ABSTRACT
The influenza viruses cause seasonal respiratory illness that affect millions of people globally every year. Prophylactic vaccines are the recommended method to prevent the breakout of influenza epidemics. One of the current commercial influenza vaccines consists of inactivated viruses that are selected months prior to the start of a new influenza season. In many seasons, the vaccine effectiveness (VE) of these vaccines can be relatively low. Therefore, there is an urgent need to develop an improved, more universal influenza vaccine (UIV) that can provide broad protection against various drifted strains in all age groups. To meet this need, the computationally optimized broadly reactive antigen (COBRA) methodology was developed to design a hemagglutinin (HA) molecule as a new influenza vaccine. In this study, COBRA HA-based inactivated influenza viruses (IIV) expressing the COBRA HA from H1 or H3 influenza viruses were developed and characterized for the elicitation of immediate and long-term protective immunity in both immunologically naïve or influenza pre-immune animal models. These results were compared to animals vaccinated with IIV vaccines expressing wild-type H1 or H3 HA proteins (WT-IIV). The COBRA-IIV elicited long-lasting broadly reactive antibodies that had hemagglutination-inhibition (HAI) activity against drifted influenza variants.
Introduction
Every year, the seasonal influenza viruses are responsible for ~3–5 million worldwide cases of severe illness and result in up to 650,000 deaths.Citation1 For the subtype influenza A virus (IAV), the H2N2 subtype influenza viruses circulated from 1957 to 1968 and the H1N1 and H3N2 influenza viruses are currently circulating in people. Both H1N1 and H3N2 cause seasonal epidemics worldwide, but in general, influenza seasons in which H3N2 viruses are prevalent tend to be more severe with a greater number of hospitalizations and deaths.Citation2 The World Health Organization (WHO) recommends that vaccination is the most effective way to prevent infection and severe outcomes caused by influenza viruses. The most commonly used influenza virus vaccine is composed of wild-type (WT) inactivated influenza viruses (IIV). Typically, WT influenza strains are chosen annually for inclusion in the seasonal vaccine either the elicitation of narrow or strain-specific immune responses.Citation3 Selected strains are amplified in embryonic chicken eggs and then inactivated with formaldehyde (FA) or beta-propiolactone (BPL).Citation4 The IIV refers to both the whole inactivated influenza virus (WIV) vaccine or the split inactivated influenza virus (SIV) vaccine. The WIV vaccine was first developed for human use in the 1940s, but due to its high reactogenicity, it is no longer used in most regions of the world.Citation5 The SIV is manufactured by adding detergents to split the WIV and this vaccine replaced the WIV as the most commonly administered commercial influenza virus vaccine in the U.S.Citation6 The current influenza vaccine includes four representative influenza strains, which are updated annually. However, due to extensive viral genetic and antigenic evolution, the vaccine effectiveness (VE) of current SIV vaccine is limited,Citation7 with an estimated VE of ~60% for all populations but can be lower when poorly matched to circulating strains.Citation8
To address the continuous changes in influenza hemagglutinin (HA) and neuraminidase (NA) surface viral glycoproteins and subsequent evasion of elicited immune responses in the host, the next-generation influenza virus vaccines are in development. The goal of these new seasonal influenza vaccine strategies is to elicit durable protective immunity against influenza A and B strains for all populations and all age groups.Citation9 HA is the most abundant and antigenic glycoprotein of the influenza virus. It binds to the sialic acid on the host cell surfaces to facilitate the endocytosis of virions.Citation10 Therefore, the anti-HA antibodies that can efficiently block virus entry are the focus of many vaccine designs. One of these approaches uses the computationally optimized broadly reactive antigen (COBRA) methodology to design the antigens by multiple rounds of consensus building based on thousands of influenza strains circulating in a time period.Citation11 The COBRA candidates were tested in vivo for screening down to the one with the best effectiveness in eliciting broadly reactive antibodies. Previously, the COBRA antigens Y2 (H1) and J4 (H3) were proven to overcome the diversity of epitopes in the HA and, when used as an immunogen, elicit antibodies against various drifted strains.Citation11–16 In these studies, COBRA HA immunogens have been delivered to the host immune system intramuscularly as recombinant HA (rHA),Citation15 in virus-like particles (VLP).Citation11 The reduced immunogenicity of rHA and VLP requires the addition of an adjuvant or multiple doses to elicit expected immune responses, which increases the manufacturing cost and safety concerns. With the development of reverse genetic technology, combinations of hemagglutinin and neuraminidase can be mixed to generate recombinant viruses and then inactivated as a vaccine.Citation17
IIV consisting of all viral antigens shows higher antigenicity than the platforms tested before, such as rHA and VLP, and is a more promising format for the universal influenza vaccine. Therefore, this study designed the bivalent IIV vaccines expressing the H1 COBRA HA or the H3 COBRA HA with or without adjuvant and tested their effectiveness in naïve and pre-immune mouse models. COBRA H1 candidate, Y2, was based on circulating strains from 2016 to 2018,Citation15 and the H3 COBRA HA candidate, J4, was designed using circulating strains from 2013 to 2016).Citation12 Most people are not immunologically naïve to influenza due to previous infections and/or vaccinations.Citation18,Citation19 Therefore, the COBRA-IIV vaccines were tested in mice with preexisting immunity to historical influenza viruses or immunologically naïve animals. Overall, these COBRA-IIV vaccines elicited long-lasting protective immune responses that recognized a broader number of H1N1 and H3N2 contemporary viruses than IIV vaccines expressing wild-type HA proteins.
Materials and methods
Recombinant influenza virus rescue
Recombinant influenza viruses expressing COBRA HA antigens were recused using the eight-plasmid reverse genetic system.Citation20 Briefly, the A/Puerto Rico/8/1934 (H1N1) (PR/8) was used as the template with the genes encoding for the WT HA replaced with the gene encoding COBRA HA sequence for Y2 or J4. The cDNA representing one of the influenza gene segments was cloned into the pHW-2000 template resulting in eight expression plasmids: pHW2000-PR8 PB2, pHW2000-PR8 PB1, pHW2000-PR8 PA, pHW2000-PR8 NP, pHW2000-PR8 M, pHW2000-PR8 NS, pHW2000-PR8 NA, and pHW2000-HA Y2 or pHW2000-HA J4. Madin-Darby canine kidney (MDCK) cells and 293 cells were mixed and plated before each transfection and allowed to grow to 80–90% confluency. Each plasmid (1 μg) was added to the cells and the cells were incubated with OptiMEM at 33°C/5%CO2 overnight for ~16 h. Cell culture media was replaced by OptiMEM containing TPCK-Trypsin (2 μg/mL final concentration) and incubated at 33°C/5%CO2 overnight or ~16 h. Cells daily until the cytopathogenic effect (CPE) was observed for most cells and then HA titer of the cell supernatant was determined. The rescued viruses were centrifuged at 1500rpm/5 min to clarify and stored in a −80 freezer. MDCK (0.01 MOI) plus TPCK-Trypsin (1 μg/ml) were infected to amplify viruses. The infected cells were incubated at 33°C/5%CO2, and obvious CPE was observed after 3 days, which confirmed their infectivity.
