ABSTRACT
Francisella tularensis is one of the several biothreat agents for which a licensed vaccine is needed. To ensure vaccine protection is achieved across a range of virulent F. tularensis strains, we assembled and characterized a panel of F. tularensis isolates to be utilized as challenge strains. A promising tularemia vaccine candidate is rLVS ΔcapB/iglABC (rLVS), in which the vector is the LVS strain with a deletion in the capB gene and which additionally expresses a fusion protein comprising immunodominant epitopes of proteins IglA, IglB, and IglC. Fischer rats were immunized subcutaneously 1–3 times at 3-week intervals with rLVS at various doses. The rats were exposed to a high dose of aerosolized Type A strain Schu S4 (FRAN244), a Type B strain (FRAN255), or a tick derived Type A strain (FRAN254) and monitored for survival. All rLVS vaccination regimens including a single dose of 107 CFU rLVS provided 100% protection against both Type A strains. Against the Type B strain, two doses of 107 CFU rLVS provided 100% protection, and a single dose of 107 CFU provided 87.5% protection. In contrast, all unvaccinated rats succumbed to aerosol challenge with all of the F. tularensis strains. A robust Th1-biased antibody response was induced in all vaccinated rats against all F. tularensis strains. These results demonstrate that rLVS ΔcapB/iglABC provides potent protection against inhalational challenge with either Type A or Type B F. tularensis strains and should be considered for further analysis as a future tularemia vaccine.
Introduction
Francisella tularensis is a gram-negative bacterium and the causative agent of tularemia. F. tularensis is one of the most infectious bacterial pathogens for humans as it has been reported that inoculation or inhalation of less than 10 organisms can cause disease.Citation1 Due to the threat posed by its low infectious dose, severity of the disease, and ability for aerosol dissemination, F. tularensis has been listed as a Tier 1 Select Agent by the United States (U.S.) Department of Health and Human Services.Citation2 The two F. tularensis subspecies that are a risk to humans are the highly virulent F. tularensis subsp. tularensis (Type A) and the less virulent F. tularensis subsp. holarctica (Type B). Typically, the Type A strains are limited to North America, whereas Type B strains are present across the Northern Hemisphere.Citation3–5
F. tularensis infects a wide range of animal species, including humans. Humans can be exposed through a number of routes, the most common being to the skin through the bite of an infected insect or other arthropod vector.Citation5–8 F. tularensis can be transmitted to humans through the mucous membranes, gastrointestinal tract, and lungs. Thus, tularemia can range from an ulceroglandular to a much more severe pneumonic or typhoidal disease. The greatest concern to the biodefense community is the intentional use of aerosolized F. tularensis.Citation2,Citation9–11 Despite this threat, a U.S. Food and Drug Administration (FDA) approved tularemia vaccine does not currently exist.
Live Vaccine Strain (LVS) was derived from an unknown Type B strain in the former Soviet Union. The vaccine is delivered by scarification, forms a small lesion, and provides some level of protection against challenge.Citation1,Citation12–14 However, LVS is available only as an Investigational New Drug vaccine in the U.S. In addition, LVS is not considered an ideal vaccine for several reasons, including significant cutaneous reactogenicity, and documented breakthrough in protection.Citation1,Citation15–18 Therefore, an improved vaccine to prevent tularemia is needed.
When evaluating a new vaccine, protection studies have typically been completed with the prototype Schu S4 strain that was obtained from a human ulceroglandular disease.Citation17 However, the virulence of F. tularensis strains can vary between Type A and B isolates.Citation19–23 Therefore, a successful pneumonic tularemia vaccine needs to protect against a wide variety of F. tularensis strains which may differ in geographic origin or virulence attributes. In order to facilitate the efficacy testing of new vaccines against pneumonic tularemia, we previously developed a well-characterized panel of F. tularensis strains which represents a variety of historical, clinical, and environmental isolates.Citation19 In this study, we have utilized our F. tularensis panel and a rat aerosol model of pneumonic tularemia to test the efficacy of the rLVS ΔcapB/iglABC vaccine, an improved version of the LVS vaccine. This vaccine vector, LVS ΔcapB, is a highly attenuated version of LVS that also serves as a platform to express recombinant immunoprotective antigens of target pathogens and thereby protect against several pathogens.Citation24–26 We used a version of rLVS ΔcapB that expresses a fusion protein comprising the immunodominant epitopes of F. tularensis, IglA, IglB, and IglC (IglABC).Citation24,Citation27 We show that this vaccine is able to protect Fischer rats from high challenge doses of aerosolized bacteria from multiple strains of F. tularensis.
Materials and methods
Bacterial strains and growth media
The fully virulent F. tularensis strains used in this study were previously described, prepared according to International Organization for Standardization/ISO standards and guidelines for biological reference materials, and generated as single-use vials.Citation19 The down-selected strains were FRAN244, FRAN254, and FRAN255. FRAN244 refers to the Schu S4 strain obtained from BEI Resources (NR-10492).Citation17 FRAN254 is a Type A1a strain obtained from a tick, and FRAN255 is a Type B isolate that was obtained from the pleura of a male in Kentucky, USA.Citation19 The vaccine strains tested in this study were LVS and rLVS ΔcapB/iglABC (rLVS).Citation24,Citation27 Frozen original stocks of these strains were thawed; diluted 1000-fold to ~106 CFU/ml; 0.1 ml (~105 CFU) plated on chocolate agar plates containing 1% Isovitalex (Becton Dickinson) for 3 days at 37°C; colonies scraped into PBS, centrifuged, and the pellet suspended in PBS and 20% glycerol; the suspension dispensed into tubes (1 ml/tube); and the tubes stored at −80°C. Post-freeze titers were obtained on chocolate agar plates before the use of vaccines for immunization. Growth of all strains was performed on chocolate agar plates (Remel) or brain heart infusion broth supplemented with 1% Isovitalex at 37°C.
Vaccinations
Female Fischer 344 rats (6–8 weeks old) were obtained from Charles River Laboratories and allowed a week to acclimate prior to use. The frozen vaccine strains (LVS or rLVS) were thawed on ice, and then the intended CFU doses were administered to the rats subcutaneously in 100 µl injections. Naïve rats received only PBS via the same route. The vaccine dosing and intervals are described below with eight rats per group.
Aerosol challenges
F. tularensis challenge material was prepared from brain heart infusion cultures grown overnight in a 37°C shaker at 200 rpm. The cultures were adjusted for various challenge doses as determined by serial dilutions and plating. We administered aerosolized doses of the F. tularensis strains using a dynamic 30-l humidity-controlled Plexiglas whole-body exposure chamber, as previously described.Citation28 We obtained an estimate of the inhaled doses as previously described.Citation29–31 For all challenge experiments, rats were monitored at least daily, and mortality rates (to include euthanasia when moribund in accordance with early endpoint criteria) were recorded for 21 days.
Pathology
Postmortem tissues (lung, spleen, and liver) were collected from the challenged rats and routinely processed. Briefly, tissues were fixed in 10% neutral buffered formalin, embedded in paraffin, and sectioned for hematoxylin and eosin staining. At least a single section of the above tissues were examined by a board certified veterinary pathologist and were subjectively graded on the severity of necrosis/inflammation: minimal (involving <10% of the tissue), mild (involving 11–25% of the tissue), moderate (involving 26–50% of the tissue), marked (involving 51–79% of the tissue), or severe (involving ≥80% of the tissue).
Immunological analysis
Sera were obtained from anesthetized rats prior to vaccination (Pre-Vax), 16 days following the last dose of the vaccine (Post-Vax), and for the survivors at the end of the study at 21 days post challenge (Survivors) by either submandibular vein or cranial vena cava bleeds. During the bleeding process (Pre-/Post-Vax) to obtain sera, some of the rats did not recover from the procedure; the numbers of rats in each group in the protection studies are listed in the respective figures.
