Abstract
We previously presented the cloning, heterologous expression, and characterization of a novel multidomain endoxylanase from Arthrobacter sp. GN16 isolated from the feces of Grus nigricollis. Molecular and biochemical characterization studies indicate that the glycoside hydrolase (GH) family 10 domain at the N-terminus of the multidomain xylanase (rXynAGN16L) is a low-temperature-active endoxylanase. Many low-temperature-active enzymes contain regions of high local flexibility related to their kinetic and thermodynamic properties compared with mesophilic and thermophilic enzymes. However, the thermodynamic property of low-temperature-active xylanases, including rXynAGN16L, has rarely been reported. In this study, the kinetic and thermodynamic properties of rXynAGN16L were determined using different substrates and temperature conditions to completely characterize its activity properties. The kinetic property of rXynAGN16L is similar to some low-temperature-active GH 10 endoxylanases. Moreover, the thermodynamic property indicates that rXynAGN16L is typically characterized as a low-temperature-active enzyme.
Introduction
Xylan is the most common hemicellulosic polysaccharide.Citation1 The structure of xylan contains substitute groups of acetyl, 4-O-methyl-d-glucuronosyl, and α-arabinofuranosyl residues that are linked to the backbone of β-1,4-linked xylopyranose units.Citation1 Endoxylanases (endo-β-1,4-xylanases; EC 3.2.1.8) are glycosidases that can catalyze the endohydrolysis of the xylan backbone; therefore, these enzymes have been extensively applied in many industries, including food, feed, energy, textile, paper, and pulp.Citation1
More than 80% of the Earth's biosphere, including oceans, alpine, polar regions, and caves, is permanently cold, and the remaining biosphere comprises seasonally cold environments.Citation2,3 The highest global proportion of biomass is generated at low temperatures.Citation2,3 Low-temperature-active enzymes exhibit high catalytic activity at low temperatures and can be potentially used in the cleaning, food, and feed industries; moreover, biotechnological processes are performed at cold temperatures to decrease economical cost and/or prevent product denaturation.Citation3
In recent years, low-temperature-active xylanases have elicited much attention.Citation4-13 The use of low-temperature-active xylanases can effectively improve dough properties and final bread volume (up to 28%).Citation14 Some studies have investigated the catalytic adaptation to low temperatures of xylanases.Citation4-7,13 The majority of low-temperature-active xylanases belong to the glycoside hydrolase (GH) family 10.Citation6
We previously presented the molecular and biochemical characterization of a novel multidomain endoxylanase from Arthrobacter sp GN16 isolated from the feces of Grus nigricollis.Citation13 In the previous study, the GH 10 catalytic domain at the N-terminus of the multidomain xylanase (rXynAGN16L) was expressed in Escherichia coli and the purified recombinant enzyme was characterized.Citation13 Biochemical characterization indicates that rXynAGN16L plays a key role in catalysis of xylans and is active at low temperatures.Citation13 Molecular characterization reveals that the structural adaptation of rXynAGN16L to low temperatures may be ascribed to the surface loop from A57 to Y77 and the decreased salt bridges.Citation13 Many low-temperature-active enzymes contain regions of high local flexibility related to their kinetic and thermodynamic properties.Citation3 However, the thermodynamic property of low-temperature-active xylanases, including rXynAGN16L, has rarely been reported.Citation4 In the present study, the kinetic and thermodynamic properties of rXynAGN16L were determined using different substrates and temperature conditions to completely characterize its activity properties.