Vaccine preparation
To inactivate influenza viruses, one part of 0.5 M disodium phosphate (DSP) was added to 38 parts of the virus solution, and 1 part of 2% v/v BPL aqueous solution was added to 38 parts of the virus/DSP mixture drop wisely. The mixture was incubated on ice for 30 min and then in a 37°C water bath for 2 h with occasional mixing. Afterward, the pH was adjusted to 7.3–7.4. The inactivated virus was purified by ultracentrifugation with 30% (v/v) sucrose cushion at 25,000 rpm, 4°C for 2 h. Half of the whole inactivated virus (WIV) was aliquoted and stored in a −80 freezer, and the other half was used to manufacture the split-inactivated virus (SIV) vaccine. Triton X100 at 1% (v/v) final concentration was added to the WIV solution, and then the mixture was incubated at RT for 1 h. Afterwards, 0.1 g BioBeads per mL of the mixture was added to remove the extra Triton X100 for safety. The mixture was put in the shaker with mild shaking at 4°C overnight, and then the liquid part was collected. The plaque assay was employed to confirm the inactivation and as expected, while the control virus was infectious, the inactivated and split viruses failed to generate plaques (Suppl Figure S1a). We next used a hemagglutination assay to ensure that the viral HA binding activities to sialic acid-binding were maintained after inactivation. There was no significant difference in HA titer before or after the inactivation and splitting of the recombinant COBRA viruses (Suppl Figure S1b). The HA content of the COBRA-IIVs and WT-WIVs was further confirmed and quantified using anti-H1 or anti-H3 monoclonal antibody binding on an SDS-PAGE and western blot (Suppl Figure S1c). The molecular weight of the WIV is larger than the SIV, confirming the efficacy of our splitting methodology. To broaden the protection of our COBRA-WIVs, we designed them in the bivalent format with both Y2 and J4. For wild-type comparators, the WT A/Brisbane/02/2018 H1N1 virus and the A/Kansas/14/2017 H3N2 influenza virus were inactivated by the same methodology to prepare the WT-IIV vaccines.
Viruses
All viruses were obtained through the Influenza Reagents Resource (IRR), BEI Resources, or the Centers for Disease Control (CDC) or were provided by Virapur (San Diego, CA). Viral stocks were amplified using the World Health Organization (WHO) protocol.Citation21 Viruses used in this study include the H1N1 influenza viruses: A/Singapore/6/1986 (Sing/86), A/Brisbane/02/2018 (BS/18), A/Guangdong-Maonan/SWL1536/2019 (GD/19), and A/Victoria/2570/2019 (Vic/19). The H3N2 influenza viruses include: A/Panama/2007/1999 (Pan/99), A/Switzerland/9715293/2013-mouse adapted (SW/13), A/Hong Kong/4801/2014 (HK/14), A/Singapore/IFNIMH-16-0019/2016 (Sing/16), A/Kansas/14/2017 (Kan/17), A/South Australia/34/2019 (SA/19), A/Hong Kong/2671/2019 (HK/19), and A/Tasmania/503/2020 (TAS/20). For FRA experiments, serum samples were tested against H1N1 and H3N2 viruses, including CA/09, BS/18, GD/19, SW/13, KS/17, HK/19, SA/19, TAS/20, and A/DARWIN/9/2021 (Dar/21). A comparison between the WT HA sequences and COBRA HA sequences is shown in .
Table 1. Genetic similarity between full-length WT HA and COBRA HA Footnote[a].
Vaccination and challenge
COBRA-IIV or WT-IIV vaccines were formulated the regular dose (1 μg/HA/50 μL) or a reduced dose (0.1 μg/HA/50 μL) and mixed with AddaVax adjuvant (InvivoGen, San Diego, CA, USA) (25 μL) and then phosphate buffered saline (PBS) (Gibco, Waltham, MA, USA) was used to bring the final volume of 50 μL. Similar vaccine doses were used without adjuvant, and PBS was used to bring the total volume to 50 μL.
The DBA/2J mouse strain is employed in this study because of its high susceptibility to human influenza strainsCitation22 Female DBA/2J mice (6–8 weeks old) were vaccinated intramuscularly with each vaccine and then boosted 28 days later. Blood samples were collected 2 weeks after each vaccination and centrifuged at 6,000 rpm for 5 min to collect the serum, which were aliquoted and then stored in a −20C freezer for analysis later. For the longevity study, extra serum samples were collected at weeks 10, 14, 18, and 20 to monitor the antibody dynamics. At either week 8 or week 22, mice were infected intranasally with either the H1N1 BS/18 (3.6 × 10^6PFU/50 μL) virus or the H3N2 SW/13 (3.36 × 10^4PFU/50 μL) in PBS solution. All the mice were monitored for 14 days after the infection. Lung samples (n = 3/group/virus) were collected on 3DPI for plaque assay.
For pre-infection, one historical H1N1 strain, A/Singapore/6/1986 (Sing/86), and one historical H3N2 strain, A/Panama/2007/1999 (Pan/99), were used. A mixture of Sing/86 and Pan/99 at the final concentration of 5 × 10^5PFU/virus/50 μL in PBS solution was administrated intranasally to each mouse under anesthesia 28 days before the primary vaccination. Blood samples were collected 14 days after the pre-infection to confirm the preexisting antibodies.
Mice were humanely euthanized once they lost 25% of their original body weight or reached to clinical score of 3. At the end of the study, all mice were humanely euthanized. All procedures were performed in accordance with the Guide for the Care and Use of Laboratory Animals, the Animal Welfare Act, and Biosafety in Microbiological and Biomedical Laboratories.