Immunoglobulin (Ig) total IgG (IgG) and subclass IgG titers (IgG1, IgG2a, -2b, and -2c) from rats were determined by an ELISA performed in 96-well, Immulon 2 HB, round-bottom plates (Thermo Fisher). Suspensions of FRAN244 or FRAN255 were irradiated in a JL Shepherd irradiator Model 109–68 at approximately 21 kGy on dry ice and confirmed to be inactivated. These irradiated samples were individually used as antigens and diluted in 0.1 M carbonate buffer, pH 9.5, to a concentration of 10 μg/ml. Plates were covered and stored overnight at 4°C. The plates were washed five times with 1X wash buffer (1× PBS, 0.05% Tween 20) with a Biotek ELx405ts plate washer and incubated with a blocking buffer (1% Casein in PBS, Pierce/Fisher Scientific) for 30 min at 37°C. Blocking buffer was removed by washing as stated above, then twofold serial dilutions of rat sera, at a starting dilution of no less than 1:50, were made with antibody assay diluent (1X PBS, 0.25% Casein) in triplicate, and plates were incubated for 1 h at 37°C. After the plates were washed as previously mentioned, diluted goat anti-rat-IgG, -IgG1, -IgG2a, -IgG2b, or -IgG2c horseradish peroxidase conjugate (1:5,000; Southern Biotechnology Associates, Inc.) was added to the wells and the plates were incubated for 30 min at 37°C. After the plates were washed as previously stated, buffered hydrogen peroxide and 3,3′,5,5′-tetramethylbenzidine solution (Pierce, Thermo Fisher) were added to each well, and plates were incubated for 20 min at 37°C. The reaction was stopped with 2 N sulfuric acid, and the amount of bound antibody was determined colorimetrically by reading at 450 nm with a reference filter (570 nm) using a Biotek ELx808 plate reader. The results are reported to be the reciprocal of the highest dilution giving a mean OD of at least 0.1 (which was at least twice the background) ± 1 SD.
Statistics
The LD50 values were estimated by Probit analysis, using log10 dose as the predictor. The effect of strains on LD50 is tested by a chi-square test based on the Probit model, under the assumption of a common effect of log10 dose. The analysis is implemented in SAS PROC PROBIT, SAS PROC LIFETEST, and SAS PROC LIFEREG, and SAS version 9.4. The survival curves are compared by a Log-rank test for trends with an analysis completed with GraphPad Prism 9.2.0. Rat weight changes were compared between any two groups by one-way ANOVA with Tukey’s post comparison test with analysis completed with GraphPad Prism 9.2.0. For the antibody titers, data were log10 transformed prior to analysis. Pairwise treatment groups were compared using the Wilcoxon rank sum test. The analysis was implemented in SAS version 9.4 (Carey, NC).
Results
Determine rat LD50 values with F. tularensis panel strains
In our previous study characterizing this panel of F. tularensis strains, we determined the LD50 values in BALB/c mice to confirm virulence.Citation19 In previous studies, we also demonstrated that the rLVS vaccine is highly protective in the BALB/c mouse model of lethal pneumonic tularemia.Citation24 However, mice show a different pattern of susceptibility to F. tularensis strains than humans, as unlike humans, mice are highly sensitive to LVS and Francisella novicida. In this respect, the Fischer rat, which is also resistant to LVS and F. novicida, more closely reflects human susceptibility.Citation32,Citation33 Therefore, we extended our studies to the Fischer rat model of lethal pneumonic tularemia.
As shown in and , we performed whole-body aerosols to determine the LD50 value for Fischer rats with our down-selected strains FRAN244 (LD50 = 433 CFU), FRAN254 (a Type A strain derived from a tick) (LD50 = 23 CFU), and FRAN255 (a Type B strain) (LD50 = 5,672 CFU). From these determinations, the estimated inhaled LD50 for the three strains in rats had a wide distribution, and the FRAN254 and FRAN255 strains were significantly different from the FRAN244 strain. Furthermore, the range of LD50 values obtained for this model using these strains is different as compared to what we previously reported for intranasal challenge in mice where the LD50 for all virulent strains was <1 CFU.Citation19
Figure 1. Survival data of rats challenged with aerosolized F. tularensis strains for determination of LD50. Groups of Fischer rats (n = 8/group) were challenged by whole body aerosolization with strains FRAN244, FRAN254, or FRAN255 as indicated, and survival monitored. The calculated LD50 values from these experiments are shown in .
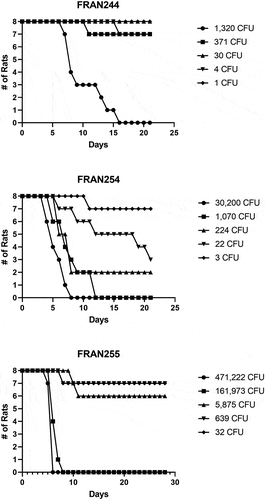
Table 1. Rat aerosol LD50 analysis of F. tularensis strains.
The rLVS vaccine protects rats against Schu S4 aerosolization challenge
Previously, it was demonstrated that the rLVS vaccine was able to protect mice against intranasal challenge with Schu S4.Citation24 Here, we demonstrate the efficacy of rLVS against challenge with aerosolized FRAN244 strain in Fischer rats. The rats were vaccinated with rLVS by subcutaneous inoculation with ~106 (2 doses), ~107 (1, 2, and 3 doses), or ~108 (2 doses) CFU as shown in and . As indicated, multiple-vaccine doses were given 3 weeks apart. The comparators for this study were rats receiving a single dose of parent LVS at ~107 CFU and naïve rats receiving a single dose of PBS. Prior to and during the vaccinations, the weights were collected weekly for all rats (vaccinated and naïve), and no weight loss or other adverse side effects were observed (), demonstrating that this vaccine is safe and well tolerated in rats.
Figure 2. Efficacy of rLVS against aerosol challenge with F. tularensis (FRAN244) in Fischer rats. (a). Experimental schedule. Fischer rats (n = 7 or 8/group) were immunized subcutaneously (SQ) once with PBS (n = 7/group), 107 CFU LVS (n = 8/group), or 107 CFU rLVS (n = 7/group); twice with 106 CFU rLVS (n = 8/group), 107 CFU rLVS (n = 7/group), or 108 CFU rLVS (n = 7/group) rLVS; or three times with 107 CFU rLVS (n = 8/group), 3 weeks apart; challenged with aerosolized F. tularensis FRAN244 (72 LD50) at Week 9; and monitored for signs of illness, weight change, and death for 3 weeks post challenge, as indicated. (b). Survival post immunization and respiratory challenge. The survival curves are compared by log-rank test for trend, ****, p < 0.0001. (c). All rats were weighed weekly. Weight change post immunization and post respiratory challenge.
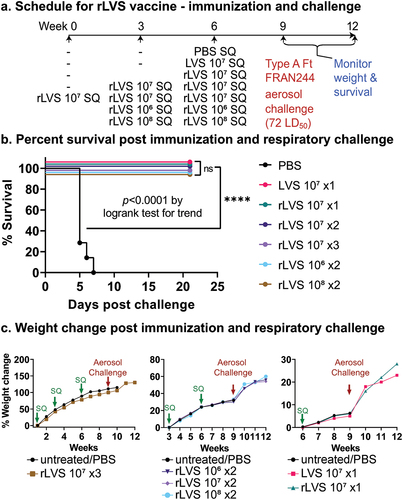
Table 2. Vaccine doses for testing protection against FRAN244 in rats.
