightly different from the actual number of entries. (2) We only used the Web of Science Core Collection for systematic literature search, and there is a possibility of missing some relevant literature, which may influence the results of this study. (3) Due to its characteristics, the analysis of the visualization software may overlook crucial details. (4) We used English as the only language for literature searches and therefore may have missed some high-quality non-English literature data.
Conclusion
In conclusion, publications in the pancreatic cancer immunotherapy field have increased annually worldwide in the past 20 years. In the pancreatic cancer immunotherapy field, cancer vaccines and monoclonal antibodies have been constantly optimized, and research on the tumor microenvironment and immune cell function has been conducted gradually. The combination therapy of traditional chemotherapy, targeted therapy, and immune checkpoint blockade is being explored. The latest evidence for cancer immunotherapy will be provided through prospective, multicenter, and high-quality clinical research. Future research should focus on combining immunotherapy and other therapies to identify the latest methods for prolonging the pancreatic cancer patient survival.
Authors’ contributions
JN, WJ, WZ and HZ designed the study. JN and WJ drafted the initial manuscript. JN and XL analyzed the data and performed the statistical analyses. WJ and DF performed the visualization. WZ and HZ provided the language help. All authors reviewed the drafted manuscript for critical content and approved the final version.
Ethics approval and consent to participate
Since the data from this study were obtained from a public database, ethical approval from an institutional review board or ethics committee was not needed.
Acknowledgments
The authors would thank the Web of Science Core Collection (https://www.webofscience.com/wos/woscc) for providing the data and Ningbo freescience Information Technology Co., Ltd. for the expert linguistic services provided.
Disclosure statement
No potential conflict of interest was reported by the author(s).
Data availability statement
Original datasets are available in a publicly accessible repository: The original contributions presented in the study are publicly available. This data can be found here: (https://www.webofscience.com/wos/woscc/).
Additional information
Funding
References
- Klein AP. Pancreatic cancer epidemiology: understanding the role of lifestyle and inherited risk factors. Nat Rev Gastroenterol Hepatol. 2021;18(7):493–12. PMID: 34002083. doi:10.1038/s41575-021-00457-x.
- Chen X, Yi B, Liu Z, Zou H, Zhou J, Zhang Z, Xiong L, Wen Y. Global, regional and national burden of pancreatic cancer, 1990 to 2017: results from the global burden of disease study 2017. Pancreatology. 2020;20(3):462–9. PMID: 32113937. doi: 10.1016/j.pan.2020.02.011.
- Collaborators GBD Pancreatic Cancer. The global, regional, and national burden of pancreatic cancer and its attributable risk factors in 195 countries and territories, 1990–2017: a systematic analysis for the global burden of disease study 2017. Lancet Gastroenterol Hepatol. 2019;4(12):934–47. PMID: 32061329. doi:10.1016/S2468-1253(20)30018-2.
- Morrison AH, Byrne KT, Vonderheide RH. Immunotherapy and prevention of pancreatic cancer. Trends Cancer. 2018;4(6):418–28. PMID: 29860986. doi:10.1016/j.trecan.2018.04.001.
- Hegde PS, Chen DS. Top 10 challenges in cancer immunotherapy. Immunity. 2020;52(1):17–35. PMID: 31940268. doi:10.1016/j.immuni.2019.12.011.
- Drake CG, Lipson EJ, Brahmer JR. Breathing new life into immunotherapy: review of melanoma, lung and kidney cancer. Nat Rev Clin Oncol. 2014;11(1):24–37. PMID: 24247168. doi:10.1038/nrclinonc.2013.208.
- Morad G, Helmink BA, Sharma P, Wargo JA. Hallmarks of response, resistance, and toxicity to immune checkpoint blockade. Cell. 2021;184(21):5309–37. PMID: 34624224. doi: 10.1016/j.cell.2021.09.020.
- Van Eck NJ, Waltman L. Software survey: VOSviewer, a computer program for bibliometric mapping. Scientometrics. 2010;84(2):523–38. PMID: 20585380. doi:10.1007/s11192-009-0146-3.
- Chen C. Searching for intellectual turning points: progressive knowledge domain visualization. Proc Natl Acad Sci U S A. 2004;101(Suppl 1):5303–10. PMID: 14724295. doi:10.1073/pnas.0307513100.