Hemagglutination inhibition (HAI) assay
The HAI assay can detect Abs that bind to the HA head, which inhibits the binding of viral HA to sialic acid on the red blood cells. This causes hemagglutination of the red blood cells and can be read easily. All serum samples were first treated with a receptor-destroying enzyme (RDE; Denka Seiken, Co., Japan) to inactivate the nonspecific inhibitors. The serum samples were mixed with the RDE at a 1:3 ratio and incubated at 37°C overnight. Then, the mixture was put in a 56°C water bath for 45 min to inactivate the RDE. PBS was added after it cooled down to room temperature (RT), and the final ratio of serum: RDE: PBS is 1:3:6. The RDE-treated serum samples were diluted by PBS in a 2-fold series dilution in a v-bottom 96-well plate. For H1N1 viruses, turkey red blood cells (TRBC) (Lampire Biologicals, Pipersville, PA, USA) were diluted in PBS to the final concentration of 0.8%. Each virus was adjusted to the concentration of eight hemagglutination units (HAU)/50 μL, and an equal volume of prepared virus was added to the serum plate. The serum was incubated with the virus for 20 min, and then 0.8% TRBC was added to every well. Plates were gently tapped to mix them well and incubated at RT for 30 min. For H3N2 viruses, guinea pig red blood cells (GPRBC) (Lampire Biologicals, Pipersville, PA) were diluted in PBS to a concentration of 0.75%. Each virus was adjusted to the concentration of 8HAU/50 μL with the presence of 20 nM Oseltamivir, and an equal volume of prepared virus was added to the serum plate. The serum was incubated with the virus for 30 min, and then 0.75% GPRBC was added to every well. Plates were gently tapped to mix them well and incubated at RT for 1 h. The HAI titers were determined by the reciprocal dilution of the last well where non-hemagglutination was observed. According to the WHO instruction, HAI titers >1:40 are associated with a 50% reduction in the risk of influenza infection, and are considered HAI positive in this study.Citation23
Focus reduction assay (FRA)
The FRA was used to detect the comprehensive neutralizing antibody levels in this study. The whole procedure was described previously.Citation24 One day before the experiment, 100 μL/well of MDCK-SIAT1 cells (Sigma, St. Louis, MO) at 2.5 × 10^5 to 3 × 10^5 cells/mL was added to 96-well plates. Plates were incubated at 37°C overnight to form a 90–100% confluent monolayer. The next day, RDE-treated serum samples were 2-fold diluted with the starting 1:20 dilution, and the virus was adjusted to a concentration of 1.2 × 10^4 focus-forming units (FFU)/mL in virus growth media containing 1 μg/mL TPCK-treated trypsin (VGM-T). The monolayer was rinsed with 0.01 M PBS to remove the cell culture media. 50 μL/well of serially diluted serum samples and 50 μL/well of prepared virus solution were added to plates. Plates were incubated at 37°C and 5% CO2 for 2 h to let the antibody neutralize the virus and let the unnaturalized virus attach to the MDCK-SIAT1 cells. Then, 100 μL/well of overlay containing 1.2% Avicel (Type RC581 NF; FMC Health and Nutrition, Philadelphia, PA) and 1 μg/mL TPCK-treated trypsin were added to the plates to limit the spreading of the virus. Plates were incubated overnight at 37°C and 5% CO2. Then, the overlay was discarded, and the plates were washed twice with PBS. 100 μL/well of ice-cold 4% formalin in PBS was added and incubated at 4°C for 30 min to fix the cells. Later, the formalin solution was discarded, and after PBS wash, 100 μL/well of permeabilization solution was added and incubated at RT for 20 min. After this step, the following experiment is as enzyme-limited immunosorbent assay (ELISA). The plates were incubated at RT for 1 h with the primary antibody mouse anti-A/NP mAb pool (IRR, Manassas, VA) diluted 1:2000 in ELISA buffer. Then, after wash x3, the plates were incubated at RT for 1 h with the secondary antibody goat anti-mouse peroxidase-labeled IgG (KPL 474–1802, 1 mg/mL; Sera Care, Inc., Milford, MA) diluted 1:2,000 in ELISA buffer. Plates were washed x3 again and incubated at RT for 10 min with True TrueBlue substrate (Sera Care, Inc., Milford, MA) containing 0.03% H2O2 to visualize the infectious foci. The plates were washed five times with tape water to stop the reaction and put in the hood to dry. Plates were read using a BioSpot analyzer with ImmunoCapture 6.4.87 software (CTL, Shaker Heights, OH). The FRA titer was determined as the reciprocal of the highest dilution of serum that can reduce 50% infectious foci.
Enzyme-linked immunosorbent assay (ELISA)
ELISA was conducted to detect the total IgG and IgG subtypes specific to different antigens as previously described.Citation15 Briefly, the Immulon 4HBX plates (Thermo Fisher Scientific, Waltham, MA, USA) were coated with the coating solution containing BS/18 rHA, SW/13 rHA, cH6/1, or cH7/3 with the concentration of 1 μg/mL. Assay plates were incubated with the coating solution in a humified chamber at 4°C overnight. The next day, those plates were blocked by adding 200 μL/well blocking buffer and then incubated at 37°C for 90 min. Meanwhile, serum samples were 3-fold serial diluted starting from 1:500 in the blocking buffer. After the blocking, the blocking buffer in assay plates was discarded, and 100 μL/well of the diluted samples was added to the plates. After incubation at 37°C for 1 h, the 1:2000 diluted goat anti-mouse IgG (Southern Biotech, Birmingham, AL, USA) secondary antibody was added to the plates (100 μL/well), and incubated at 37°C for 1 h. Finally, 50 μL of ABTS substrate (VWR Corporation) was added into each well and incubated at 37°C for 15 min. The reaction was terminated by adding 50 μL of 1% SDS into each well. The O.D. values (OD 414) were measured by a spectrophotometer (PowerWave XS, BioTek) at the wavelength of 414 nm.
Plaque assay
Plaque assay was conducted following the protocol described previously.Citation12–25Citation26Citation27 Briefly, 2 days before the assay, MDCK cells were seeded at 2.5 × 10^5 cells/well in 6-well plates in cell growth media. Start the assay when the cell confluence is above 90%. Lung samples were homogenized and centrifuged at 400 rpm for 10 min to remove cell debris. Supernatants were collected and 10-fold serially diluted from 10^0 to 10^-5 with wash buffer. Diluted samples were added to each well in 6-well plates in sequence and incubated at 37°C for 1 h with gentle rocking every 15 min. Media was removed, and plates were washed twice with wash buffer to remove unattached viruses. Two-milliliter 0.8% agarose (Cambrex, East Rutherford, NJ, USA) was added to each well. Plates were incubated at 37°C with 5% CO2 for 72 h. Then, the agarose overlay was removed and discarded. The cell monolayer was fixed with 10% formalin solution and stained with crystal violet. Then, wash the plates throughout with tape water and let them air dry. The number of plaques of each plate was counted and calculated in the format of PFU per milliliter for each virus.
FluoroSpot assay
Spleen samples were collected on day 7 after vaccination and homogenized. Cells were counted and stored in liquid nitrogen for later use. The procedure of FluoroSpot assay is describedCitation28 previously. Assay plates, Abs, and all reagents were included in the mouse IgM/IgG1/IgG2a/IgG2b Four-Color ImmunoSpot® kit (CTL, Shaker Heights, OH, USA), and all procedures followed the instructions provided by the manufacturer. For detecting the total Ig, the assay plates were activated by 70% (v/v) ethanol and coated with anti-Igκ/λ capture Abs. For detecting the Ag-specific ASC, the activated assay plates were coated with COBRA Y2 or COBRA J4 rHA proteins. Plates were incubated in 4°C in a humified chamber overnight. The next day, cell samples were thawed and 2-fold serially diluted in triplicates with the starting concentration of 1 × 10^5 (for total Ig) or 3 × 10^5 (for Ag-specific ASC) live cells/well in 96-well tissue culture plates (Corning, Corning, NY, USA). After one wash with PBS, assay plates were blocked by B cell media (BCM) at RT for 1 h. Then, the diluted samples were transferred to assay plates and incubated at 37°C with 5% CO2 for 16 h. After the incubation, the detection Abs with different fluorochrome conjugates were added, followed by the addition of different subtracts to visualize the spot-forming unit (SFU). The assay plates were air-dried before being scanned by the ImmunoSpot® S6 Ultimate Analyzer. Then, the scanned results were counted by the Basic Count mode of CTL ImmunoSpot SC Studio (Version 1.6.2, Shaker Heights, OH, USA).
Statistical analysis
Differences in weight loss, dynamic antibody titers against challenge strains were analyzed by two-way ANOVA. The differences in HAI titer, IgG titers, ASCs and lung viral titers were analyzed by ordinary one-way ANOVA. Statistical significance was defined as p-value of .05. Statistical analyses were done using GraphPad Prism software.
Results
COBRA-WIV vaccine elicited better protection than WT-WIV against heterologous strains in naïve mice
To determine the effectiveness of WIV vaccine expressing either COBRA HA or wild-type HA antigens, mice were vaccinated (week 0) and boosted (week 4) with either vaccine without adjuvant, 0.1 μg or 1 μg (). Mock vaccinated mice were administered PBS only. Mice vaccinated with WT-WIV had the highest serum HAI activity against the homologous strains, BS/18 or KS/17 (). COBRA-WIV also elicited antibodies with broadly protective HAI titers against these viruses (>1:40) and detected significantly more antibodies against the heterologous strains SW/13 and HK/14 compared to mice vaccinated with the WT-WIV (). Even mice vaccinated with the lower dose (0.1 μg) of the COBRA-WIV maintained seroprotective HAI activity against CA/09, BS/18, SW/13, and HK/14. In contrast, mice vaccinated with the lower dose of the WT-WIV had antibodies with seroprotective HAI activity against CA/09, BS/18, SW/13, and KS/17 ().