Three weeks after the final vaccination, the rats were challenged by whole-body exposure to aerosolized FRAN244. Rats from each group were included in each of the multiple aerosol runs to ensure equal representation during the challenge sprays. The average inhaled dose for the FRAN244 challenged rats was 72 LD50. As shown in , all rats administered a vaccine (either LVS or rLVS) survived the FRAN244 challenge, and no outwardly apparent clinical signs of infection were noted. rLVS provided 100% protection whether administered once, twice or three times at a dose of 10Citation7 CFU, and whether administered twice at a dose of 106, 107 or 108 CFU. In contrast, all rats in the naïve group succumbed to infection or were euthanized in accordance with early endpoint euthanasia criteria by Day 8 (). At the end of the study, 21 days post-challenge, a portion of the lungs and spleens from the surviving rats were processed for pathology (described below), and the remainder of the organs were homogenized, diluted, and plated for CFU counts. None of the surviving animals had any detectable F. tularensis bacteria (limit of detection is 10 CFU).
The rLVS vaccine can protect rats against inhalational tularemia caused by diverse F. tularensis strains
Based upon the protection provided by rLVS against the FRAN244 strain, we proceeded to challenges of vaccinated rats with the down-selected F. tularensis panel strains (FRAN254 and FRAN255) (). In addition, the vaccination strategy for rLVS was modified to consist of a single vaccination with ~107 CFU or two vaccinations at ~108 CFU. The controls for this study were again administered PBS (naïve) or the LVS parent with a single vaccination at ~10Citation7 CFU ( and ). Prior to and during vaccinations, the weights were collected weekly for all rats (vaccinated and naïve), and again no weight loss or other adverse side effects were observed for those rats receiving the vaccine compared to PBS alone ().
Figure 3. Efficacy of rLVS against aerosol challenge with F. tularensis FRAN254 and FRAN255 in Fischer rats. (a). Experimental schedule. Fischer rats were immunized subcutaneously (SQ) once at Week 0 with PBS (naïve, n = 6 and 7/group for Type A and Type B challenge, respectively), 107 CFU LVS (n = 7 and 8/group for Type A and Type B challenge, respectively), 107 CFU rLVS (n = 8/group for both Type A and Type B challenge) or twice at Week 0 and Week 3 with 108 CFU rLVS (n = 8/group for both Type A and Type B challenge); challenged with aerosolized F. tularensis Type A (FRAN254, 233 LD50) or Type B (FRAN255, 73 LD50) at Week 6; and monitored for signs of illness, weight change, and death for 3 weeks, as indicated. (b). Weight change and survival after vaccination and challenge. Weight changes were compared between any two groups by one-way ANOVA with Tukey’s post comparison’s test. No significant differences were found. The survival curves are compared by log-rank test for trend (Prism 9.2.0). ***, p < 0.001; ****, p < 0.0001.
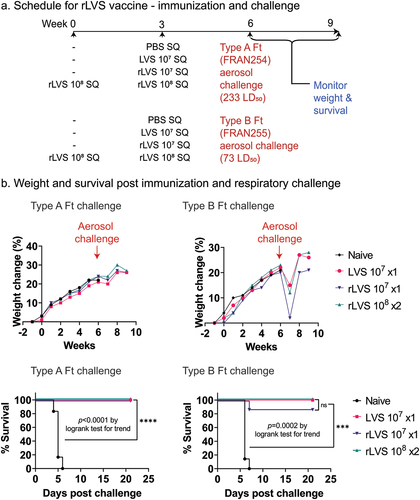
Table 3. Vaccine doses for testing protection against FRAN254 and FRAN255 in rats.
Three weeks after the final vaccination, the rats were challenged by whole-body aerosolization with FRAN254 or FRAN255. The average inhaled dose for the FRAN254 challenged rats was 233 LD50 and 73 LD50 for FRAN255. As shown in , all rats immunized with either LVS or rLVS survived the high challenge dose with FRAN254 (Type A), and no clinical signs were observed post-challenge for all of the vaccinated groups. In contrast, all naïve rats challenged with FRAN254 succumbed to infection or were euthanized by Day 6 (, lower left graph). At the end of the study, 21 days post-challenge, a portion of the lungs and spleens from the surviving rats were processed for pathology (described below) and the remainder was homogenized, diluted, and plated for CFU counts. Again, there was no evidence of any F. tularensis bacteria in the examined organs in any of the surviving rats.
For those rats exposed to aerosols of the Type B strain FRAN255, all rats immunized with LVS or twice with rLVS (108 CFU) survived to the end of the study at Day 21 post-challenge (, lower right graph). For rats immunized just once with a low dose (107 CFU) of rLVS, all but one of the rats survived to the end of the study; the one rat that succumbed died on Day 7. Among the surviving rats, low-level clinical signs of infection, such as chromodacryorrhea, were observed for some of the rats in all vaccinated groups. These first appeared on Day 5 post-challenge and resolved by Day 8 (data not shown). In addition, weight loss (, upper right graph) was also detected for the vaccinated rats post-challenge. However, their weights did rebound by the second week post-challenge. The results for the naïve rats were drastically different, as all animals succumbed to infection by Day 7.
Histopathological analysis of vaccinated rats
In vaccinated animals challenged with the FRAN244/SchuS4 strain, as described in , no significant pathological differences were observed among the challenged animals within any of the groups that received rLVS at different concentrations or among animals that received the traditional LVS vaccine (, top row, middle, and rightmost images). All animals from every vaccinated group that were reviewed microscopically had similar histopathologic changes within the lungs, consisting of multifocal areas of alveolar inflammation comprising macrophages, lymphocytes, and plasma cells, with a severity ranging from mild to moderate. The lesions observed in all vaccinated animals were considered to be a consequence of aerosolized F. tularensis infection and resolving. In marked contrast, unvaccinated rats infected with FRAN244, all of which had succumbed to infection, showed alveolar necrosis and damage, more severe and widespread inflammation (moderate to marked) and hyperplasia of bronchus associated lymphoid tissue (, top row, leftmost image).
Figure 4. Histopathology of lungs from F. tularensis challenged rats. Rats were unvaccinated (naïve) or vaccinated with rLVS, or LVS, as indicated; challenged with FRAN244 (top), FRAN254 (middle), or FRAN255 (bottom); and monitored for survival for 21 days. All naïve rats were necropsied at death or following euthanasia when moribund. Vaccinated rats survived and were euthanized and necropsied at the end of the experiment; however one rLVS (single dose) rat challenged with FRAN255 did succumb). Lungs were examined for histopathology. A representative animal from each group is shown. For the rLVS group, all images shown are from the single dose 107 CFU vaccinated group.
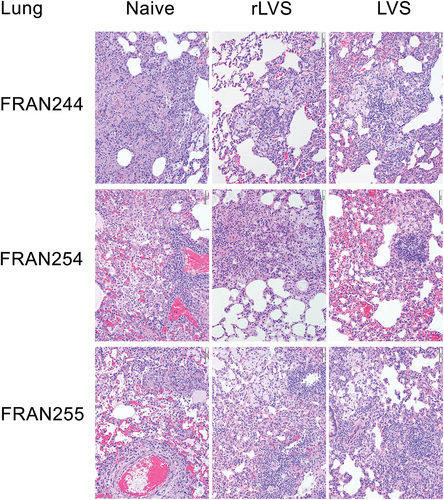
Findings within the liver of vaccinated animals challenged with FRAN244 were minor and classified as incidental; these included minimal inflammatory infiltrates with occasional single-cell necrosis (supplementary Figure S1, left panel, top row). In contrast, unvaccinated rats challenged with FRAN244 displayed multifocal areas of necrosis within hepatic lobules as well as hepatocyte vacuolation and inflammation. In vaccinated rats that survived challenged with FRAN244, the spleen appeared normal in almost all animals; only a few animals displayed decreased cellularity in the marginal zone of the white pulp (supplementary Figure S1, right panel, top row). Again, in marked contrast, the spleens of unvaccinated rats, all of which succumbed to the challenge with FRAN244, showed necrosis in both the red and white pulp as well as lymphoid depletion, inflammation, and accumulation of fibrin and/or fibrin thrombi.