- Ren D, Lee B, Brehmer M. Charticulator: interactive construction of bespoke chart layouts. IEEE Trans Vis Comput Graph. 2019;25(1):789–799. PMID: 30136992. doi:10.1109/TVCG.2018.2865158.
- Korman AJ, Garrett-Thomson SC, Lonberg N. The foundations of immune checkpoint blockade and the ipilimumab approval decennial. Nat Rev Drug Discov. 2022;21(7):509–28. PMID: 34937915. doi:10.1038/s41573-021-00345-8.
- Association Pancreatic Cancer Committee of Chinese Anticancer. Comprehensive guidelines for the diagnosis and treatment of pancreatic cancer (2020 version). Zhonghua Wai Ke Za Zhi. 2021;59(2):81–100. PMID: 33378799. doi:10.3760/cma.j.cn112139-20201113-00794.
- Smith DR. Bibliometrics, dermatology and contact dermatitis. Contact Dermatitis. 2008;59(3):133–6. PMID: 18759892. doi:10.1111/j.1600-0536.2008.01405.x.
- Hinshaw DC, Shevde LA. The tumor microenvironment innately modulates cancer progression. Cancer Res. 2019;79(18):4557–66. PMID: 31350295. doi:10.1158/0008-5472.CAN-18-3962.
- Wang S, Li Y, Xing C, Ding C, Zhang H, Chen L, You L, Dai M, Zhao Y. Tumor microenvironment in chemoresistance, metastasis and immunotherapy of pancreatic cancer. Am J Cancer Res. 2020;10(7):1937–53. PMID: 32774994.
- Jang JE, Hajdu CH, Liot C, Miller G, Dustin ML, Bar-Sagi D. Crosstalk between regulatory T cells and tumor-associated dendritic cells negates anti-tumor immunity in pancreatic cancer. Cell Rep. 2017;20(3):558–71. PMID: 28723561. doi: 10.1016/j.celrep.2017.06.062.
- Arenas-Ramirez N, Zou C, Popp S, Zingg D, Brannetti B, Wirth E, Calzascia T, Kovarik J, Sommer L, Zenke G, et al. Improved cancer immunotherapy by a CD25-mimobody conferring selectivity to human interleukin-2. Sci Transl Med. 2016;8(367):367ra166. PMID: 27903862. doi:10.1126/scitranslmed.aag3187.
- Jacobs C, Duewell P, Heckelsmiller K, Wei J, Bauernfeind F, Ellermeier J, Kisser U, Bauer CA, Dauer M, Eigler A, et al. An ISCOM vaccine combined with a TLR9 agonist breaks immune evasion mediated by regulatory T cells in an orthotopic model of pancreatic carcinoma. Int J Cancer. 2011;128(4):897–907. PMID: 20473889. doi:10.1002/ijc.25399.
- Soares KC, Rucki AA, Kim V, Foley K, Solt S, Wolfgang CL, Jaffee EM, Zheng L. TGF-β blockade depletes T regulatory cells from metastatic pancreatic tumors in a vaccine dependent manner. Oncotarget. 2015;6(40):43005–15. PMID: 26515728. doi: 10.18632/oncotarget.5656.
- Ohl K, Tenbrock K. Reactive oxygen species as regulators of MDSC-mediated immune suppression. Front Immunol. 2018;9:2499. PMID: 30425715. doi:10.3389/fimmu.2018.02499.
- Gabrilovich DI, Nagaraj S. Myeloid-derived suppressor cells as regulators of the immune system. Nat Rev Immunol. 2009;9(3):162–74. PMID: 19197294. doi:10.1038/nri2506.
- Huang B, Pan PY, Li Q, Sato AI, Levy DE, Bromberg J, Divino CM, Chen S-H. Gr-1+CD115+ immature myeloid suppressor cells mediate the development of tumor-induced T regulatory cells and T-cell anergy in tumor-bearing host. Cancer Res. 2006;66(2):1123–31. PMID: 16424049. doi: 10.1158/0008-5472.CAN-05-1299.
- Denardo DG, Galkin A, Dupont J, Zhou L, Bendell J. GB1275, a first-in-class CD11b modulator: rationale for immunotherapeutic combinations in solid tumors. J Immunother Cancer. 2021;9(8):e003005. PMID: 34452928. doi: 10.1136/jitc-2021-003005.