Figure 1. COBRA-WIVs elicited more broadly reactive antibodies than WT-WIV against heterologous strains.
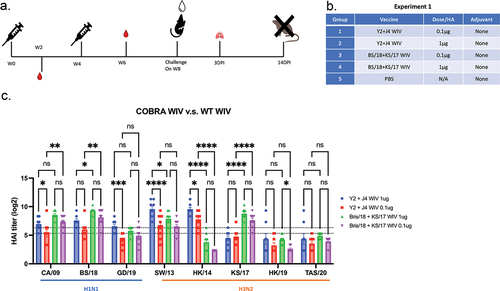
Collected mouse sera was tested using an FRA to determine the overall neutralizing activity of the elicited antibodies by each vaccine (). Mice vaccinated with COBRA-WIV elicited antibodies with 2–5 log2 higher neutralizing activity against heterologous strains (SA/19, HK/19, Dar/21, and GD/19) than sera from mice vaccinated with WT-WIV, regardless of dose, albeit at lower titers. Interestingly, sera collected from mice vaccinated with COBRA-WIV poorly elicited antibodies with HAI activity against KS/17, the same sera had abundant KS/17-specific neutralizing antibodies.
Table 2. COBRA-WIV can elicit more broadly reactive neutralizing antibodies than WT-WIV.
All mice were challenged with the BS/18 influenza virus. Mock vaccinated mice lost ~75% weight following the challenge until reaching the clinical endpoint at 6 days post infection (DPI) (Suppl Figure S2a) with high lung titers (10^6 pfu/g) detected at 3DPI (Suppl Figure S2b). In contrast, mice vaccinated with either COBRA-WIV or WT-WIV, regardless of dose, lose on average 8–13% of their original body weight by day 3–4 post-infection and then slowly recovered to their original body weight after 14 days (Suppl Figure S2a). Viral titers from vaccinated mice were 3–5 logs less than mock vaccinated mice (Suppl Figure S2b).
COBRA-IIV elicited broad and long-lasting antibodies in naïve mice
Next, the split-inactivated virus vaccine expressing COBRA HA antigens was tested for immunogenicity and protection against challenge (). These vaccines were examined for the elicitation of acute immediate and long-lived HAI activity, as well as protection against influenza virus challenge after 4 months post-vaccination (). Mice vaccinated with these SIV vaccines expressing COBRA HA antigens were also compared to mice vaccinated with the COBRA-WIV vaccines (). Mock vaccinated mice or mice vaccinated with AddaVax adjuvant only had no detectable serum HAI activity (). Mice vaccinated with COBRA-WIV elicited antibodies with significantly higher HAI activity than mice vaccinated with COBRA-SIV vaccines against 6 of 10 strains tested (BS/18, GD/19, Vic/19, SW/13, HK/14, and Sing/16). The mean HAI titer elicited in mice vaccinated with COBRA-WIV was slightly higher than the HAI titer elicited in mice vaccinated with COBRA-SIV vaccines. Mice had similar HAI titers following vaccination with either vaccine plus AddaVax for all strains, except for Vic/19, where the COBRA-SIV elicited higher HAI titers than COBRA-WIV (p < .01). HAI activity was maintained against the challenge strains BS/18 and SW/13 over a 20-week period (Suppl Figure S3).
Figure 2. Mouse experimental 2 outline.
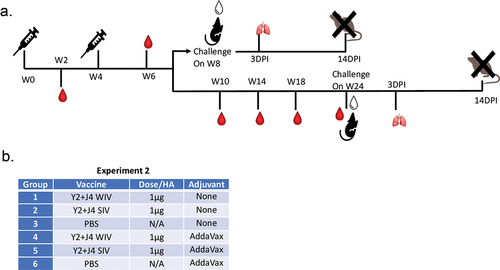
Figure 3. COBRA-IIVs can elicit broadly reactive antibodies in naïve mice.
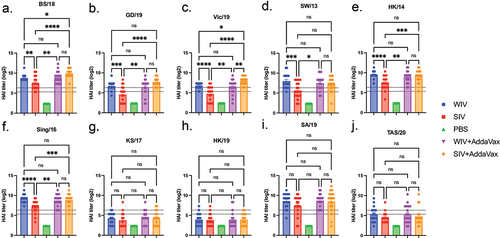
AddaVax enhances acute and long-term protective antibodies elicited by COBRA-IIV vaccines
To test the immediate and long-term protection elicited by the COBRA-IIV vaccines, mice were challenged with BS/18 (H1N1) or SW/13 (H3N2) at week 8 or 24, which is 4 or 20 weeks following the booster vaccination (). The mice were monitored for viral replication and body weight loss after the challenges. The statistical analysis of the body weight loss is listed in Suppl Table S1. Mock or adjuvant only vaccinated mice that were challenged 4 weeks post-boost rapidly lost weight following infection with BS/18 (). By 6DPI, all mice had lost >25% of their original body weight and succumbed to infection. Mock vaccinated mice immediately challenged with SW/13 lost ~15% of their original weight by 6DPI and then slowly regained their weight (). In contrast, mock vaccinated mice that were vaccinated challenge with these same viruses 20 weeks post-boost had severe weight loss and clinical signs of influenza infection. Once again, mock or adjuvant only vaccinated mice challenged with BS/18 lost ~25% body weight by 6DPI and subsequently died from infection (). Meanwhile, mock vaccinated mice challenged with SW/13 lost >20% of their original body weight by 10DPI and did not significantly recover for the 14 days of observation (). All vaccinated mice survived challenges with significantly less body weight loss than the mock vaccinated mice. Among all tested vaccines, mice vaccinated with the COBRA-SIV without AddaVax lost the most body weight over the 14-day period of observation (). In general, mice administered vaccine plus AddaVax adjuvant lost less body weight than mice administered vaccine without AddaVax, except in mice that were challenged with BS/18 at 20 weeks post-boost. Mice vaccinated with the COBRA-WIV plus AddaVax lost more weight than mice vaccinated with COBRA-WIV without AddaVax but recovered rapidly within 6 days ().
Figure 4. AddaVax can improve the acute and long-term protection elicited by COBRA-IIV in naïve mice.
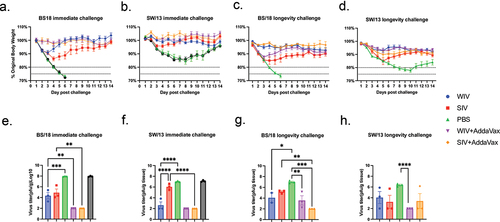
Mock vaccinated mice had high viral lung titers at 3DPI (~10^8 pfu/g) when challenged 4 weeks post-boost (), with similar, but ~1 log less, in mice challenged 20 weeks post-boost (). Mice vaccinated with the vaccines without adjuvant had some mice with detectable lung viral titers, whereas mice vaccinated with COBRA-WIV or COBRA-SIV plus AddaVax adjuvant had mostly no detectable viral lung titers, regardless if they were challenged at 4 or 18 weeks post-boost ().
