Abstract
Poly(3-hydroxypropionate) (P3HP) is a biodegradable and biocompatible thermoplastic. Previous studies demonstrated that engineered Escherichia coli strains can produce P3HP with supplementation of expensive vitamin B12. The present study examined the production of P3HP from glycerol in the recombinant Klebsiella pneumoniae strain, which naturally synthesizes vitamin B12. The genes glycerol dehydratase and its reactivation factor (dhaB123, gdrA, and gdrB from K. pneumoniae), aldehyde dehydrogenase (aldH from E. coli) were cloned and expressed in K. pneumoniae to produce 3-hydroxypropionate (3HP), with 2 genes (dhaT and yqhD) for biosynthesis of 1,3-propanediol were deleted. To obtain P3HP production, propionyl-CoA synthetase (prpE from E. coli) and polyhydroxyalkanoate synthase (phaC from Ralstonia eutropha) were introduced. Under the appropriate aeration condition, the cell yield and P3HP content were 0.24 g/L and 12.7% (wt/wt [cell dry weight]) respectively along with 2.03 g/L 3HP after 48 h cultivation. Although the yield is relatively low, this study shows the feasibility of producing P3HP in K. pneumoniae from glycerol without vitamin B12 for the first time. The results also suggest that the aeration conditions should be optimized carefully for the efficient production of P3HP.
Introduction
As the main byproduct of biodiesel, about 10 tons of crude glycerol is produced with 100 tons of biodiesel produced via transesterification of vegetable oils or animal fats.Citation1 With the rapid development of the biodiesel industry, glycerol has become an inexpensive and abundant carbon source. Glycerol is both a cell metabolite and a carbon substrate for growth.Citation2 It can be converted into many valuable products, such as 1,3-propanediol,Citation3 lactate,Citation4 succinate,Citation5 3HP,Citation6 and polyhydroxyalkanoates (PHAs).Citation1
Poly(3-hydroxypropionate) (P3HP) is one kind of PHA exhibiting high rigidity, ductility, and exceptional tensile strength in drawn films, and was regarded as one of the alternatives to petrochemical-derived plastic.Citation1 Thus far, P3HP cannot be produced by any native bacterial strains. When some structurally-related carbon sources like 3HP, α,ω-alkanediols, acrylate, γ-butyrolactone or 1,3-propanediol were added, PHAs containing the 3HP monomers could be produced by some microbes like Alcaligenes eutrophus,Citation7 Alcaligenes latus,Citation8 Ralstonia eutropha,Citation9 Methylobacterium,Citation10 and engineered Escherichia coli.Citation11 However, the addition of these expensive precursors increased the production cost and restricted the further development.
The first attempt to produce PHAs containing the 3HP monomer from unrelated carbon sources was reported in 2009 by engineered Cupriavidus necator, and the product was P(3HP-co-3HB).Citation12 In recent years, P3HP was produced successfully from glycerol using engineered E. coli strains. However, E. coli requires the addition of vitamin B12 as the coenzyme of glycerol dehydratase employed in the pathway for conversion glycerol into 3-hydroxypropioaldehyde.Citation13 Vitamin B12 is expensive and economically not feasible.
Klebsiella pneumoniae is one kind of microorganism studied sufficiently which can produce vitamin B12 naturally.Citation14 It can grow well on glycerol as the sole carbon and energy source. In this study, recombinant strains of K. pneumoniae were developed and evaluated, which can produce P3HP from glycerol (). To improve the yield of 3HP, Q1546 was developed by disrupting 1,3-PDO oxidoreductases dhaT and yqhD, and overexpression of aldehyde dehydrogenase aldH from E. coli, glycerol dehydratase dhaB123 and glycerol dehydratase reactivation factor gdrAB from K. pneumoniae. In order to obtain P3HP, plasmid pBAD18-pp with propionyl-CoA synthetase prpE from E. coli and PHA synthase phaC from R. eutropha H16 was transformed into the above 3HP producing K. pneumoniae. The recombinant strain Q1643 was cultured in vitamin B12 free medium under a range of aeration conditions. The effects of aeration on 3HP and P3HP production were compared. This study shows the potential and limitation of K. pneumoniae in the production P3HP from glycerol in vitamin B12 free medium.
Figure 1. Metabolic pathway and genetic modification strategy for P3HP production by recombinant K. pneumoniae strain from glycerol. DhaB: glycerol dehydratase; AldH: aldehyde dehydrogenase; PrpE: propionyl-CoA synthetase; PhaC: polyhydroxyalkanoate synthetase; DhaT and YqhD: 1,3-propanediol oxidoreductases.