- Kuang DM, Zhao Q, Peng C, Xu J, Zhang J-P, Wu C, Zheng L. Activated monocytes in peritumoral stroma of hepatocellular carcinoma foster immune privilege and disease progression through PD-L1. J Exp Med. 2009;206(6):1327–37. PMID: 19451266. doi: 10.1084/jem.20082173.
- Lu SW, Pan HC, Hsu YH, Chang K-C, Wu L-W, Chen W-Y, Chang M-S. IL-20 antagonist suppresses PD-L1 expression and prolongs survival in pancreatic cancer models. Nat Commun. 2020;11(1):4611. PMID: 32929072. doi: 10.1038/s41467-020-18244-8.
- Kaneda MM, Cappello P, Nguyen AV, Ralainirina N, Hardamon CR, Foubert P, Schmid MC, Sun P, Mose E, Bouvet M, et al. Macrophage PI3Kγ drives pancreatic ductal adenocarcinoma progression. Cancer Discovery. 2016;6(8):870–85. PMID: 27179037. doi:10.1158/2159-8290.CD-15-134.
- Hosein AN, Brekken RA, Maitra A. Pancreatic cancer stroma: an update on therapeutic targeting strategies. Nat Rev Gastroenterol Hepatol. 2020;17(8):487–505. PMID: 32393771. doi:10.1038/s41575-020-0300-1.
- Feig C, Jones JO, Kraman M, Wells RJB, Deonarine A, Chan DS, Connell CM, Roberts EW, Zhao Q, Caballero OL, et al. Targeting CXCL12 from FAP-expressing carcinoma-associated fibroblasts synergizes with anti–PD-L1 immunotherapy in pancreatic cancer. Proc Natl Acad Sci U S A. 2013;110(50):20212–7. PMID: 24277834. doi:10.1073/pnas.1320318110.
- Jiang H, Hegde S, Knolhoff BL, Zhu Y, Herndon JM, Meyer MA, Nywening TM, Hawkins WG, Shapiro IM, Weaver DT, et al. Targeting focal adhesion kinase renders pancreatic cancers responsive to checkpoint immunotherapy. Nat Med. 2016;22(8):851–60. PMID: 27376576. doi:10.1038/nm.4123.
- Symeonides SN, Anderton SM, Serrels A. FAK-inhibition opens the door to checkpoint immunotherapy in pancreatic cancer. J Immunother Cancer. 2017;5(1):17. PMID: 28239470. doi: 10.1186/s40425-017-0217-6.
- Tang D, Yuan Z, Xue X, Lu Z, Zhang Y, Wang H, Chen M, An Y, Wei J, Zhu Y, et al. High expression of galectin-1 in pancreatic stellate cells plays a role in the development and maintenance of an immunosuppressive microenvironment in pancreatic cancer. Int J Cancer. 2012;130(10):2337–48. PMID: 21780106. doi:10.1002/ijc.26290.
- Kesh K, Garrido VT, Dosch A, Durden B, Gupta VK, Sharma NS, Lyle M, Nagathihalli N, Merchant N, Saluja A, et al. Stroma secreted IL6 selects for “stem-like” population and alters pancreatic tumor microenvironment by reprogramming metabolic pathways. Cell Death Dis. 2020;11(11):967. PMID: 33177492. doi:10.1038/s41419-020-03168-4.
- Nezhad Shamohammadi F, Yazdanifar M, Oraei M, Kazemi MH, Roohi A, Mahya Shariat Razavi S, Rezaei F, Parvizpour F, Karamlou Y, Namdari H, et al. Controversial role of γδ T cells in pancreatic cancer. Int Immunopharmacol. 2022;108:108895. PMID: 35729831. doi:10.1016/j.intimp.2022.108895.
- Chabab G, Boissière-Michot F, Mollevi C, Ramos J, Lopez-Crapez E, Colombo P-E, Jacot W, Bonnefoy N, Lafont V. Diversity of tumor-infiltrating, γδ T-cell abundance in solid cancers. Cells. 2020;9(6):1537. PMID: 32599843. doi: 10.3390/cells9061537.
- Seifert AM, List J, Heiduk M, Decker R, von Renesse J, Meinecke A-C, Aust DE, Welsch T, Weitz J, Seifert L. Gamma-delta T cells stimulate IL-6 production by pancreatic stellate cells in pancreatic ductal adenocarcinoma. J Cancer Res Clin Oncol. 2020;146(12):3233–40. PMID: 32865617. doi: 10.1007/s00432-020-03367-8.