To better understand why mice were less protected against viral infection at 20 weeks compared to those challenged at 4 weeks post-boost, antibody titers were examined in more details. Mice still maintained at high HAI titers at week 20 post-boost (Suppl Figure S3), but the anti-HA IgG titers decreased compared to the level compared to the titers assessed at week 2 post-boost (Suppl Figure S4). Overall, at 2 weeks post-boost, mice vaccinated with the SIV formulated with AddaVax had higher anti-HA IgG than mice vaccinated with any of the other three vaccines (Suppl Figure S4a,b). These mice had a predominate IgG1 isotype profile with little IgG2a or IgG2b. This pattern was similar in mice vaccinated with WIV plus AddaVax, as well as unadjuvanted SIV. However, mice vaccinated with unadjuvanted WIV, there was a slight bias towards IgG2a anti-HA antibodies, albeit a lower level. The similar patterns was observed in sera collected at 20 weeks post-boost, even though the overall titers were lower (Suppl Figure S4c,d).
COBRA-IIVs can elicit broad and long-lasting antibodies in pre-immune mice
Based upon vaccine performance in immunologically naïve mice, the next set of studies only used adjuvanted COBRA-IIV vaccines. Herein, these adjuvanted vaccines were tested in mice with preexisting anti-influenza immune responses (). Mice were infected intranasally with historical H1N1 (Sing/86) and H3N2 (Pan/99) strains and allowed to rest 28 days prior to vaccination. Sing/86-specific and Pan/99-specific antibodies were detected in the sera collected 14 days after the pre-infection. Following vaccination, mice had sera with HAI activity (>1:40) 2 weeks post-boost against all the H1N1 and 5 of the 7 H3N2 strains tested (). Mice vaccinated with COBRA-SIV plus AddaVax tended to have higher HAI titers against the panel of influenza viruses than mice vaccinated with COBRA-WIV plus AddaVax. Similar results were observed at 20 weeks post-boost with these HAI titers maintained over the 20-week period (Suppl ). In addition, splenocytes collected from vaccinated mice at 7 days post-boost had similar numbers of anti-HA secreting antibody cells, regardless of the vaccine administered (). Following only one vaccination, the adjuvanted COBRA-IIV vaccine recalled IgG secreting memory B cells; however, they were not Y2 HA specific memory B cells (). Interestingly, mice vaccinated with the COBRA-WIV plus AddaVax had more IgG2a and IgG2b, rather than IgG1 secreting B cells (). While in contrast, mice vaccinated with the COBRA-SIV plus AddaVax had more IgG1 secreting B cells (). This evidence indicates that the COBRA-WIV stimulates more Th1 pathway, while COBRA-SIV stimulates more Th2 pathway.Citation29
Figure 5. COBRA-IIVs plus AddaVax elicited long-lasting broadly reactive antibodies and recalled memory B cells in pre-immune mice.
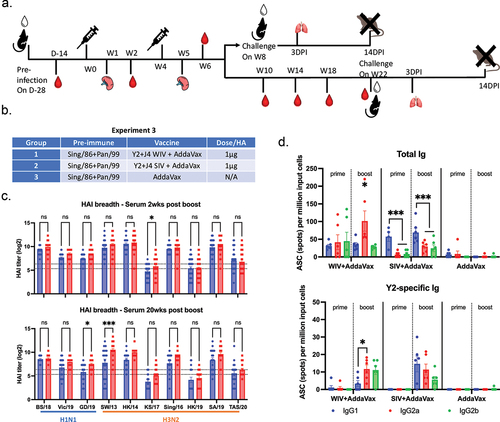
Compared to the immunologically naïve vaccinated mice, mice with preexisting immune responses to historical influenza viruses that received the same vaccines had sera with HAI activity that recognized a broader number of influenza virus strains (). Interestingly, there were anti-HA stem-binding antibodies detected in both pre-immune and naïve mice. Following two vaccinations with either COBRA-WIV or COBRA-SIV vaccines, there were higher HA stem-binding antibody titers in the pre-immune mice compared to the naïve mice (Suppl Figure S6).
COBRA-IIV plus AddaVax elicited acute and long-term protection in pre-immune mice
To test the acute and long-term protection elicited by the COBRA-IIV vaccines, mice were challenged with H1N1 and H3N2 influenza viruses at 4 or 20 weeks after the boost vaccination (). The mice were monitored for viral replication and body weight loss after the challenges. The statistical analysis of the body weight loss is listed in Suppl Table S2. Adjuvant only vaccinated mice that were challenged 4 weeks post-boost lost ~15% of their original body weight by 3-4DPI and then slowly regained their weight, regardless if they were challenged with BS/18 or SW/13 influenza viruses (). All vaccinated mice had little weight loss following immediate BS/18 or SW/13 viral challenge (). All pre-immune mice survived the immediate challenges.
Figure 6. COBRA-IIV with AddaVax provided long-term protection in pre-immune mice.
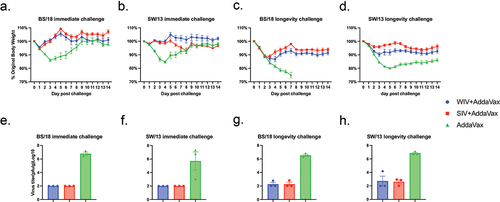
In contrast, mock vaccinated mice that were challenged with these same viruses 20 weeks post-boost had severe weight loss and clinical signs of influenza infection. These mice challenged with BS/18 lost ~25% body weight by 7DPI and subsequently died from infection (). Mock vaccinated mice challenged with SW/13 had similar severe disease losing 20% of their original body weight by 5DPI and did not significantly recover for the 14 days of observation (). Mice vaccinated with either COBRA-WIV or COBRA-SIV lost ~5–10% of their original body weight and had fewer clinical symptoms of disease (). While there was no difference in the survival rate of naïve and pre-immune mice following SW/13 challenge, BS/18 was lethal in naïve mice, but not in pre-immune mice when challenged 3 months after the pre-infection (Suppl Figure S7a). Pre-immune mice had lower viral lung titers at 3DPI compared to naïve mice (Suppl Figure S7b). However, at 7 months after the pre-infection, there was no difference in the survival rate or virus lung titers between mock vaccinated pre-immune mice and naïve mice (Suppl Figure S7).
Mock vaccinated mice had high viral lung titers at 3DPI (~10^8 pfu/g) when challenged at 4 weeks post-boost (), with similar viral titers in mice challenged at 20 weeks post-boost (). Mice vaccinated with COBRA-WIV or COBRA-SIV plus AddaVax adjuvant had no detectable viral lung titers 3 DPI following challenge 4 weeks post-boost with either BS/18 or SW/13 (). However, when mice vaccinated with the same vaccines were challenged at 24 weeks post boost, there were detectable viral lung titers collected at 3DPI, albeit the overall viral titers were significantly lower than the mock vaccinated mice ().
Discussion
To overcome the hypervariation of influenza viruses, improved vaccine antigens are necessary to elicit broadly reactive, long-lasting immune responses. To address the development of next-generation influenza vaccines, the COBRA methodology was developed and used to test HA or NA vaccine antigens in a variety of formats.Citation11,Citation14,Citation15 In this study, WIV and SIV platforms expressing the COBRA HA proteins were tested for effectiveness in naïve and pre-immune mouse models.