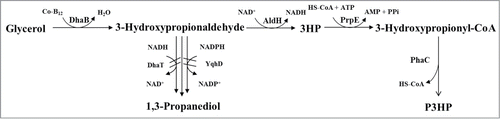
Results and discussion
Confirmation of target proteins in Klebsiella pneumoniae
Glycerol can be converted into 3-hydroxypropionaldehyde (3-HPA) through catalysis by glycerol dehydratase in K. pneumoniae. And then 3-HPA is reduced to 1,3-PDO by 1,3-PDO oxidoreductases or oxidized to 3HP by aldehyde dehydrogenase. The wild type K. pneumoniae did not produce 3HP at a significant level during glycerol fermentation and 1,3-PDO accumulated simultaneously.Citation15 To improve 3HP production, 1,3-PDO synthesis was disrupted by deleting dhaT and yqhD, and genes related to 3HP synthesis were cloned and overexpressed in K. pneumoniae ATCC25955.
The expression of cloned genes in Q1546 and Q1643 after growing in LB medium was analyzed. With L-arabinose induced, all the targeted proteins were observed as a prominent band with the expected molecular on SDS-PAGE (). The samples showed the presence of the target protein bands at 62 kDa (DhaB1 and GdrA), 55 kDa (AldH), 21 kDa (DhaB2), and 16 kDa (DhaB3 and GdrB), whereas the Q1643 cultures also showed a prominent band at 70 kDa (PrpE), and 40 kDa (PhaC). The results demonstrated that these proteins were properly expressed in recombinant strains.
3HP production by different recombinant strains
The production of 3HP in different recombinant strains were compared (). In this study, only 0.63 ± 0.07 g/L 3HP was produced by the control strain Q1188. To improve the 3HP biosynthesis, genes related to 1,3-PDO synthesis, dhaT and yqhD, were knocked out using suicide vector pRE112. After 48 h cultivation, the dhaT mutant strain Q1189 produced 1.51 ± 0.19 g/L 3HP and dhaT yqhD double mutant strain Q1153 accumulated 2.07 ± 0.11 g/L 3HP. Deleting of dhaT and yqhD enhanced the glycerol carbon flux toward to 3HP. The results were consistent with the previous report.Citation14
Figure 3. Production of 3HP by different recombinant strains. The strains were grown in minimal medium under shake flask condition in triplicate, and standard deviation was shown.
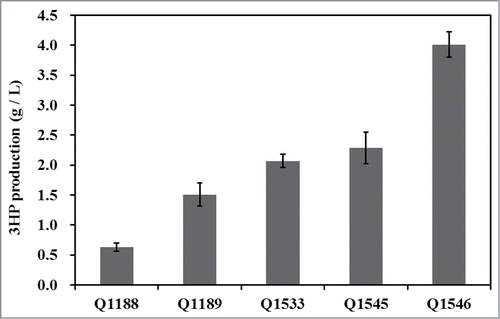
When target proteins were overexpressed, 3HP production was dramatically improved, Q1545 produced 2.29 ± 0.26 g/L 3HP and Q1546 produced 4.01 ± 0.21 g/L 3HP which were improved to 3.64 and 6.37 times from the production observed using the control strain Q1188 respectively. This shows that overexpression of the genes related to the synthesis of 3HP can play an important role in improving the production. As Q1546 exhibited the highest production, it was used as a host for producing P3HP in further study.
