Abstract
Ashbya gossypii is a filamentous fungus that naturally overproduces riboflavin. Indeed, engineered strains are currently used for the industrial production of riboflavin, replacing the chemical synthesis processes formerly used. The utilization of A. gossypii for biotechnological applications affords significant advantages that involve low-cost media use and cheap downstream processing for some applications. Although A. gossypii cannot be considered a bona fide oleaginous microorganism, the accumulation of lipid droplets within hyphae has been described. In view of the genomic and molecular tools available for its manipulation, the metabolism of A. gossypii was engineered aiming to increase total lipid accumulation. Blocking the β-oxidation pathway through the knock-out of the AgPOX1 gene was sufficient to obtain strains with high lipid yields, comparable to those of the best oleaginous microorganisms. Thus, the poxΔ strain of A. gossypii constitutes a novel promising tool for the production of microbial oils in forthcoming modified A. gossypii strains.
Ashbya Gossypii as a Novel Organism for Microbial Oil Production
Our society is strongly dependent on crude oil for the production of fuels, fine chemicals, and many other commodities. However, fossil oil supplies are limited, and their use has negative environmental consequences.Citation1 Thus, in recent years new technologies have been developed for the production of both fuels and chemicals from renewable sources, such as plant oils, animal fats or microbial oils.
Microbial oils (also called single-cell oils, SCOs) have several advantages over other oil sources: i) the fermentation process of microorganisms is independent of season and climate; ii) many industrial by-products can be used as fermentation substrates; and iii) microorganisms can be efficiently modified via metabolic engineering approaches, hence allowing the optimization of the amount and properties of the SCOs.
In light of the above, biotechnological research has focused on oleaginous microorganisms, which can accumulate at least 20% of their cell dry-weight (CDW) as lipid content. Additionally, some oleaginous microorganisms can reach higher levels of lipid, accumulating up to 80% of their CDW when cultured in oil-containing media.Citation2 However, the biotechnological optimization of some oleaginous microorganisms for industrial applications is difficult owing to the lack of appropriate metabolic engineering tools. Accordingly, a potential alternative is the manipulation of industrial microorganisms that can be engineered to afford lipid yields comparable to oleaginous microorganisms. In this sense, some model organisms, such as Escherichia coli and Saccharomyces cerevisiae, have been engineered to overproduce lipids.Citation3-6
Ashbya gossypii is a filamentous hemiascomycete that is extensively used for the industrial production of riboflavin.Citation7-9 Indeed, the use of A. gossypii in industry is considered a paradigm of sustainable white biotechnology for the industrial overproduction of riboflavin and other vitamins.
Importantly, a large number of genomic, bioinformatic and biotechnological tools are available for A. gossypii,Citation10-13 thus allowing the development of systems metabolic engineering approaches to industrial applications of the fungus. In addition, the use of A. gossypii for microbial fermentation has other biotechnological advantages such as the ability to grow both in some industrial by-products and low-cost oils, the partial autolysis of hyphae at late growth phases, and the harvesting of mycelia by simple filtration. Moreover, A. gossypii can be grown by pellet fermentation, which reduces broth viscosity and improves mixing and mass transfer, saving both costs and energy.
A. gossypii has not been considered an oleaginous fungus because it is not able to accumulate more than 20% of its cell dry weight in the form of fatty acids. However, as previously mentioned the fungus can be manipulated to overproduce different metabolites. Thus, in our recent paper entitled “Strain Design of Ashbya gossypii for Single-Cell Oil Production” we described the generation of A. gossypii strains that were engineered to accumulate up to 70% of their CDW as lipids in glucose+oil-containing media (1% glucose + 2% oleic acid).Citation14 This represents a novel promising microbial platform for the production of single-cell oil.