Compared to WT-WIV, COBRA-WIV elicited enhanced broadly reactive antibodies against heterologous drifted strains compared to the WT-WIV (). More importantly, the reduced dose of COBRA-WIV still elicited seroprotective antibodies against multiple influenza strains () and protected naïve mice from lethal challenge following two vaccinations (Suppl ). This further confirmed the COBRA-WIV’s effectiveness and its potential for commercial use. However, with the wide HAI breadth, the COBRA-IIVs did not induce seroprotective antibodies with HAI activity against KS/17 in naïve mice. A similar result was reported in the previous research that tested the J4-based rHA vaccine.Citation12 This is likely due to the genetic distance between the HA of KS/17 and the J4 structures ( and Suppl Figure S8). KS/17 belongs to clade 3c3.a and the J4 is based on H3N2 strains circulating from 2013 to 2016, most of which belong to clade 3c2. It was reported that vaccination of the 3c3.a strain could only elicit very limited reactivities to the 3c2 strains.Citation30 Meanwhile, our FRA results showed the COBRA-WIV could elicit abundant KS/17-specific neutralizing antibodies (), which indicated that the neutralizing antibodies did not target KS/17 HA’s receptor-binding site but other sites, for example, the stem region. The stem-binding Abs have been reported to exhibit robust neutralization activities and, more importantly, a potent cross-reaction.Citation31
The influenza WIV was previously reported to have more adverse side effects,Citation5 however, the SIV is generally insufficient to stimulate protective immune responses by itself, especially in populations with weaker immunity. In this study, the WIV elicited higher HAI titers () and better immediate and long-term protection against the viral infection than the SIV (). Some commercial flu vaccines containing SIVs employ adjuvant to improve their VE, for example, the Fluad Quadrivalent approved in 2020 for people over 65 years old is adjuvanted with MF59, an oil-in-water emulsion adjuvant.Citation32 To improve the effectiveness of COBRA-IIVs, AddaVax, equivalent to MF59, was used in this study. The addition of AddaVax to COBRA-SIV improved the HAI titers against most strains () and prevented body weight loss and viral replication after exposure to challenge viruses (). Interestingly, the COBRA-WIV vaccine effectively elicited high titer, protective antibodies, with HAI activity following two vaccinations without the use of adjuvant. A possible explanation is that the COBRA-WIV vaccine may have self-adjuvanting effects elicited by internal viral proteins. For example, the viral RNA in the WIV can activate innate immunity by stimulating the Toll-like receptor 7 (TLR7).Citation33 Thereby, the addition of AddaVax adjuvant did not affect the effectiveness of COBRA-WIV on eliciting protective antibodies. However, the COBRA-WIV plus AddaVax provided better protection against the immediate viral infection than the COBRA-WIV by itself post-infection (), which indicates that the AddaVax also enhanced the VE of COVRA-WIV. Considering their similar BS/18-specific antibody levels before the challenge, we hypothesize that the AddaVax improved cellular immunity, eliminating the infectious viruses rapidly. Moreover, higher IgG, especially IgG1 level was detected in the COBRA-WIV plus AddaVax group than in the COBRA-WIV group (Suppl Figure S4a), indicating the elevated Th pathway.Citation29 In contrast, in the longevity study, the COBRA-WIV plus AddaVax group experienced more body weight loss than the COBRA-WIV group post-infection ( and Suppl Table S1). A possible explanation is that the adjuvanted COBRA-WIV recalled excessive immune responses that caused the loss of appetite.Citation34 They recovered to ~95% of the original body weight rapidly, indicating no tissue damages caused post-infection, and the significantly lower virus titer in the lungs () further confirmed the long-term effectiveness of COBRA-WIV plus AddaVax.
Pre-immune animal models have been broadly used in pre-clinical studies because of the original antigenic sin (OAS).Citation35 Natural infection can more strongly elicit antibodies with a broader spectrum than the vaccination,Citation36 therefore, immune memory is primarily driven by prior influenza virus infections compared to the vaccination.Citation36 In this study, COBRA-IIV vaccines elicited antibodies with HAI activity that recognized a broader number of H3N2 influenza viruses in mice pre-immune to historical influenza strains than immunologically naïve mice, which could result from increased H3 stem-binding antibody level (Suppl Figure S5b). The effect of preexisting antibodies did not appear to affect the breadth of HAI activity against H1N1 influenza viruses, and surprisingly, the H1 stem-binding antibody titers in pre-immune mice were lower than titers in naïve mice at 2 weeks post-boost (Suppl Figure S5a). This could be a result from the large genetic distance (80.21% identity) between the HA of Sing/86 and Y2 that is based on the circulating H1N1 strains from 2016 to 2018 and are all CA/09-like strains (). However, mice pre-immune to historical strains had higher titers of anti-H1 stem-binding antibodies than naïve mice 20 weeks post-boost. It could be because that in the naïve mice, the H1-stem binding antibodies mainly targeted the Y2 stem region but decayed faster than the H1-stem binding antibodies in the pre-immune mice that targeted the common epitopes shared by both Y2 and Pan/99 HA stems. It was reported that antibodies with a wider spectrum have a longer lifespan than highly specific ones.Citation37
To examine the longevity of the induced antibodies, mice were challenged at week 20 post-boost vaccination. One key to forming long-term protection is to stimulate the immune memory cells.Citation38 In this study, COBRA-IIV vaccines efficiently recalled memory B cells () that maintained seroprotective antibody titers for 20 weeks after the vaccination. The COBRA-WIV and COBRA-SIV showed different Th1 and Th2 pathway biases, respectively (), even though the AddaVax is proven to elicit a balanced Th1/2 response.Citation39 A similar result was reported previously that the WIV favored more of the Th1 response.Citation40 This evidence indicates that the COBRA-WIV is more efficient in stimulating the cytotoxic T lymphocytes (CTL) that eliminate intracellular pathogens, while the COBRA-SIV stimulates more humoral immunity that generates more antibodies.Citation40 Even though the challenge viruses broke through the adjuvanted COBRA-IIVs in the longevity study in both naïve and pre-immune models, the infected viruses were eliminated later probably via cellular immunity or antibody-dependent cellular cytotoxicity (ADCC).Citation41
Interestingly, the adjuvanted COBRA-IIV vaccines reduced viral replication in pre-immune mice than in naïve mice following the longevity challenge (), which appears to indicate that the pre-exposure history may enhance the long-term protective immunity elicited by these IIV vaccines. Moreover, mock vaccinated, pre-immune mice were protected against viral challenge 3 months after pre-infection but not 7 months after pre-infection, indicating that without subsequent vaccination, the immune responses induced by the pre-infection viruses were maintained for 3–7 months. However, the histological H1N1 and H3N2 pre-infected mice did not elicit antibodies with HAI activity against any currently circulating H1N1 or H3N2 influenza strains.Citation12,Citation27 Therefore, the protection is likely the result of other non-HAI mechanisms, such as cellular-induced immune responses or an anti-viral state activated by the viral infection, or by non-HAI antibody binding to the HA or other viral proteins.Citation42,Citation43
To establish the pre-exposure history, we utilized the historic H1N1 (Sing/86) and H3N2 (Pan/99) strains because of their wide prevalence in the past. However, with the rapid evolution, the seasonal H1N1 influenza viruses were circulating in the human population until 2009 when panH1N1 influenza viruses, such as CA/09, emerged and caused a pandemic.Citation44 Therefore, people born after 2009 may only have been exposed to panH1N1-like viruses, and in the future, the panH1N1-specific immunity will be dominant in these populations as adults and therefore, pre-immune models using panH1N1 strains should be developed and used for testing next-generation influenza vaccines.