P3HP accumulation and analysis
For P3HP production, plasmid pBAD18-pp harboring propionyl-CoA synthetase (prpE from E. coli, which can convert 3HP to 3-hydroxypropionyl CoA), and polyhydroxyalkanoate synthase (phaC from R. eutropha, which can polymerize 3-hydroxypropionyl CoA into P3HP) was transferred into Q1546 and Q1643 was obtained. Q1643 was cultivated with glycerol as the sole carbon source. After 48 h cultivation, the cells were harvested and PHA was extracted with hot chloroform from lyophilized cells. The extracted PHA was dissolved in deuterated chloroform (50 mg/ml) to confirm the chemical structure by NMR analysis at room temperature with tetramethylisilane (TMS) as internal chemical shift standard. The characteristic 1H and 13C-NMR spectrum of the sample is presented in . The integrated peak areas of 1H-NMR spectrum were found to be 1, 1 respectively for the CH2(2), CH2(3). A similar identification has been already performed for poly(3-hydroxypropionate).Citation13 shows also the 13C-NMR spectrum of P3HP, the chemical shifts are similar to those obtained by previous study.Citation13
Effect of aeration on P3HP production
The aeration condition plays an important role in many metabolites production. For this reason, the effect of aeration on P3HP production in the recombinant strain Q1643 was examined by varying the shaking speed (50, 100, or 200 rpm) with a working volume 100 mL in a 250 mL flask culture (). With the speed of 50 rpm, the P3HP production and CDW were at the minimum level. With the increase of aeration, the P3HP production and cell density increased, and reached 0.24 g/L and 1.89 g/L respectively at the shaking speed of 100 rpm. At the same time, 2.03 g/L 3HP was produced under such aeration condition. The enhanced P3HP production could be attributed to improved 3HP production, and the enhanced 3HP production at higher aeration can be attributed to the higher aldH expression levels.Citation16 Compared with the cultures under poorer aeration conditions, the cell density (3.57 g/L) was improved dramatically under fully aerobic condition (200 rpm). In contrast, further enhanced aeration did not result in enhanced production, only trace amounts of P3HP (0.06 g/L) and a little 3HP (0.65) were produced. It was presumably caused by the repression of dha operon involving glycerol dehydrogenase, glycerol dehydratase and PDOR.Citation17,18
Table 1. Production of P3HP under different aeration
In the engineered metabolic pathway of this study, 3HP is the precursor of P3HP. However, under all the 3 aeration conditions, P3HP production is only about 10 percent of 3HP production. The quite low efficiency of the conversion from 3HP to P3HP might due to the low enzyme activity of PrpE. The similar phenomenon was observed when propionyl-CoA synthase expressed in Cupriavidus necator.Citation12 On the other hand, PrpE is the key enzyme in propionate catabolism which catalyzes propionate into propionyl-CoA. Compared with propionate, 3HP is maybe not a suitable substrate for PrpE. The modification of PrpE and using other propionyl-CoA synthetase from different sources like Salmonella typhimuriumCitation19 should be tried in the future for improving the production of P3HP.
Conclusion
K. pneumoniae strain was metabolically engineered and showed the potential of being able to produce P3HP from glycerol without external addition of coenzyme Vitamin B12. The aeration conditions played a vital role in P3HP production and cell growth. Under controlled aeration (100 rpm, 100 mL working volume in 250 mL non-baffled flasks), Q1643 produced 0.24 g/L P3HP which accounted for 12.7% content of cell dry. This study shows the feasibility of producing P3HP from glycerol using K. pneumoniae without vitamin B12 for the first time. Further investigation and metabolic engineering approaches are required to improve the P3HP yield in K. pneumoniae and make it commercially viable.
Materials and Methods
Strains and plasmids construction
All the bacterial strains, plasmids and primers are listed in . E. coli DH5α was used for plasmid cloning and maintenance. Standard recombinant DNA procedures were used for gene cloning and plasmid isolation. The gene aldH was amplified from E. coli BL21, and cloned into SacI and XbaI restriction sites of the pBAD33 vector to generate pBAD33-aldH. PCR fragment dhaB123-gdrAB was obtained by overlapping PCR after dhaB123 and gdrAB were amplified by PCR from the genomic DNA isolated from K. pneumoniae ATCC25955. The fragment was digested with XbaI and HindIII and then ligated between the same restriction sites of pBAD33-aldH to construct pEdg. The pBAD18-pp plasmid was constructed by inserting phaC-prpE fragment (enzymatic digestion with HindIII and SalI from pHP304 constructed by our previous studyCitation20) into pBAD18. The growth conditions for construction and the methods for developing K. pneumoniae mutant strains were carried out as described by our previous study.Citation4
Table 2. Bacterial strains, plasmids, and primers used in this study
Protein expression
To confirm that the recombinant strain had been properly constructed, the strains Q1533, Q1546, and Q1643 were cultured in LB medium at 37 °C and induced at an OD600 of 0.6~0.8 by adding 0.05% L-arabinose. The temperature was set at 30 °C after induced. The cells were harvested 3h after induction and centrifuged at 12000 rpm at 4 °C for 10min. The cell pellets were washed twice with 100 mM potassium phosphate buffer (pH7.0) and resuspended in the same buffer. The cells were lysed by Ultrasonic Cell Disruption System and centrifuged at 15000 rpm for 10min and the supernatants were used for SDS-PAGE. Protein expression was examined on SDS-PAGE under denaturing conditions and Coomassie brilliant blue was used to stain the proteins.
Shake flask cultivation
Shake flask cultivation was carried out with 100 mL working volume in 250 mL non-baffled flasks at 37 °C in an orbital incubator shaker at 150 rpm for producing 3HP. To examine the effect of aeration on the P3HP production, the recombinant strain Q1643 was examined by varying the shaking speed (50, 100, 200 rpm).