In rats challenged with FRAN254, those vaccinated with LVS or rLVS, all of whom survived the challenge, were again protected against lesions in the lung, liver, and spleen. Specifically, in the lung, the vaccinated animals were spared the necrosis/alveolar damage and severe inflammation throughout the lung observed in the naïve controls (, middle row). In the liver, LVS or rLVS vaccination provided protection against the necrosis as well as more severe inflammation and hepatocyte vacuolation seen in naïve controls (supplementary Figure S1, left panel, middle row). In the spleen, vaccinated animals were protected against necrosis, more severe inflammation, fibrin accumulation, and lymphoid depletion observed in naïve controls (supplementary Figure S1, right panel, middle row). Additionally, when comparing FRAN254 infected animals that had been administered two different rLVS regimens (), the lesion type, severity, and frequency were very similar between these groups, and the lesion severity was either minimal or mild in the majority of cases in the lung, liver, and spleen.
In rats challenged with FRAN255, the histopathologic analysis again showed those vaccinated with LVS or rLVS were protected against lesions in the lung, liver, and spleen compared with unvaccinated animals. Specifically, in the lung, this included protection against necrosis/alveolar damage (, bottom row). Inflammation (alveolar, perivascular) in the lung remained elevated in vaccinated animals, similar to the severity seen in naïve controls although with a different character (e.g., many foamy macrophages and absence of neutrophils). In the liver, vaccinated rats were protected against necrosis, as well as more severe inflammation and hepatocyte vacuolation seen in naïve animals (supplementary Figure S1, left panel, bottom row). In the spleen, vaccinated rats were protected against necrosis, more severe inflammation, fibrin accumulation and/or thrombi and lymphoid depletion seen in naïve animals (supplementary Figure S1, right panel, bottom row).
When comparing the pathology among rats immunized by different vaccination regimens, the lesion type, severity, and frequency were very similar among the groups and the lesion severity was minimal in the liver and spleen. Interestingly, some differences were noted among the groups in the lungs of the survivors (). Inflammation and BALT hyperplasia were elevated in the lung in all three vaccinated groups and ranged in severity from mild to marked. In a subset of animals, those immunized once with rLVS had lower severity scores for inflammation and BALT hyperplasia than the animals immunized with rLVS twice or with LVS. There was a single FRAN255 infected animal vaccinated once with a low dose (107) of rLVS that was found dead on day 7; this rat had lesions more consistent with those seen in control animals, suggesting that this single animal was not protected by the vaccine protocol. Supplementary Table S1 provides additional details on the histopathological analysis of these challenge experiments.
Antibody analysis
Total serum IgG response was assessed at three discrete timepoints in rats: 1) prior to vaccination (Pre-vax), 2) 16 days following the last dose of the vaccine (Post-vax), and 3) from the survivors at the end of the study at 21 days post challenge (Survivors). Antibody titers were evaluated in all animals against irradiated FRAN244 (Type A), FRAN255 (Type B), or both antigens as listed. In Fischer rats, IgG2b/2c indicates a Th1 type response and IgG1/2a indicates a Th2 type response.Citation34,Citation35
Experiment 1: challenge with FRAN244
Following vaccination but prior to challenge with Schu S4, the highest total IgG responses directed against irradiated F. tularensis (FRAN244) were observed in rats vaccinated with rLVS x2 (108 CFU) followed by rats vaccinated with rLVS x3 (107 CFU) (). Of the four IgG subclass antibodies (IgG1, IgG2a, IgG2b, and IgG2c), the LVS-based vaccines appear to mediate an IgG2b dominant response following vaccination (). Rats vaccinated with rLVS x2 (108 CFU) and rLVS x3 (107 CFU) had significantly greater IgG2b titers than other vaccine groups (p < 0.05). In addition, with respect to the IgG2a response, rats vaccinated with rLVS x3 (107 CFU) had a significantly greater endpoint titer (50,625) relative to the other vaccine groups (p < 0.05). The IgG1 titers in all vaccinated groups were near background levels and similar to those of the control group. As expected, a higher concentration and a greater number of doses induced higher antibody responses.
Figure 5. Total serum IgG antibody in rats pre- and post-challenge (survivors) with Type A F. tularensis FRAN244/Schu S4 strain. Animals were immunized and challenged as described in the legend to . Serum IgG antibody and subclasses specific to irradiated FRAN244 (Type A antigen) were assayed. a. Total serum antibody and subclasses in immunized rats prior to challenge with aerosolized F. tularensis Type A FRAN244/Schu S4 strain. For IgG, IgG2a, IgG2b, and IgG2c, all vaccinated groups had significantly greater titers than the PBS group (p<0.01-p<0.001). For IgG1, all vaccinated groups except LVS, rLVS 107 x1 rLVS 107 x2 groups had significantly greater titers than the PBS group (p <0.05 - p <0.001). Values represent median with interquartile range (Q1 and Q3 for the whiskers) of serum antibody for n = 7 or 8 per group. b. Total serum antibody and subclasses in immunized rats surviving challenge with aerosolized F. tularensis Type A strains. Values represent median with interquartile range (Q1 and Q3 for the whiskers) of serum antibody. Notes: in Fischer rats, IgG2b/2c indicates a Th1 type response and IgG1/2a indicates a Th2 type response. Values that are significantly different between two groups are marked with asterisk(s) over an open horizontal line crossing above the two groups. * p <0 .05; ** p < 0.01; and *** p < 0.001. Additional descriptive statistical values are included in Supplemental Tables S2–5.
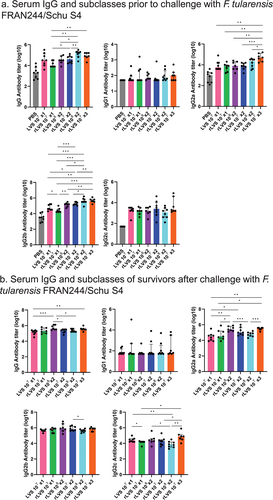
With respect to survivors of FRAN244 challenge, the total IgG antibody response of most vaccine groups was substantially increased relative to the pre-challenge antibody titers (). Furthermore, the IgG2b responses were still the most pronounced in all vaccine groups followed by the IgG2a and then the IgG2c responses, while the IgG1 response did not appear to be affected by the challenge. Surprisingly, survivors of the rLVS x2 (108 CFU) vaccinated group did not generate as notable of an increase in antibody titers relative to other vaccine groups (). Unlike the antibody responses following vaccination, the antibody responses following the challenge did not appear to be as dependent upon the concentration or number of vaccine doses.
Experiment 2: challenge with FRAN254 and FRAN255
Prevaccination baseline antibody titers for Type A and Type B antigens were obtained in all groups; there were no statistically significant differences among groups (supplementary Figure S2). Sixteen days following vaccination, the antibody response to both antigens [irradiated FRAN244 (Type A) and FRAN255 (Type B)] was significantly enhanced in all vaccine groups (p ≤ 0.0016 vs. PBS) and the magnitudes of the antibody titers were comparable against both antigens. However, the antibody titer following a single low dose of the rLVS (107 CFU) was significantly lower relative to the other vaccine groups (p < 0.05 vs. LVS or rLVS × 2) ().
Figure 6. Total serum IgG antibody in rats immunized with rLVS vaccines prior to and post challenge with Type A F. tularensis FRAN254 or Type B FRAN255 strain. Animals were immunized and challenged as described in the legend to . Sera were collected in immunized rats prior to and after challenge with Type A or Type B strains and assayed for total IgG antibody specific to irradiated FRAN244 (Type A antigen) and FRAN255 (Type B antigen). (a). Prior to challenge; All vaccinated groups in Panel a had significantly greater titers than the PBS group (p <0.01-p <0.0001). (b). Post challenge (survivors only). Values represent median with interquartile range (Q1 and Q3 for the whiskers) of serum antibody titers for n = 6–8/group. * p < 0.05; ** p <0 .01; and *** p < 0.001. Additional descriptive statistical values are included in Supplemental Tables S6,7.