Lipid accumulation in A. Gossypii
The concept of lipid accumulation refers to a metabolic imbalance between lipid biosynthesis and degradation. The biosynthesis of lipids mainly depends on a continuous supply of cytosolic acetyl-CoA, which is the substrate for the acetyl-CoA carboxylase (ACC) to form malonyl-CoA. Next, the fatty acid synthase (FAS) multienzymatic complex catalyzes the elongation of the acyl-CoA chain by condensing malonyl-CoA molecules and acetyl-CoA (). In this respect, oleaginous microorganisms are equipped with the enzyme ATP-citrate lyase, which converts citrate into cytosolic acetyl-CoA. Additionally, the biosynthesis of lipids also relies on a sufficient supply of NADPH as the essential reducing power for fatty acid biosynthesis.Citation15 In contrast to lipidogenesis, β-oxidation is the main catabolic pathway of fatty acids and, consequently, its activity competes with the accumulation of lipids. Beta-oxidation comprises a 4-step oxidative cycle that removes 2 carbons (an acetyl-CoA molecule) from the acyl-CoA chain in each cycle.Citation16
Figure 1. Simplified diagram of the lipid metabolism in A. gossypii. The ACL (ATP:citrate lyase) activity (gray) is not present in A. gossypii. ACS, acetyl-CoA synthase; ACC, acetyl-CoA carboxylase; FAS, fatty acid synthase; Pox1, fatty-acyl coenzyme A oxidase; Fox2, 3-hydroxyacyl-CoA dehydrogenase and enoyl-CoA hydratase; Fox3, 3-ketoacyl-CoA thiolase. The elongation system comprises the elongases ELO624 and ELO586 which elongate C18:0 fatty acids up to C26:0 (C > 18). The desaturation system is composed by 2 desaturases (Δ9 and DES589). Saturated fatty acids (Cn:0) can be desaturated by Δ9 to form Cn:1 fatty acids (where n is the number of carbons of the acyl chain). The Δ12 DES589 can further desaturate the Cn:1 fatty acids to form Cn:2 (mainly C18:2, linoleic acid). Dashed arrows indicate multistep pathways.
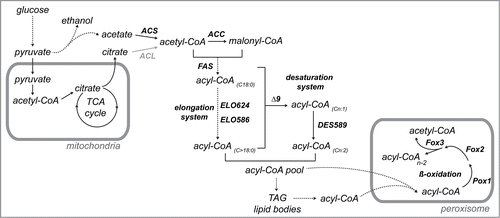
The wild-type strain of A. gossypii is able to accumulate about 5% of its CDW as lipids in glucose-rich media (8% glucose), while it can accumulate almost 20% of its CDW as lipids in glucose+oil-containing media (1% glucose + 2% oleic acid).Citation14 Lipid accumulation can be optimized both by increasing the availability of lipid precursor and by blocking the competing pathways of lipid accumulation.
A. gossypii lacks the enzyme ATP-citrate lyase, which is a hallmark of oleaginous microorganisms. In our work, A. gossypii was endowed with ATP-citrate lyase (ACL) activity through the heterologous expression of the ACL1 and ACL2 genes from Yarrowia lipolytica, which code for the 2 subunits of the ACL enzyme. Expression of the Y. lipolytica ACL genes in the wild-type strain of A. gossypii induced a 30% increase in lipid accumulation in glucose-rich medium (8% glucose) after 3 d of culture. Moreover, the β-oxidation pathway was abolished in A. gossypii through the deletion of the POX1 and FOX1 genes, which control the first and second steps of β-oxidation, respectively. The deletion of both the POX1 and FOX1 genes in A. gossypii did not exert any effect on biomass production either in glucose-rich medium or in glucose+oil-containing medium; however, growth was absent in soybean oil medium (2% soybean oil) (), indicating that the β-oxidation pathway supports growth from culture media with oil as the only carbon source. Accordingly, oil-containing medium for the quantification of lipid accumulation in either the pox1Δ or fox1Δ strains of A. gossypii must also include a non-lipid carbon source in their formulations (typically 1% glucose). The elimination of β-oxidation in A. gossypii resulted in a 2-fold increase in lipid accumulation both in glucose-rich and glucose+oil-containing media after 3 d of culture. However, the expression of the Y. lipolytica ACL enzyme in a pox1Δ strain did not show any increase in the lipid yield.