Kinetic characterization
Km, Vmax, and kcat values of the purified rXynAGN16L toward xylans were determined using our previously described method.Citation13 The kinetic values of rXynAGN16L were calculated according to the double-reciprocal plots (), and the results are summarized in . Km, Vmax, kcat, and kcat/Km values of the purified rXynAGN16L toward beechwood xylan increased from 1.42 mg/mL to 1.81 mg/mL, 4.00 μmol/min/mg to 62.89 μmol/min/mg, 3.13/s to 49.17/s, and 2.20 mL/mg/s to 27.17 mL/mg/s, respectively, when the temperature increased from 0°C to 45°C. In addition, the Km value determined at 30°C was 2.21 mg/mL. Km, Vmax, kcat, and kcat/Km values of the purified rXynAGN16L toward birchwood xylan increased from 1.62 mg/mL to 2.61 mg/mL, 5.31 μmol/min/mg to 65.36 μmol/min/mg, 4.15/s to 51.10/s, and 2.56 mL/mg/s to 19.58 mL/mg/s, respectively, when the temperature increased from 0°C to 45°C. These results implied that rXynAGN16L exhibits higher affinity and catalytic efficiency toward beechwood xylan than toward birchwood xylan. The low-temperature-active GH 10 endoxylanases from Glaciecola mesophila KMM 241, Flavobacterium johsoniae, and goat rumen contents also exhibit similar phenomenon.Citation5,11,12
Table 1. Kinetic characterization of rXynAGN16L.
Figure 1. Lineweaver–Burk plots of the purified rXynAGN16L determined using 0.5–10.0 mg/mL xylans as substrates in McIlvaine buffer (pH 5.5).
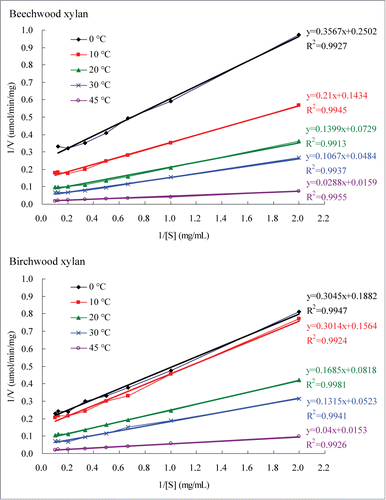
Many low-temperature-active enzymes present higher Km values than their more thermostable homologs.Citation3 However, the Km values of the low-temperature-active endoxylanases isolated from G. mesophila KMM 241, Flavobacterium sp. MSY2, and goat rumen contents toward beechwood xylan at 30°C are 1.22, 1.8, and 1.8 mg/mL, respectively;Citation8,11,12 these results are similar to the values of rXynAGN16L but lower than the values of many thermostable xylanases reviewed in a previous study.Citation15 In addition, the Km values of rXynAGN16L determined at low temperatures were higher than those determined at intermediate temperatures; this finding indicated that rXynAGN16L exhibits a high affinity toward xylan at low temperatures. The low-temperature-active endoxylanase from G. KMM 241 also exhibits similar phenomenon.Citation12 However, the Km values determined at 4°C of low-temperature-active endoxylanases from F. johsoniae and goat rumen contents toward xylans are higher than those determined at 30°C.Citation5,11
Many low-temperature-active enzymes have higher kcat values than their more thermostable homologs.Citation3 However, kcat values of the low-temperature-active endoxylanases isolated from G. mesophila KMM 241 and F. johsoniae toward beechwood xylan at 30°C are 69 and 10.70/s, respectively;Citation5,12 in addition, the value of rXynAGN16L is 16.15/s. The kcat values of the 3 low-temperature-active endoxylanases are lower than the values of many thermostable xylanases reviewed in a previous study.Citation15
Thermodynamic characterization
Activation energy (Ea), free energy of activation (ΔG*), enthalpy of activation (ΔH*), entropy of activation (ΔS*), and temperature coefficient (Q10) of rXynAGN16L were calculated using the equations described in a previous study.Citation16 The Arrhenius plots () show that the Ea values for the hydrolysis of beechwood and birchwood xylans by rXynAGN16L were 27.08 and 29.74 kJ/mol, respectively. Q10 values (35°C and 45°C) for the hydrolysis of beechwood and birchwood xylans in McIlvaine buffer (pH 5.5) by rXynAGN16L were 1.39 and 1.44, respectively. Other thermodynamic parameters, including ΔG*, ΔH*, and ΔS* at 0°C to 45°C in McIlvaine buffer (pH 5.5) for rXynAGN16L are summarized in . The ΔG* and ΔS* values of the purified rXynAGN16L toward beechwood xylan increased from 64.07 kJ/mol to 67.75 kJ/mol and from −143.80 J/mol/K to −136.21 J/mol/K, respectively, when the temperature increased from 0°C to 45°C; however, the ΔH* values decreased from 24.81 kJ/mol to 24.44 kJ/mol. The ΔG* and ΔS* values of the purified rXynAGN16L toward birchwood xylan increased from 63.43 kJ/mol to 67.65 kJ/mol and −134.32 J/mol/K to −127.53 J/mol/K, respectively, when the temperature increased from 0°C to 45°C; conversely, the ΔH* values decreased from 27.47 kJ/mol to 27.09 kJ/mol. In addition, the ΔS* value for rXynAGN16L toward birchwood xylan was −131.72 at 0°C.