All the cultures were induced at 0.6~0.8OD600 with 0.05% (w/v) L-arabinose for producing targeted product. After induced, the temperature was set at 30 °C for further cultivation. Ammonia was supplemented for pH control every 12 h along with antibiotics added. 20 g/L glycerol was added into the medium after 24h. The preculture and fermentation medium used in this study contained the following components (per liter of deionized water): glycerol, 20 g; yeast extract, 3 g; citric acid, 0.42 g; KH2PO4, 2 g; K2HPO4, 1.6 g; NH4Cl, 5.4 g; MgSO4·7H2O, 0.2 g; and 1 mL of trace elements solution (FeCl3·6H2O, 5 g; MnCl2·4H2O, 2 g; ZnCl2, 0.684 g; CoCl2·6H2O, 0.476 g; CuCl2·2H2O, 0.17 g; H3BO3, 0.062 g; Na2MoO4·2H2O, 0.005 g; and 10 mL of concentrated HCl per liter).
Analytical methods
The samples were withdrawn to determine the 3HP and P3HP yield after induced 48 h. All shake experiments were carried out in triplicates. The concentration of 3HP was determined by HPLC using the method reported by Raj et al.Citation16 The cells were harvested after cultivation by centrifugation and lyophilized. PHA was extracted from the cells with hot chloroform in a Soxhlet apparatus for 10 h, and precipitated by ice-cold ethanol.Citation21 After ethanol and chloroform volatilized completely at room temperature, the polymer structure was analyzed by NMR using an Advanced III 600 NMR Spectrometer (Bruker, Switzerland). Both 13C NMR and 1H NMR spectra of the sample were measured at 25 °C in deuterated chloroform (CDCl3) (50 mg/mL) with Tetramethylisilane (TMS) as internal chemical shift standard.
Disclosure of Potential Conflicts of Interest
No potential conflicts of interest were disclosed.
Acknowledgments
We acknowledge Dr. Roy Curtiss III (Arizona State University) for strain χ7213 and pRE112, NBRP-E.coli at NIG for the clone vector pBAD18-kan.
Funding
This research was financially supported by the 100-Talent Project of CAS (for GZ), Natural Science Foundation of Shandong Province (ZR2013EMZ002), and Key Program of the Chinese 270 Academy of Sciences (KGZD-EW-606-1).
References
- Andreeßen B, Lange AB, Robenek H, Steinbüchel A. Conversion of glycerol to poly(3-hydroxypropionate) in recombinant Escherichia coli. Appl Environ Microbiol 2010; 76: 622-6; http://dx.doi.org/10.1128/AEM.02097-09
- Rossi DM, Souza EA, Ayub MAZ. Biodiesel residual glycerol metabolism by Klebsiella pneumoniae: pool of metabolites under anaerobiosis and oxygen limitation as a function of feeding rates. Appl Biochem Biotechnol 2013; 169(6): 1952-64; PMID:23354500; http://dx.doi.org/10.1007/s12010-013-0114-5
- Menzel K, Zeng AP, Deckwer WD. 1997. High concentration and productivity of 1,3-propanediol from continuous fermentation of glycerol by Klebsiella pneumoniae. Enzyme Microb Tech 1997; 20: 82-86; http://dx.doi.org/10.1016/S0141-0229(96)00087-7
- Feng X, Ding Y, Xian M, Xu X, Zhang R, Zhao G. 2014. Production of optically pure D-lactate from glycerol by engineered Klebsiella pneumonia strain. Bioresour Technol 2014; 172:269-75; PMID:25270041; http://dx.doi.org/10.1016/j.biortech.2014.09.074
- Blankschien MD, Clomburg JM, Gonzalez R. Metabolic engineering of Escherichia coli for the production of succinate from glycerol. Metab Eng 2010; 12:409-19; PMID:20601068; http://dx.doi.org/10.1016/j.ymben.2010.06.002
- Luo LH, Kim CH, Heo S, Oh B, Hong W, Kim S, Kim D, Seo J. 2012. Production of 3-hydroxypropionic acid through propionaldehyde dehydrogenase PduP mediated biosynthetic pathway in Klebsiella pneumoniae. Bioresour Technol 2012; 103:1-6; PMID:22018749; http://dx.doi.org/10.1016/j.biortech.2011.09.099