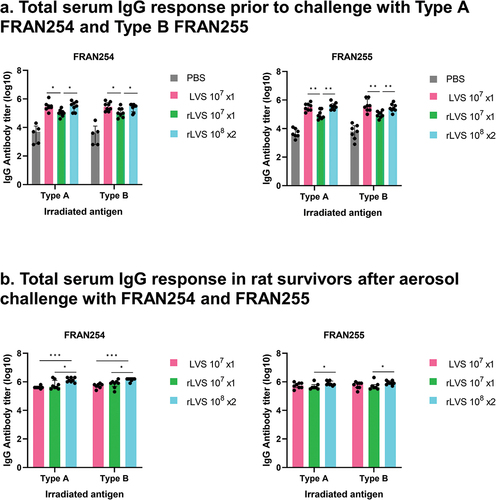
Three weeks following the challenge, the antibody response in surviving animals was substantially increased in all vaccine groups relative to pre-challenge titers. Following the challenge with FRAN254, rats vaccinated with a double dose of rLVS had significantly higher endpoint titers against both FRAN244 (Type A) and FRAN255 (Type B) antigens (p <0 .05 vs. LVS and rLVS). Following FRAN255 challenge, the higher titer of the rats immunized with a double dose of rLVS only reached significance relative to rats administered a single dose of rLVS (p < 0.05 vs. rLVS). Surviving rats from the LVS and rLVS vaccinated groups had similar antibody titers relative to each other at the conclusion of the study ().
Discussion
Currently, the U.S. biodefense community is lacking an approved FDA vaccine to prevent tularemia. Attenuated strains of pathogens have the potential to be used as live-attenuated vaccines that establish mild infections in the host and mimic the natural infection which allows for the presentation of diverse antigens to the host immune system to promote the induction of both antibody and cell-mediated immune responses.Citation36,Citation37 Some of the concerns with the unlicensed LVS are in regard to its safety and reactogenicity, despite its having two major attenuating deletions (FTT0918 and pilA) and several minor deletions.Citation38 However, a modified version of LVS (rLVS ΔcapB), with a third major attenuating deletion in capB, was demonstrated to be much less virulent than the parent LVS (intranasal LD50 > 10,000 fold that of LVS in highly susceptible mice) and has the potential to be used as a vaccine platform to express immunogenic proteins against multiple pathogens in addition to F. tularensis (Yersinia pestis, Bacillus anthracis, and severe acute respiratory syndrome coronavirus 2).Citation24–26,Citation39 The rLVS ΔcapB platform was engineered to express epitopes of the F. tularensis type VI secretion proteins IglA, IglB, and IglC. The vaccine rLVS ΔcapB/iglABC (rLVS), administered intradermally or intranasally, was shown to be highly protective in mice against respiratory challenge with the Schu S4 strain of F. tularensis. Here, we further demonstrate the potential of rLVS as a vaccine in the rat model of tularemia by whole-body aerosol challenge with not only the prototype Schu S4 strain but additional strains of F. tularensis from a diversity panel.Citation19
Our results show that the rLVS vaccine was able to protect against the SchuS4 strain in addition to two down-selected strains, FRAN254, a highly virulent Type A strain derived from a tick, and FRAN255, a clinically derived Type B strain. We previously characterized these strains and determined the LD50 in a mouse intranasal challenge model. The LD50 for each of these three strains in BALB/c mice was only 1 CFU.Citation19 However, when testing these strains in the Fischer rat model following whole-body aerosol challenge, the differences in LD50 for these strains became apparent (). The differences in the LD50 for FRAN254 (Type A & LD50 = 23 CFU) and FRAN255 (Type B & LD50 = 5,672 CFU) were each statistically significant when compared to the prototype Schu S4 (designated as FRAN244 for this study) having an LD50 of 433 CFU (). The higher aerosol LD50 value determined for FRAN255 as when compared to the Type A strains would not be unexpected as Type B strains are typically described as being less virulent than Type A strains.Citation40 In contrast, FRAN254, an additional Type A strain derived from a tick, was found to have a lower LD50 as compared to the Schu S4 strain when tested in rats. These data further underscore the high level of susceptibility of mice to F. tularensis infection and support the choice of the rat model, which better reflects human susceptibility to F. tularensis than mice, as an appropriate rodent species for vaccine efficacy studies.Citation32,Citation33,Citation41–44 More importantly, these results highlight the LD50 differences between isolates of F. tularensis and the critical need to test prospective tularemia vaccine candidates against more than just the prototype Schu S4 laboratory strain to assure protection against multiple strains.
As described here, rats receiving the rLVS vaccine in any of the doses/series provided were protected against high LD50 challenges of the respective strains. Complete protection was observed following challenges with either of the Type A strains used, FRAN244 or a tick derived strain FRAN254. Interestingly, following a challenge with the high LD50 Type B strain (FRAN255), one rat which only received one low dose of rLVS succumbed to infection after challenge. In addition, we noted that all rats vaccinated with either LVS or rLVS displayed some low-level clinical signs of infection, which were quickly resolved. During histopathologic analysis at the end of the study, lungs from survivors of FRAN255 challenge showed elevated levels of inflammation (alveolar, perivascular) that consisted of many foamy macrophages and no neutrophils. It is possible that these results following challenge with FRAN255 are due to the higher CFU numbers of bacteria needed to reach 70 LD50 of the less virulent Type B strain versus the equivalent CFU numbers to reach 70 LD50 of FRAN244. We note that some of these pathologic differences between the naïve control and vaccinated groups may not only be due to the effect of vaccination but also to the different time points of sampling in the course of disease (i.e., naïve animals that succumbed during the acute stage/day 5 versus vaccinated animals that survived to the end of study/subacute-chronic stage/day 21). Nevertheless, the rLVS vaccine offered protection and considerable melioration of disease caused by the Type B strain. Additional experimentation could include sampling the vaccinated versus naïve rats at set time points post-challenge to allow a better comparison of pathologic differences in protection and to determine what level of infection, as assessed by CFU counts from lungs and spleens, occurs for the vaccinated animals. In addition, considerations should be given to exploring the protection provided by rLVS against challenge with additional Type B F. tularensis strains.