Table 2. Thermodynamic characterization of rXynAGN16L
Many low-temperature-active enzymes present lower Ea values than thermostable homologs to allow easier conformational changes during catalysis at low temperatures.Citation3 The Ea of the thermophilic GH 10 endoxylanase from Bacillus halodurans TSEV1 for birchwood xylan hydrolysis is 30.51 kJ/mol,Citation17 which is higher than that required for rXynAGN16L. Furthermore, transition-state theory indicates that an equilibrium exists between the ground-state and transition- or activated-state reactants affected by the magnitude of the ΔG* barrier between them.Citation3 The ΔG* of the thermophilic GH 10 endoxylanase from B. halodurans TSEV1 for birchwood xylan hydrolysis is 197.65 kJ/mol;17 the result indicates that more energy is needed for the thermophilic endoxylanase to form the activated complex compared with rXynAGN16L.
Conclusion
This study presented the kinetic and thermodynamic properties of the GH 10 endoxylanase rXynAGN16L from Arthrobacter sp GN16 isolated from the feces of G. nigricollis. The kinetic property of rXynAGN16L is similar to that of some low-temperature-active GH 10 endoxylanases. Moreover, the thermodynamic property indicates that rXynAGN16L is typically characterized as a low-temperature-active enzyme.
Disclosure of potential conflicts of interest
No potential conflicts of interest were disclosed.
Funding
This work was supported by the Key Technologies Research and Development Program of China (2013BAD10B01), the National Natural Science Foundation of China (31260215), and the Applied and Basic Research Foundation of Yunnan Province (2011FB048).
References
- Collins T, Gerday C, Feller G. Xylanases, xylanase families and extremophilic xylanases. FEMS Microbiol Rev 2005; 29:3-23; PMID:15652973; http://dx.doi.org/10.1016/j.femsre.2004.06.005
- Rodrigues DF, Tiedje JM. Coping with our cold planet. Appl Environ Microb 2008; 74:1677-86; PMID:18203855; http://dx.doi.org/10.1128/aem.02000-07
- Siddiqui KS, Cavicchioli R. Cold-adapted enzymes. Annu Rev Biochem 2006; 75:403-33; PMID:16756497; http://dx.doi.org/10.1146/annurev.biochem.75.103004.142723
- Petrescu I, Lamotte-Brasseur J, Chessa JP, Ntarima P, Claeyssens M, Devreese B, Marino G, Gerday C. Xylanase from the psychrophilic yeast Cryptococcus adeliae. Extremophiles 2000; 4:137-44; PMID:10879558
- Chen S, Kaufman MG, Miazgowicz KL, Bagdasarian M, Walker ED. Molecular characterization of a cold-active recombinant xylanase from Flavobacterium johnsoniae and its applicability in xylan hydrolysis. Bioresource Technol 2013; 128:145-55; PMID:23196234; http://dx.doi.org/10.1016/j.biortech.2012.10.087
- Liu Q, Wang Y, Luo H, Wang L, Shi P, Huang H, Yang P, Yao B. Isolation of a novel cold-active family 11 xylanase from the filamentous fungus Bispora antennata and deletion of its N-terminal amino acids on thermostability. Appl Biochem Biotech 2015; 175:925-36; PMID:25351632; http://dx.doi.org/10.1007/s12010-014-1344-x