In summary, the COBRA HA-based IIV vaccines elicited broadly reactive and durable protection against future drifted influenza A viruses in both immunologically naïve mice, as well as mice with preexisting immunity and subsequently vaccinated twice. Considering the well-regulated manufacturing process of IIV vaccines and the approval of adjuvanted SIV (Fluad Quadrivalent, Sanofi-Pasteur, Swiftwater, PA, USA) for vaccination in the elderly, the path forward to license adjuvanted COBRA-IIV vaccines may be straight forward and is a promising candidate for a next-generation influenza vaccine.
Supplemental Figure 1.tiff
Download TIFF Image (12.2 MB)Supplemental figure 2_.tif
Download TIFF Image (2.3 MB)Supplemental Figure 4_.tif
Download TIFF Image (4.1 MB)Supplemental Figure 8.tiff
Download TIFF Image (24.3 MB)Supplemental_table_1_.docx
Download MS Word (15.4 KB)Supplemental Figure 6_.tif
Download TIFF Image (2.6 MB)Supplemental Figure 7.tiff
Download TIFF Image (3 MB)Supplemental Figure 3.tiff
Download TIFF Image (5.6 MB)Supplemental_table_2_.docx
Download MS Word (14.9 KB)Supplemental figure 5.tiff
Download TIFF Image (4.8 MB)Acknowledgments
We would like to thank James Allen and Ivette Nuñez for designing COBRA HA vaccines and Spencer Pierce for purifying the HA antigens. We thank Naoko Uno technical assistance. We also thank the University of Georgia Animal Resource staff, technicians, and veterinarians for the excellent animal care. We also appreciate Benjamin Chadwick for proofreading and language editing for this paper. This project has been funded as part of the Collaborative Influenza Vaccine Innovations Centers (CIVICs) by the National Institute of Allergy and Infectious Diseases, a component of the NIH, Department of Health and Human Services, under contract 75N93019C00052. TMR is also supported in part as a Georgia Eminent Scholar by the Georgia Research Alliance, GRA-001.
Disclosure statement
No potential conflict of interest was reported by the author(s).
Data availability statement
The data that support the findings of this study are available from the corresponding author upon reasonable request.
Supplementary material
Supplemental data for this article can be accessed on the publisher’s website at https://doi.org/10.1080/21645515.2024.2356269
Additional information
Funding
Notes
[a] The multiple alignment is shown in Supplemental Figure S8.
References
- Iuliano AD, Roguski KM, Chang HH, Muscatello DJ, Palekar R, Tempia S, Cohen C, Gran JM, Schanzer D, Cowling BJ. Estimates of global seasonal influenza-associated respiratory mortality: a modelling study. Lancet. 2018;391(10127):1285–12. doi: 10.1016/S0140-6736(17)33293-2.
- Vemula SV, Zhao J, Liu J, Wang X, Biswas S, Hewlett I. Current approaches for diagnosis of influenza virus infections in humans. Viruses. 2016;8(4):96. doi: 10.3390/v8040096.
- Alymova IV, York IA, Air GM, Cipollo JF, Gulati S, Baranovich T, Kumar A, Zeng H, Gansebom S, McCullers JA. Glycosylation changes in the globular head of H3N2 influenza hemagglutinin modulate receptor binding without affecting virus virulence. Sci Rep. 2016;6(1):1–15. doi: 10.1038/srep36216.
- Stöhr K, Bucher D, Colgate T, Wood J, Le J, Manojkumar R, Pokorny BA, Silverman J, Bucher D, Wood JM. Influenza virus surveillance, vaccine strain selection, and manufacture. In: Kawaoka Y, Neumann G, editors. Influenza virus: methods and protocols. New York, NY, USA: Springer; 2012. p. 147–62.
- Barberis I, Myles P, Ault S, Bragazzi N, Martini M. History and evolution of influenza control through vaccination: from the first monovalent vaccine to universal vaccines. J Prev Med Hyg. 2016;57(3):E115.
- Huang X, Fan T, Li L, Nian X, Zhang J, Gao X, Zhao W, Chen W, Zhang Z, Yao Z. Safety and immunogenicity of a quadrivalent, inactivated, split-virion influenza vaccine (IIV4-W) in healthy people aged 3–60 years: a phase III randomized clinical noninferiority trial. Hum Vaccines Immunother. 2022;18(5):2079924. doi: 10.1080/21645515.2022.2079924.
- Lewnard JA, Cobey S. Immune history and influenza vaccine effectiveness. NATO Adv Sci Inst Se. 2018;6(2):28. doi: 10.3390/vaccines6020028.
- Weir JP, Gruber MF. An overview of the regulation of influenza vaccines in the United States. Influenza Other Respi Viruses. 2016;10(5):354–60. doi: 10.1111/irv.12383.
- Erbelding EJ, Post DJ, Stemmy EJ, Roberts PC, Augustine AD, Ferguson S, Paules CI, Graham BS, Fauci AS. A universal influenza vaccine: the strategic plan for the national institute of allergy and infectious diseases. J Infect Dis. 2018;218(3):347–54. doi: 10.1093/infdis/jiy103.
- Skehel JJ, Wiley DC. Receptor binding and membrane fusion in virus entry: the influenza hemagglutinin. Annu Rev Biochem. 2000;69(1):531–69. doi: 10.1146/annurev.biochem.69.1.531.
- Giles BM, Ross TM. A computationally optimized broadly reactive antigen (COBRA) based H5N1 VLP vaccine elicits broadly reactive antibodies in mice and ferrets. Vaccine. 2011;29(16):3043–54. doi: 10.1016/j.vaccine.2011.01.100.
- Allen JD, Ross TM, Subbarao K. Bivalent H1 and H3 COBRA recombinant hemagglutinin vaccines elicit seroprotective antibodies against H1N1 and H3N2 influenza viruses from 2009 to 2019. J Virol. 2022;96(7):e01652–01621. doi: 10.1128/jvi.01652-21.
- Carter DM, Darby CA, Lefoley BC, Crevar CJ, Alefantis T, Oomen R, Anderson SF, Strugnell T, Cortés-Garcia G, Vogel TU, et al. Design and characterization of a computationally optimized broadly reactive hemagglutinin vaccine for H1N1 influenza viruses. J Virol. 2016;90(9):4720–34. doi: 10.1128/JVI.03152-15.
- Giles BM, Crevar CJ, Carter DM, Bissel SJ, Schultz-Cherry S, Wiley CA, Ross TM. A computationally optimized hemagglutinin virus-like particle vaccine elicits broadly reactive antibodies that protect nonhuman primates from H5N1 infection. J Infect Dis. 2012;205(10):1562–70. doi: 10.1093/infdis/jis232.
- Huang Y, França MS, Allen JD, Shi H, Ross TM. Next generation of computationally optimized broadly reactive HA vaccines elicited cross-reactive immune responses and provided protection against H1N1 virus infection. NATO Adv Sci Inst Se. 2021;9(7):793. doi: 10.3390/vaccines9070793.
- Wong TM, Allen JD, Bebin-Blackwell A-G, Carter DM, Alefantis T, DiNapoli J, Kleanthous H, Ross TM, Schultz-Cherry S. Computationally optimized broadly reactive hemagglutinin elicits hemagglutination inhibition antibodies against a panel of H3N2 influenza virus cocirculating variants. J Virol. 2017;91(24):e01581–01517. doi: 10.1128/JVI.01581-17.