Following vaccination with two doses of rLVS, a robust total IgG response was induced that was comparable to a single LVS vaccination (). Due to the targeted deletion of the capsule synthesis gene (capB), the rLVS was strikingly more attenuated in BALB/c mice, with an intranasal LD50 dose that was >10,000-fold relative to the parental LVS. Furthermore, rLVS replication in the lungs was significantly lower at the peak of infection and was cleared from the lungs, liver, and spleen faster relative to LVS.Citation39 Hence, when administered at the same relatively low dose (107), a double dose of rLVS was necessary to achieve antibody titers similar to those observed with LVS. Furthermore, increasing the rLVS dose improved the endpoint titers that surpassed titers produced following LVS vaccination. The predominant IgG2b as well as IgG2c response, of which the latter was much less induced, was indicative of a Th1 skewed response, which increased following vaccination with increasing dose and number of injections.Citation34 Likewise, the Th2 associated IgG2a response was much less induced, while the IgG1 tiers remained close to baseline levels ().Citation35 A similar trend in total IgG titers was observed in survivors following the challenge with FRAN244, although the challenge served as a heterologous boost and drastically increased the overall titers in all vaccinated groups (). A strong humoral response plays a critical role in protecting against Francisella infection. Previous studies have shown that prophylactic treatment of rats with purified immune IgG prior to Schu S4 challenge provided significant protection and diminished bacterial growth.Citation45 In addition, in both mouse and rat models, the protection afforded by antibodies was linked to strong T cell dependent responses for bacterial clearance of which the CD8 T cells appear to play a more critical protective role relative to CD4 T cells, although both cell populations are necessary.Citation45,Citation46
The antibody response was also similar against both FRAN244 (Type A) and FRAN255 (Type B) strains following vaccination with either LVS or rLVS in sera collected pre-challenge and in the surviving rats (). Of note, there was roughly a 10-fold difference in titers between the two vaccine studies ( vs. ). Rats vaccinated in the second experiment () received roughly ~4- to 8-fold more bacteria per dose relative to the doses in the initial study () and thus, this increased vaccine dose may have contributed to higher antibody titers. Furthermore, it is well established that the phenotypic diversity of Francisella colony morphology termed phase or antigenic variation is prominent and contributes to vaccine lot variability and dramatically affects virulence and immunogenicity based on a multitude of phenotypic attributes and their prominence within each lot, such as the ratios of the blue (induce protective immune response) vs. gray (do not induce immunity) variants.Citation12,Citation17,Citation47–50 This confounding factor during the preparation of these vaccines may also lead to variability in vaccine efficacy and impede precise reproducibility of antibody titers across current studies. However, this limitation may be addressed with cultivation of these vaccines under-improved cGMP conditions, which have been shown to yield 100% blue phenotypes that are associated with conferring protection.Citation47 However, for the studies described here, although the vaccination studies were performed at different times, the LVS and rLVS used were from the same frozen preparations and therefore any variants present in these vaccines would have been the same. Furthermore, the frozen vaccine stocks were prepared by rapid growth on chocolate agar so as to minimize the likelihood of phase variation.Citation51
Our current study has focused on the use of the rLVS vaccine as this is the first vaccine tested using our F. tularensis diversity panel to demonstrate protection against aerosol challenge in the Fischer rat model. Several other live and subunit vaccines continue to be explored with some showing promising results, though these studies have been usually limited to mouse models or challenges with only Schu S4.Citation52–60 Additional live tularemia vaccine candidates, such as the Francisella novicida iglD and the F. tularensis Schu S4-ΔclpB mutant strains, have published studies demonstrating protection with these vaccines in rats or nonhuman primate models of pneumonic tularemia with the Schu S4 strain.Citation61,Citation62 It would be important to test these vaccine candidates against additional F. tularensis strains to assure protection against multiple virulent strains.
We have shown that immunization with the rLVS vaccine induces high levels of protection against exposure to three different F. tularensis strains in Fischer rats to the same level of protection as observed with the parent LVS. However, rLVS offers additional benefits that LVS does not, such as a decreased level of virulence (at least in a murine model of tularemia), which could address one of the issues hindering LVS from receiving FDA approval.Citation39 In addition, rLVS has the ability to be engineered to express multiple protective antigens against multiple pathogens rather than just F. tularensis alone; this would simplify not only vaccine administration but also manufacturing and regulatory approval compared with that of multiple disparate vaccines.Citation24–26 Finally, the rLVS vaccine can be administered by multiple routes including intranasally, intradermally, subcutaneously, intramuscularly, and even orally.Citation24–27 Based upon the current lack of an approved tularemia vaccine in the U.S., consideration should be given to advancing the rLVS ΔcapB/iglABC vaccine to further studies in a nonhuman primate model of tularemia challenge to confirm protection.Citation63–65
Supplemental Material
Download PDF (930.1 KB)Acknowledgments
We acknowledge the assistance of Mr. William Discher (USAMRIID) for his assistance with the generating the histopathology figures.
The following reagent was obtained through BEI Resources, NIAID, NIH. Francisella tularensis subsp. tularensis Schu S4 Submaster Cell Bank, NR-10492.
Animal research at the United States Army of Medical Research Institute of Infectious Diseases (USAMRIID) was conducted and approved under an Institutional Animal Care and Use Committee (USAMRIID IACUC) in compliance with the Animal Welfare Act, Public Health Service Policy, and other federal statutes and regulations relating to animals and experiments involving animals. The facility where this research was conducted is accredited by the Association for Assessment and Accreditation of Laboratory Animal Care, International and adheres to principles stated in the Guide for the Care and Use of Laboratory Animals, National Research Council, 2011.
Opinions, interpretations, conclusions, and recommendations are those of the authors and are not necessarily endorsed by the U.S. Army.
USAMRIID is compliant with all federal and Department of Defense regulations pertaining to the use of Select Agents.s
Disclosure statement
No potential conflict of interest was reported by the author(s).
Supplementary data
Supplemental data for this article can be accessed on the publisher’s website at https://doi.org/10.1080/21645515.2023.2277083.
Additional information
Funding
References
- Saslaw S, Eigelsbach HT, Prior JA, Wilson HE, Carhart S. Tularemia vaccine study. II. Respiratory challenge. Arch Intern Med. 1961;107(5):702–14. doi:10.1001/archinte.1961.03620050068007.
- Dennis DT, Inglesby TV, Henderson DA, Bartlett JG, Ascher MS, Eitzen E, Fine AD, Friedlander AM, Hauer J, Layton M, et al. Tularemia as a biological weapon: medical and public health management. JAMA. 2001;285(21):2763–73. [ pii]. doi:10.1001/jama.285.21.2763.
- Jellison WL. Tularemia in Montana. Mont Wildl. 1971:5–24. PMID: 11614973.
- Sjostedt A. Tularemia: history, epidemiology, pathogen physiology, and clinical manifestations. Ann N Y Acad Sci. 2007;1105(1):1–29. doi:10.1196/annals.1409.009.
- Ellis J, Oyston PC, Green M, Titball RW. Tularemia. Clin Microbiol Rev. 2002;15(4):631–46. doi:10.1128/CMR.15.4.631-646.2002.
- Jellison WL. Tularemia; geographical distribution of deer fly fever and the biting fly, Chrysops discalis Williston. Public Health Rep. 1950;65(41):1321–9. doi:10.2307/4587498.
- Markowitz LE, et al. Tick-borne tularemia. An outbreak of lymphadenopathy in children. JAMA. 1985;254(20):2922–5. doi:10.1001/jama.1985.03360200074030.
- Goethert HK, Shani I, Telford SR III. Genotypic diversity of Francisella tularensis infecting Dermacentor variabilis ticks on martha’s vineyard, Massachusetts. J Clin Microbiol. 2004;42(11):4968–73. doi:10.1128/JCM.42.11.4968-4973.2004.
- Kaufmann AF, Meltzer MI, Schmid GP. The economic impact of a bioterrorist attack: are prevention and postattack intervention programs justifiable? Emerg Infect Dis. 1997;3(2):83–94. doi:10.3201/eid0302.970201.
- World Health Organization. Health aspects of chemical and biological weapons. Geneva, Switzerland: World Health Organization; 1970. p. 105–7.
- Franz DR, Jahrling PB, McClain DJ, Hoover DL, Russell Byrne W, Pavlin JA, Christopher GW, Cieslak TJ, Friedlander AM, et al. Clinical recognition and management of patients exposed to biological warfare agents. JAMA. 1997;278(5):399–411. doi:10.1001/jama.1997.03550050061035.
- Eigelsbach HT, Downs CM. Prophylactic effectiveness of live and killed tularemia vaccines. I. Production of vaccine and evaluation in the white mouse and guinea pig. J Immunol. 1961;87(4):415–25. doi:10.4049/jimmunol.87.4.415.
- Tigertt WD. Soviet viable Pasteurella tularensis vaccines a review of selected articles. Bacteriol Rev. 1962;26(3):354–73. doi:10.1128/br.26.3.354-373.1962.
- McCrumb FR. Aerosol infection of man with Pasteurella tularensis. Bacteriol Rev. 1961;25(3):262–7. doi:10.1128/br.25.3.262-267.1961.
- Eigelsbach HT, Tulis JJ, Overholt EL, Griffith WR. Aerogenic immunization of the monkey and guinea pig with live tularemia vaccine. Proc Soc Exp Biol Med. 1961;108(3):732–4. doi:10.3181/00379727-108-27049.
- Conlan JW. Vaccines against Francisella tularensis–past, present and future. Expert Rev Vaccines. 2004;3(3):307–14. [ pii]. doi:10.1586/14760584.3.3.307.