- Van Petegem F, Collins T, Meuwis MA, Gerday C, Feller G, Van Beeumen J. The structure of a cold-adapted family 8 xylanase at 1.3 angstrom resolution-structural adaptations to cold and investigation of the active site. J Biol Chem 2003; 278:7531-9; PMID:12475991; http://dx.doi.org/10.1074/jbc.M206862200
- Lee CC, Smith M, Kibblewhite-Accinelli R, Williams TG, Wagschal K, Robertson GH, Wong DWS. Isolation and characterization of a cold-active xylanase enzyme from Flavobacterium sp. Curr Microbiol 2006; 52:112-6; PMID:16450065; http://dx.doi.org/10.1007/s00284-005-4583-9
- Lee CC, Kibblewhite-Accinelli RE, Wagschal K, Robertson GH, Wong DWS. Cloning and characterization of a cold-active xylanase enzyme from an environmental DNA library. Extremophiles 2006; 10:295-300; PMID:16532363; http://dx.doi.org/10.1007/s00792-005-0499-3
- Zhou JP, Huang HQ, Meng K, Shi PJ, Wang YR, Luo HY, Yang PL, Bai YG, Zhou ZG, Yao B. Molecular and biochemical characterization of a novel xylanase from the symbiotic Sphingobacterium sp. TN19. Appl Microbiol Biot 2009; 85:323-33; PMID:19554324; http://dx.doi.org/10.1007/s00253-009-2081-x
- Wang G, Luo H, Wang Y, Huang H, Shi P, Yang P, Meng K, Bai Y, Yao B. A novel cold-active xylanase gene from the environmental DNA of goat rumen contents: direct cloning, expression and enzyme characterization. Bioresource Technol 2011; 102:3330-6; PMID:21106368; http://dx.doi.org/10.1016/j.biortech.2010.11.004
- Guo B, Chen XL, Sun CY, Zhou BC, Zhang YZ. Gene cloning, expression and characterization of a new cold-active and salt-tolerant endo-β-1,4-xylanase from marine Glaciecola mesophila KMM 241. Appl Microbiol Biot 2009; 84:1107-15; PMID:19506861; http://dx.doi.org/10.1007/s00253-009-2056-y
- Zhou JP, Shen JD, Zhang R, Tang XH, Li JJ, Xu B, Ding JM, Gao YJ, Xu DY, Huang ZX. Molecular and biochemical characterization of a novel multidomain xylanase from Arthrobacter sp. GN16 isolated from the feces of Grus nigricollis. Appl Biochem Biotech 2015; 175:573-88; PMID:25331377; http://dx.doi.org/10.1007/s12010-014-1295-2
- Dornez E, Verjans P, Arnaut F, Delcour JA, Courtin CM. Use of psychrophilic xylanases provides insight into the xylanase functionality in bread making. J Agr Food Chem 2011; 59:9553-62; PMID:21806059; http://dx.doi.org/10.1021/jf201752g
- Beg QK, Kapoor M, Mahajan L, Hoondal GS. Microbial xylanases and their industrial applications: a review. Appl Microbiol Biot 2001; 56:326-38; PMID:11548999; http://dx.doi.org/10.1007/s002530100704
- Siddiqui K, Cavicchioli R, Thomas T. Thermodynamic activation properties of elongation factor 2 (EF-2) proteins from psychrotolerant and thermophilic Archaea. Extremophiles 2002; 6:143-50; PMID:12013435; http://dx.doi.org/10.1007/s007920100237
- Kumar V, Satyanarayana T. Biochemical and thermodynamic characteristics of thermo-alkali-stable xylanase from a novel polyextremophilic Bacillus halodurans TSEV1. Extremophiles 2013; 17:797-808; PMID:23877303; http://dx.doi.org/10.1007/s00792-013-0565-1