- Nachbagauer R, Feser J, Naficy A, Bernstein DI, Guptill J, Walter EB, Berlanda-Scorza F, Stadlbauer D, Wilson PC, Aydillo T, et al. A chimeric hemagglutinin-based universal influenza virus vaccine approach induces broad and long-lasting immunity in a randomized, placebo-controlled phase I trial. Nat Med. 2021;27(1):106–14. doi: 10.1038/s41591-020-1118-7.
- Skarlupka AL, Ross TM. Immune imprinting in the influenza ferret model. NATO Adv Sci Inst Se. 2020;8(2):173. doi: 10.3390/vaccines8020173.
- Vatti A, Monsalve DM, Pacheco Y, Chang C, Anaya J-M, Gershwin ME. Original antigenic sin: a comprehensive review. J Autoimmun. 2017;83:12–21. doi: 10.1016/j.jaut.2017.04.008.
- Zhou B, Meliopoulos VA, Wang W, Lin X, Stucker KM, Halpin RA, Stockwell TB, Schultz-Cherry S, Wentworth DE, Dermody TS. Reversion of cold-adapted live attenuated influenza vaccine into a pathogenic virus. J Virol. 2016;90(19):8454–63. doi: 10.1128/JVI.00163-16.
- Eisfeld AJ, Neumann G, Kawaoka Y. Influenza a virus isolation, culture and identification. Nat Protoc. 2014;9(11):2663–81. doi: 10.1038/nprot.2014.180.
- Pica N, Iyer A, Ramos I, Bouvier NM, Fernandez-Sesma A, García-Sastre A, Lowen AC, Palese P, Steel J. The DBA.2 mouse is susceptible to disease following infection with a broad, but limited, range of influenza a and B viruses. J Virol. 2011;85(23):12825–9. doi: 10.1128/jvi.05930-11.
- Use CFMPFH. Guideline on influenza vaccines—non-clinical and clinical module. Eur Med Agency EMA/CHMP/VWP/457259/2014. 2016;44:1–31.
- Allen JD, Ross TM. Next generation methodology for updating HA vaccines against emerging human seasonal influenza a (H3N2) viruses. Sci Rep. 2021;11(1):4554. doi: 10.1038/s41598-020-79590-7.
- Allen JD, Ray S, Ross TM, Kang S-M. Split inactivated COBRA vaccine elicits protective antibodies against H1N1 and H3N2 influenza viruses. PLOS ONE. 2018;13(9):e0204284. doi: 10.1371/journal.pone.0204284.
- Allen JD, Ross TM. H3N2 influenza viruses in humans: Viral mechanisms, evolution, and evaluation. Hum Vaccines Immunother. 2018;14(8):1840–7. doi: 10.1080/21645515.2018.1462639.
- Carter DM, Darby CA, Johnson SK, Carlock MA, Kirchenbaum GA, Allen JD, Vogel TU, Delagrave S, DiNapoli J, Kleanthous H, et al. Elicitation of protective antibodies against a broad panel of H1N1 viruses in ferrets preimmune to historical H1N1 influenza viruses. J Virol. 2017;91(24):e01283–01217. doi: 10.1128/JVI.01283-17.
- Ross TM, Gokanapudi N, Ge P, Shi H, Richardson RA, Pierce SR, Sanchez P, Ullah S, De Luca E, Sautto GA, et al. Kinetic of the antibody response following AddaVax-adjuvanted immunization with recombinant influenza antigens. Vaccines. 2022;10:1315.
- Firacative C, Gressler AE, Schubert K, Schulze B, Müller U, Brombacher F, von Bergen M, Alber G. Identification of T helper (Th)1- and Th2-associated antigens of Cryptococcus neoformans in a murine model of pulmonary infection. Sci Rep. 2018;8(1):2681. doi: 10.1038/s41598-018-21039-z.
- Gouma S, Weirick M, Hensley SE. Antigenic assessment of the H3N2 component of the 2019–2020 Northern Hemisphere influenza vaccine. Nat Commun. 2020;11(1):2445. doi: 10.1038/s41467-020-16183-y.
- Bangaru S, Zhang H, Gilchuk IM, Voss TG, Irving RP, Gilchuk P, Matta P, Zhu X, Lang S, Nieusma T. A multifunctional human monoclonal neutralizing antibody that targets a unique conserved epitope on influenza HA. Nat Commun. 2018;9(1):2669. doi: 10.1038/s41467-018-04704-9.
- Pulendran B, Arunachalam S, P. & O’Hagan DT. Emerging concepts in the science of vaccine adjuvants. Nat Rev Drug Discov. 2021;20(6):454–75. doi: 10.1038/s41573-021-00163-y.
- Akira S, Uematsu S, Takeuchi O. Pathogen recognition and innate immunity. Cell. 2006;124(4):783–801. doi: 10.1016/j.cell.2006.02.015.
- Gardner EM, Beli E, Clinthorne JF, Duriancik DM. Energy intake and response to infection with influenza. Annu Rev Nutr. 2011;31(1):353–67. doi: 10.1146/annurev-nutr-081810-160812.
- Kim JH, Skountzou I, Compans R, Jacob J. Original antigenic sin responses to influenza viruses. J Immunol. 2009;183(5):3294–301. doi: 10.4049/jimmunol.0900398.
- Krammer F. The human antibody response to influenza a virus infection and vaccination. Nat Rev Immunol. 2019;19(6):383–97. doi: 10.1038/s41577-019-0143-6.
- Nachbagauer R, Choi A, Hirsh A, Margine I, Iida S, Barrera A, Ferres M, Albrecht RA, García-Sastre A, Bouvier NM. Defining the antibody cross-reactome directed against the influenza virus surface glycoproteins. Nat Immunol. 2017;18(4):464–73. doi: 10.1038/ni.3684.
- Kurosaki T, Kometani K, Ise W. Memory B cells. Nat Rev Immunol. 2015;15(3):149–59. doi: 10.1038/nri3802.
- Nian X, Zhang J, Deng T, Liu J, Gong Z, Lv C, Yao L, Li J, Huang S, Yang X. Addavax formulated with PolyI: C as a potential adjuvant of MDCK-based influenza vaccine enhances local, cellular, and antibody protective immune response in mice. Aaps Pharm Sci Tech. 2021;22(8):270. doi: 10.1208/s12249-021-02145-0.
- Hovden AO, Cox R, Haaheim L. Whole influenza virus vaccine is more immunogenic than split influenza virus vaccine and induces primarily an IgG2a response in BALB/c mice. Scand J Immunol. 2005;62(1):36–44. doi: 10.1111/j.1365-3083.2005.01633.x.
- Jegaskanda S, Reading PC, Kent SJ. Influenza-specific antibody-dependent cellular cytotoxicity: toward a universal influenza vaccine. J Immunol. 2014;193(2):469–75. doi: 10.4049/jimmunol.1400432.
- Heui Seo S, Hoffmann E, Webster RG. Lethal H5N1 influenza viruses escape host anti-viral cytokine responses. Nat Med. 2002;8(9):950–4. doi: 10.1038/nm757.
- Soghoian DZ, Streeck H. Cytolytic CD4+ T cells in viral immunity. Expert Rev Vaccines. 2010;9(12):1453–63. doi: 10.1586/erv.10.132.
- Hay AJ, Gregory V, Douglas AR, Lin YP. The evolution of human influenza viruses. Phil Trans R Soc Lond B. 2001;356(1416):1861. doi: 10.1098/rstb.2001.0999.