- Eigelsbach HT, Braun W, Herring RD. Studies on the variation of Bacterium tularense. J Bacteriol. 1951;61(5):557–69. doi:10.1128/jb.61.5.557-569.1951.
- Saslaw S, Eigelsbach HT, Wilson HE, Prior JA, Carhart S. Tularemia vaccine study. I. Intracutaneous challenge. Arch Intern Med. 1961;107(5):689–701. doi:10.1001/archinte.1961.03620050055006.
- Bachert BA, Richardson JB, Mlynek KD, Klimko CP, Toothman RG, Fetterer DP, Luquette AE, Chase K, Storrs JL, Rogers AK, et al. Development, phenotypic characterization and genomic analysis of a Francisella tularensis panel for tularemia vaccine testing. Front Microbiol. 2021;12. doi:10.3389/fmicb.2021.725776.
- Twine SM, Shen H, Kelly JF, Chen W, Sjöstedt A, Conlan JW. Virulence comparison in mice of distinct isolates of type A Francisella tularensis. Microb Pathog. 2006;40(3):133–8. [ pii]. doi:10.1016/j.micpath.2005.12.004.
- Farlow J, Wagner DM, Dukerich M, Stanley M, Chu M, Kubota K, Petersen J, Keim P. Francisella tularensis in the United States. Emerg Infect Dis. 2005;11(12):1835–41. doi:10.3201/eid1112.050728.
- Kugeler KJ, Mead P, Janusz A, Staples J, Kubota K, Chalcraft L, Petersen J. Molecular epidemiology of Francisella tularensis in the United States. Clin Infect Dis. 2009;48(7):863–70. doi:10.1086/597261.
- Birdsell DN, Johansson A, Öhrman C, Kaufman E, Molins C, Pearson T, Gyuranecz M, Naumann A, Vogler AJ, Myrtennäs K, et al. Francisella tularensis subsp. tularensis group A.I, United States. Emerg Infect Dis. 2014;20(5):861–5. doi:10.3201/eid2005.131559.
- Jia Q, Bowen R, Dillon BJ, Masleša-Galić S, Chang BT, Kaidi AC, Horwitz MA. Single vector platform vaccine protects against lethal respiratory challenge with Tier 1 select agents of anthrax, plague, and tularemia. Sci Rep. 2018;8(1):7009. doi:10.1038/s41598-018-24581-y.
- Jia Q, Bielefeldt-Ohmann H, Maison RM, Hartwig A, Masleša-Galić S, Bowen RA, Horwitz MA. Oral Administration of universal bacterium-vectored nucleocapsid-expressing COVID-19 vaccine is efficacious in hamsters. Microbiol Spectr. 2023;e0503522(2). doi:10.1128/spectrum.05035-22.
- Jia Q, Bielefeldt-Ohmann H, Maison RM, Masleša-Galić S, Cooper SK, Bowen RA, Horwitz MA. Replicating bacterium-vectored vaccine expressing SARS-CoV-2 Membrane and Nucleocapsid proteins protects against severe COVID-19-like disease in hamsters. NPJ Vaccines. 2021;6(1):47. doi:10.1038/s41541-021-00321-8.
- Jia Q, Bowen R, Lee B-Y, Dillon BJ, Masleša-Galić S, Horwitz MA. Francisella tularensis live vaccine strain deficient in capB and overexpressing the fusion protein of IglA, IglB, and IglC from the bfr promoter induces improved protection against F. tularensis respiratory challenge. Vaccine. 2016;34(41):4969–78. doi:10.1016/j.vaccine.2016.08.041.
- Roy CJ, Hale M, Hartings JM, Pitt L, Duniho S. Impact of inhalation exposure modality and particle size on the respiratory deposition of ricin in BALB/c mice. Inhal Toxicol. 2003;15(6):619–38. doi:10.1080/08958370390205092.
- Dabisch P, Bower K, Dorsey B, Wronka L. Recovery efficiencies for Burkholderia thailandensis from various aerosol sampling media. Front Cell Inf Microbio. 2012;2:78. doi:10.3389/fcimb.2012.00078.
- Dabisch P, Yeager J, Kline J, Klinedinst K, Welsch A, Pitt ML. Comparison of the efficiency of sampling devices for aerosolized Burkholderia pseudomallei. Inhal Toxicol. 2012;24(5):247–54. doi:10.3109/08958378.2012.666682.
- Glynn A, Roy CJ, Powell BS, Adamovicz JJ, Freytag LC, Clements JD. Protection against aerosolized Yersinia pestis challenge following homologous and heterologous prime-boost with recombinant plague antigens. Infect Immun. 2005;73(8):5256–61. doi:10.1128/IAI.73.8.5256-5261.2005.
- Hutt JA, Lovchik JA, Dekonenko A, Hahn AC, Wu TH. The Natural History of Pneumonic Tularemia in Female Fischer 344 Rats after Inhalational Exposure to Aerosolized Francisella tularensis Subspecies tularensis Strain SCHU S4. Am J Pathol. 2017;187(2):252–67. doi:10.1016/j.ajpath.2016.09.021.
- Ray HJ, Chu P, Wu TH, Lyons CR, Murthy AK, Guentzel MN, Klose KE, Arulanandam BP. The Fischer 344 rat reflects human susceptibility to Francisella pulmonary challenge and provides a new platform for virulence and protection studies. PLoS One. 2010;5(4):e9952. doi:10.1371/journal.pone.0009952.
- Binder J, Graser E, Hancock WW, Wasowska B, Sayegh MH, Volk H-D, Kupiec-Weglinski JW. Downregulation of intragraft IFN-γ expression correlates with increased IgG1 alloantibody response following intrathymic immunomodulation of sensitized rat recipients1,2. Transplantation. 1995;60(12):1516–24. doi:10.1097/00007890-199560120-00025.
- Gracie JA, Bradley JA. Interleukin-12 induces interferon-γ-dependent switching of IgG alloantibody subclass. Eur J Immunol. 1996;26(6):1217–21. doi:10.1002/eji.1830260605.
- Drabner B, Guzman CA. Elicitation of predictable immune responses by using live bacterial vectors. Biomol Eng. 2001;17(3):75–82. doi:10.1016/S1389-0344(00)00072-1.
- Salomonsson E, Kuoppa K, Forslund A-L, Zingmark C, Golovliov I, Sjostedt AJ, Noppa L, Forsberg A. Reintroduction of two deleted virulence loci restores full virulence to the live vaccine strain of Francisella tularensis. Infect Immun. 2009;77(8):3424–31. doi:10.1128/IAI.00196-09.
- Rohmer L, Fong C, Abmayr S, Wasnick M, Larson Freeman T, Radey M, Guina T, Svensson K, Hayden HS, Jacobs M, et al. Comparison of Francisella tularensis genomes reveals evolutionary events associated with the emergence of human pathogenic strains. Genome Biol. 2007;8(6):R102. doi:10.1186/gb-2007-8-6-r102.
- Jia Q, Lee B-Y, Bowen R, Dillon BJ, Som SM, Horwitz MA. A Francisella tularensis live vaccine strain (LVS) mutant with a deletion in capB, encoding a putative capsular biosynthesis protein, is significantly more attenuated than LVS yet induces potent protective immunity in mice against F. tularensis challenge. Infect Immun. 2010;78(10):4341–55. doi:10.1128/IAI.00192-10.
- Svensson K, Larsson P, Johansson D, Bystrom M, Forsman M, Johansson A. Evolution of subspecies of Francisella tularensis. J Bacteriol. 2005;187(11):3903–8. doi:10.1128/JB.187.11.3903-3908.2005.
- Raymond CR, Conlan JW. Differential susceptibility of Sprague–Dawley and Fischer 344 rats to infection by Francisella tularensis. Microb Pathog. 2009;46(4):231–4. [ pii]. doi:10.1016/j.micpath.2009.01.002.