- Andreeßen B, Taylor N, Steinbüchel A. Poly(3-hydroxypropionate): a promising alternative fossil fuel-based materials. Appl Environ Microbiol 2014; 80(21):6574-82; http://dx.doi.org/10.1128/AEM.02361-14
- Shimamura E, Scandola M, Doi Y. Microbial syntheisis and characterization of poly(3-hydroxybutyrate-co-3-hydroxypropionate). Macromolecules 1994; 27(16): 4429-35
- Green PR, Kemper J, Schechtman L, Guo L, Satkowski M, Fiedler S, Steinbüchel A, Rehm B. Formation of short chain length/medium chain length polyhydroxyalkanoate copolymers by fatty acid β-oxidation inhibited Ralstonia eutropha. Biomacromolecules 2002, 3(1): 208-13; PMID:11866575; http://dx.doi.org/10.1021/bm015620m
- Kang CK, Lee HS, Kim JH. Accumulation of PHA and its copolyesters by Methylobacterium sp. KCTC 0048. Biotechnol Lett 1993; 15(10):1017-20; http://dx.doi.org/10.1007/BF00129929
- Meng D, Shi Z, Wu L, Zhou Q, Wu Q, Chen J, Chen G. Production and characterization of poly(3-hydroxypropionate-co-4-hydroxybutyrate) with fully controllable structures by recombinant Escherichia coli containing an engineered pathway. Metab Eng 2012; 14(4):317-24; PMID:22561235; http://dx.doi.org/10.1016/j.ymben.2012.04.003
- Fukui T, Suzuki M, Tsuge T, Nakamura S. Microbial synthesis of poly((R)-3-hydroxybutyrate-co-3-hydroxypropionate) from unrelated carbon sources by engineered Cupriavidus necator. Biomacromolecules 2009; 10(4): 700-6; PMID:19267466; http://dx.doi.org/10.1021/bm801391j
- Wang Q, Yang P, Liu C, Xue Y. Xian M, Zhao G. Biosynthesis of poly(3-hydroxypropionate) from glycerol by recombinant Escherichia coli. Bioresour Technol 2013; 131:548-51; PMID:23414748; http://dx.doi.org/10.1016/j.biortech.2013.01.096
- Ashok S, Sankaranarayanan M, Ko Y, Jae K, Ainala SK, Kuamr V, Park S. Production of 3-hydroxypropionic acid from glycerol by recombinant Klebsiella pneumoniae ΔdhaTΔyqhD which can produce vitamin B12 naturally. Biotechnol Bioeng 2013; 110(2):511-24; PMID:22952017; http://dx.doi.org/10.1002/bit.24726
- Ashok S, Raj SM, Rathnasingh C, Park S. Development of recombinant Klebsiella pneumoniae ΔdhaT strain for the co-production of 3-hydroxypropionic acid and 1,3-propanediol from glycerol. Appl Microbiol Biotechnol 2011; 90(4):1253-65; PMID:21336929; http://dx.doi.org/10.1007/s00253-011-3148-z
- Huang Y, Li Z, Shimizu K, Ye Q. Co-production of 3-hydroxypropioic acid and 1,3-propanediol by Klebsiella pneumonia expressing aldH under microaerobic conditions. Bioresour Technol 2013; 128:505-12; PMID:23201906; http://dx.doi.org/10.1016/j.biortech.2012.10.143
- Lin ECC, Levin AP, Magasanik B. The effect of aerobic metabolism on the inducible glycerol dehydrogenase of Aerobacter aerogenes. J Biol Chem 1960; 235:1824-9; PMID:14417008
- Forage RG, Lin EC. DHA system mediating aerobic and anaerobic dissimilation of glycerol in Klebsiella pneumoniae NCIN418. J Bacteriol 1982; 151(2):591; PMID:6284704
- Lee SK, Newman JD, Keasling JD. Catabolite repression of the propionate catabolic genes in Escherichia coli and Salmonella enterica: evidence for involvement of the cyclic AMP receptor protein. J Bacteriol 2005; 187(8): 2793-800; PMID:15805526; http://dx.doi.org/10.1128/JB.187.8.2793-2800.2005
- Wang Q, Liu C, Xian M, Zhang Y, Zhao G. Biosynthetic pathway for poly(3-Hydroxypropionate) in recombinant Escherichia coli. J Microbiol 2012; 50(4):693-7; PMID:22923122; http://dx.doi.org/10.1007/s12275-012-2234-y
- Lageveen RG, Huisman GW, Preusting H, Ketelaar P, Eggink G, Witholt B. Formation of polyesters by Pseudomonas oleovorans - Effect of substrates on formation and composition of poly-(R)-3-hydroxyalkanoates and poly-(R)-3-hydroxyal-kenoates. Appl Environ Microbiol 1998; 54:2924-32.