- Daley D, Zambirinis CP, Seifert L, Akkad N, Mohan N, Werba G, Barilla R, Torres-Hernandez A, Hundeyin M, Mani VRK, et al. γδ T cells support pancreatic oncogenesis by restraining αβ T cell activation [published correction appears in cell. 2020 Nov 12;183(4): 1134-1136]. Cell. 2016;166(6):1485–1499.e15. PMID: 27569912. doi:10.1016/j.cell.2016.07.046.
- Bejarano L, Jordao MJC, Joyce JA. Therapeutic targeting of the tumor microenvironment. Cancer Discov. 2021;11(4):933–59. PMID: 3381112. doi:10.1158/2159-8290.CD-20-1808.
- Ho WJ, Jaffee EM. Macrophage-targeting by CSF1/1R blockade in pancreatic cancers. Cancer Res. 2021;81(24):6071–3. PMID: 34911778. doi:10.1158/0008-5472.CAN-21-3603.
- Hopkins AC, Yarchoan M, Durham JN, Yusko EC, Rytlewski JA, Robins HS, Laheru DA, Le DT, Lutz ER, Jaffee EM. T cell receptor repertoire features associated with survival in immunotherapy-treated pancreatic ductal adenocarcinoma. JCI Insight. 2018;3(13). PMID: 29997287. doi:10.1172/jci.insight.122092.
- Le DT, Picozzi VJ, Ko AH, Wainberg ZA, Kindler H, Wang-Gillam A, Oberstein P, Morse MA, Zeh HJ, Weekes C, et al. Results from a phase IIb, randomized, multicenter study of GVAX Pancreas and CRS-207 compared with chemotherapy in adults with Previously treated metastatic pancreatic adenocarcinoma (ECLIPSE study). Clin Cancer Res. 2019;25(18):5493–502. PMID: 31126960. doi:10.1158/1078-0432.CCR-18-2992.
- Padoan A, Plebani M, Basso D. Inflammation and pancreatic cancer: focus on metabolism, cytokines, and immunity. Int J Mol Sci. 2019;20(3):676. PMID: 30764482. doi: 10.3390/ijms20030676.
- Shima H, Tsurita G, Wada S, Hirohashi Y, Yasui H, Hayashi H, Miyakoshi T, Watanabe K, Murai A, Asanuma H, et al. Randomized phase II trial of survivin 2B peptide vaccination for patients with HLA -A24-positive pancreatic adenocarcinoma. Cancer Sci. 2019;110(8):2378–85. PMID: 31218770. doi:10.1111/cas.14106.
- Vonderheide RH, Bajor DL, Winograd R, Evans RA, Bayne LJ, Beatty GL. CD40 immunotherapy for pancreatic cancer. Cancer Immunol Immunother. 2013;62(5):949–54. PMID: 23589109. doi: 10.1007/s00262-013-1427-5.
- Lau SP, van Montfoort N, Kinderman P, Lukkes M, Klaase L, van Nimwegen M, van Gulijk M, Dumas J, Mustafa DAM, Lievense SLA, et al. Dendritic cell vaccination and CD40-agonist combination therapy licenses T cell-dependent antitumor immunity in a pancreatic carcinoma murine model. J Immunother Cancer. 2020;8(2):e000772. PMID: 32690771. doi:10.1136/jitc-2020-000772.
- June CH, O’Connor RS, Kawalekar OU, Ghassemi S, Milone MC. CAR T cell immunotherapy for human cancer. Science. 2018;359(6382):1361–5. PMID: 29567707. doi: 10.1126/science.aar6711.
- Ali AI, Oliver AJ, Samiei T, Chan JD, Kershaw MH, Slaney CY. Genetic redirection of T cells for the treatment of pancreatic cancer. Front Oncol. 2019;9:56. PMID: 30809507. doi:10.3389/fonc.2019.00056.
- Bear AS, Vonderheide RH, O’Hara MH. Challenges and opportunities for pancreatic cancer immunotherapy. Cancer Cell. 2020;38(6):788–802. PMID: 32946773. doi:10.1016/j.ccell.2020.08.004.