- Jemski JV. Respiratory tularemia: comparison of selected routes of vaccination in Fischer 344 rats. Infect Immun. 1981;34(3):766–72. doi:10.1128/iai.34.3.766-772.1981.
- De Pascalis R, Hahn A, Brook HM, Ryden P, Donart N, Mittereder L, Frey B, Wu TH, Elkins KL, et al. A panel of correlates predicts vaccine-induced protection of rats against respiratory challenge with virulent Francisella tularensis. PLoS One. 2018;13(5):e0198140. doi:10.1371/journal.pone.0198140.
- Wu TH, Zsemlye JL, Statom GL, Hutt JA, Schrader RM, Scrymgeour AA, Lyons CR. Vaccination of Fischer 344 rats against pulmonary infections by Francisella tularensis type a strains. Vaccine. 2009;27(34):4684–93. doi:10.1016/j.vaccine.2009.05.060.
- Mara-Koosham G, Hutt JA, Lyons CR, Wu TH, Morrison RP. Antibodies contribute to effective vaccination against respiratory infection by type a Francisella tularensis strains. Infect Immun. 2011;79(4):1770–8. doi:10.1128/IAI.00605-10.
- Kirimanjeswara GS, Olmos S, Bakshi CS, Metzger DW. Humoral and cell-mediated immunity to the intracellular pathogen Francisella tularensis. Immunol Rev. 2008;225(1):244–55. doi:10.1111/j.1600-065X.2008.00689.x.
- Pasetti MF, Cuberos L, Horn TL, Shearer JD, Matthews SJ, House RV, Sztein MB. An improved Francisella tularensis live vaccine strain (LVS) is well tolerated and highly immunogenic when administered to rabbits in escalating doses using various immunization routes. Vaccine. 2008;26(14):1773–85. doi:10.1016/j.vaccine.2008.01.005.
- Mlynek KD, Lopez CT, Fetterer DP, Williams JA, Bozue JA. Phase variation of LPS and capsule is responsible for stochastic biofilm formation in Francisella tularensis. Front Cell Infect Microbiol. 2022;11:11. doi:10.3389/fcimb.2021.808550.
- Waag DM, Sandstrom G, England MJ, Williams JC. Immunogenicity of a new lot of Francisella tularensis live vaccine strain in human volunteers. FEMS Immunol Med Microbiol. 1996;13(3):205–9. doi:10.1111/j.1574-695X.1996.tb00238.x.
- Oyston PC. Francisella tularensis vaccines. Vaccine. 2009;27(Suppl 4):D48–51. doi:10.1016/j.vaccine.2009.07.090.
- Soni S, Ernst RK, Muszyński A, Mohapatra NP, Perry MB, Vinogradov E, Carlson RW, Gunn JS. Francisella tularensis blue–gray phase variation involves structural modifications of lipopolysaccharide O-Antigen, core and lipid a and affects intramacrophage survival and vaccine efficacy. Front Microbio. 2010;1:129. doi:10.3389/fmicb.2010.00129.
- Pechous RD, McCarthy TR, Mohapatra NP, Soni S, Penoske RM, Salzman NH, Frank DW, Gunn JS, Zahrt TC, et al. A Francisella tularensis Schu S4 purine auxotroph is highly attenuated in mice but offers limited protection against homologous intranasal challenge. PLoS One. 2008;3(6):e2487. doi:10.1371/journal.pone.0002487.
- Qin A, Scott DW, Thompson JA, Mann BJ. Identification of an essential Francisella tularensis subsp. tularensis virulence factor. Infect Immun. 2009;77(1):152–61. [ pii]. doi:10.1128/IAI.01113-08.
- Golovliov I, Twine SM, Shen H, Sjostedt A, Conlan W, Kaufmann GF. A ΔclpB mutant of Francisella tularensis subspecies holarctica strain, FSC200, is a more effective live vaccine than F. tularensis LVS in a mouse respiratory challenge model of tularemia. PLoS One. 2013;8(11):e78671. doi:10.1371/journal.pone.0078671.
- Straskova A, Cerveny L, Spidlova P, Dankova V, Belcic D, Santic M, Stulik J. Deletion of IglH in virulent Francisella tularensis subsp. holarctica FSC200 strain results in attenuation and provides protection against the challenge with the parental strain. Microbes Infection/Institut Pasteur. 2012;14(2):177–87. doi:10.1016/j.micinf.2011.08.017.
- Bakshi CS, Malik M, Mahawar M, Kirimanjeswara GS, Hazlett KRO, Palmer LE, Furie MB, Singh R, Melendez JA, Sellati TJ, et al. An improved vaccine for prevention of respiratory tularemia caused by Francisella tularensis SchuS4 strain. Vaccine. 2008;26(41):5276–88. doi:10.1016/j.vaccine.2008.07.051.
- Rockx-Brouwer D, Chong A, Wehrly TD, Child R, Crane DD, Celli J, Bosio CM. Low dose vaccination with attenuated Francisella tularensis strain SchuS4 mutants protects against tularemia independent of the route of vaccination. PLoS One. 2012;7(5):e37752. doi:10.1371/journal.pone.0037752.
- Whelan AO, Flick-Smith HC, Homan J, Shen ZT, Carpenter Z, Khoshkenar P, Abraham A, Walker NJ, Levitz SM, Ostroff GR, et al. Protection induced by a Francisella tularensis subunit vaccine delivered by glucan particles. PLoS One. 2018;13(10):e0200213. doi:10.1371/journal.pone.0200213.
- Jia Q, Lee BY, Clemens DL, Bowen RA, Horwitz MA. Recombinant attenuated Listeria monocytogenes vaccine expressing Francisella tularensis IglC induces protection in mice against aerosolized Type A F. tularensis. Vaccine. 2009;27(8):1216–29. doi:10.1016/j.vaccine.2008.12.014.
- O’Malley KJ, Bowling JL, Stinson E, Cole KS, Mann BJ, Namjoshi P, Hazlett KRO, Barry EM, Reed DS, et al. Aerosol prime-boost vaccination provides strong protection in outbred rabbits against virulent type a Francisella tularensis. PLoS One. 2018;13(10):e0205928. doi:10.1371/journal.pone.0205928.
- Chu P, Cunningham AL, Yu J-J, Nguyen JQ, Barker JR, Lyons CR, Wilder J, Valderas M, Sherwood RL, Arulanandam BP, et al. Live attenuated Francisella novicida vaccine protects against Francisella tularensis pulmonary challenge in rats and non-human primates. PLoS Pathog. 2014;10(10):e1004439. doi:10.1371/journal.ppat.1004439.
- De Pascalis R, Frey B, Rice HM, Bhargava V, Wu TH, Peterson RL, Conlan JW, Sjöstedt A, Elkins KL. Working correlates of protection predict SchuS4-derived-vaccine candidates with improved efficacy against an intracellular bacterium, Francisella tularensis. NPJ Vaccines. 2022;7(1):95. doi:10.1038/s41541-022-00506-9.
- Frick OM, Livingston VA, Whitehouse CA, Norris SL, Alves DA, Facemire PR, Reed DS, Nalca A. The natural history of aerosolized Francisella tularensis infection in cynomolgus macaques. Pathogens. 2021;10(5):597. doi:10.3390/pathogens10050597.
- Glynn AR, Alves DA, Frick O, Erwin-Cohen R, Porter A, Norris S, Waag D, Nalca A. Comparison of experimental respiratory tularemia in three nonhuman primate species. Comp Immunol Microbiol Infect Dis. 2015;39:13–24. doi:10.1016/j.cimid.2015.01.003.
- Nelson M, Lever MS, Savage VL, Salguero FJ, Pearce PC, Stevens DJ, Simpson AJH. Establishment of lethal inhalational infection with Francisella tularensis (tularaemia) in the common marmoset (Callithrix jacchus). Int J Experimental Path. 2009;90(2):109–18. doi:10.1111/j.1365-2613.2008.00631.x.