- Nywening TM, Wang-Gillam A, Sanford DE, Belt BA, Panni RZ, Cusworth BM, Toriola AT, Nieman RK, Worley LA, Yano M, et al. Targeting tumour-associated macrophages with CCR2 inhibition in combination with FOLFIRINOX in patients with borderline resectable and locally advanced pancreatic cancer: a single-centre, open-label, dose-finding, non-randomised, phase 1b trial. Lancet Oncol. 2016;17(5):651–62. PMID: 27055731. doi:10.1016/S1470-2045(16)00078-4.
- Linehan D, Noel MS, Hezel AF, Wang-Gillam A, Eskens F, Sleijfer S, Desar IME, Erdkamp F, Wilmink J, Diehl J, et al. Overall survival in a trial of orally administered CCR2 inhibitor CCX872 in locally advanced/metastatic pancreatic cancer: correlation with blood monocyte counts. J Clin Oncol. 2018;36(5_suppl):92–. doi:10.1200/JCO.2018.36.5_suppl.92.
- Li M, Li M, Yang Y, Liu Y, Xie H, Yu Q, Tian L, Tang X, Ren K, Li J, et al. Remodeling tumor immune microenvironment via targeted blockade of PI3K-γ and CSF-1/CSF-1R pathways in tumor associated macrophages for pancreatic cancer therapy. J Control Release. 2020;321:23–35. PMID: 32035193. doi:10.1016/j.jconrel.2020.02.011.
- Han Y, Liu D, Li L. PD-1/PD-L1 pathway: current researches in cancer. Am J Cancer Res. 2020;10(3):727–42. PMID: 32266087.
- O’Hara MH, O’Reilly EM, Varadhachary G, Wolff RA, Wainberg ZA, Ko AH, Fisher G, Rahma O, Lyman JP, Cabanski CR, et al. CD40 agonistic monoclonal antibody APX005M (sotigalimab) and chemotherapy, with or without nivolumab, for the treatment of metastatic pancreatic adenocarcinoma: an open-label, multicentre, phase 1b study. Lancet Oncol. 2021;22(1):118–31. PMID: 33387490. doi:10.1016/S1470-2045(20)30532-5.
- Bockorny B, Semenisty V, Macarulla T, Borazanci E, Wolpin BM, Stemmer SM, Golan T, Geva R, Borad MJ, Pedersen KS, et al. BL-8040, a CXCR4 antagonist, in combination with pembrolizumab and chemotherapy for pancreatic cancer: the COMBAT trial. Nat Med. 2020;26(6):878–85. PMID: 32451495. doi:10.1038/s41591-020-0880-x.
- Rotte A. Combination of CTLA-4 and PD-1 blockers for treatment of cancer. J Exp Clin Cancer Res. 2019;38(1):255. PMID: 31196207. doi:10.1186/s13046-019-1259-z.
- Pretta A, Lai E, Persano M, Donisi C, Pinna G, Cimbro E, Parrino A, Spanu D, Mariani S, Liscia N, et al. Uncovering key targets of success for immunotherapy in pancreatic cancer. Expert Opin Ther Targets. 2021;25(11):987–1005. PMID: 34806517. doi:10.1080/14728222.2021.2010044.
- Yamamoto K, Venida A, Yano J, Biancur DE, Kakiuchi M, Gupta S, Sohn ASW, Mukhopadhyay S, Lin EY, Parker SJ, et al. Autophagy promotes immune evasion of pancreatic cancer by degrading MHC-I. Nature. 2020;581(7806):100–5. PMID: 32376951. doi:10.1038/s41586-020-2229-5.
- O’Reilly EM, Oh DY, Dhani N, Renouf DJ, Lee MA, Sun W, Fisher G, Hezel A, Chang S-C, Vlahovic G, et al. Durvalumab with or without tremelimumab for patients with metastatic pancreatic ductal adenocarcinoma: a phase 2 randomized clinical trial. JAMA Oncol. 2019;5(10):1431–8. PMID: 31318392. doi:10.1001/jamaoncol.2019.1588.
- TTB H, Nasti A, Seki A, Komura T, Inui H, Kozaka T, Kitamura Y, Shiba K, Yamashita T, Yamashita T, et al. Combination of gemcitabine and anti-PD-1 antibody enhances the anticancer effect of M1 macrophages and the Th1 response in a murine model of pancreatic cancer liver metastasis. J Immunother Cancer. 2020;8(2):e001367. PMID: 33188035. doi:10.1136/jitc-2020-001367.