Figure 2. Effect of the abolition of the β-oxidation pathway on biomass production in A. gossypii. The wild-type strain of A. gossypii and the pox1Δ, fox1Δ and pox1Δ/fox1Δ mutants were grown in glucose-based media (2% glucose) (A) and soybean oil media (2% soybean-oil) (B). Biomass production (g/L) was measured over time.
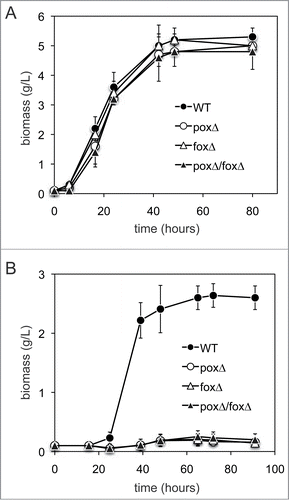
Regarding the levels of acetyl-CoA in the engineered strains, the expression of the ACL genes in the wild-type induced a significant increase in the intracellular acetyl-CoA pool (2-fold more acetyl-CoA). Interestingly, we found that the pox1Δ strain had levels of acetyl-CoA 4-times higher than those of the wild-type strain and, unexpectedly, the pox1Δ strain had higher levels of acetyl-CoA (approximately 2.5-fold higher) than the ACL-overexpressing strain. In addition, the pox1Δ strain showed a lower increase in acetyl-CoA levels (50% more acetyl-CoA) after combined expression of the ACL genes from Y. lipolytica. Accordingly, lipid accumulation was not correlated with the increase in acetyl-CoA levels in the different strains. Furthermore, the pox1Δ strain expressing ATP-citrate lyase did not show any increase in the total lipid content, indicating that an additional metabolic bottleneck exists after the limitation in acetyl-CoA synthesis has been overcome. The block in the β-oxidation pathway in the pox1Δ strain of A. gossypii was sufficient to trigger a huge increase in fatty acid accumulation in glucose+oil-containing medium. After 7 d of culture in glucose+oil-containing medium, the pox1Δ strain was able to accumulate up to 70% of the cell dry weight as lipid content (). Indeed, the pox1Δ strain showed a characteristic phenotype of its hyphae when growing on glucose + oil-containing media due to the high lipid accumulation (). In contrast, the pox1Δ strain showed a lower total fatty acid yield (about 60% of CDW) when it was engineered to overexpress both the ACL1 and ACL2 genes from Y. lipolytica.Citation14 This effect was inversely correlated with higher levels of acetyl-CoA in that strain, again supporting the idea that acetyl-CoA does not limit lipid accumulation under those conditions.
Figure 3. Lipid accumulation in the pox1Δ strain of A. gossypii. (A) Lipid quantification in A. gossypii WT and pox1Δ strains of cultures grown either in glucose-rich medium (8% glucose) or glucose+oil-containing medium (1% glucose + 2% oleic acid) for 7 d. The results are the means of 2 independent experiments performed in duplicate. Error bars represent standard deviations.(B) Micrographs of the WT and pox1Δ strains of A. gossypii grown either in glucose-rich medium or oil-containing medium for 7 d. Lipid bodies were stained with Bodipy (Molecular Probes BV) and visualized under fluorescence microscopy with a Universal Microscope Axioplan 2 imaging system (Carl Zeiss) using a 100× objective. The micrographs were taken using 488 nm excitation wavelength and a 490–535 nm long-pass emission filter.
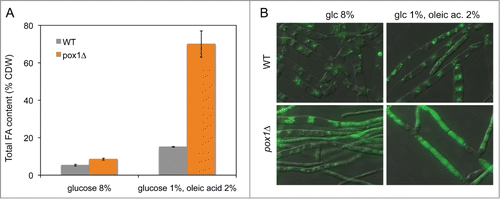
These results suggest that substrate limitation for lipid synthesis in A. gossypii can be partially prevented by the expression of ATP-citrate lyase. However, once acetyl-CoA levels are no longer a limiting factor for de novo lipid synthesis, other mechanisms seem to affect lipid production. The increased pool of intracellular acyl-CoA might be responsible for these limitations since it has been demonstrated that high levels of acyl-CoA can inhibit fatty acid synthesis in other microorganisms.Citation17,18 Accordingly, the enzymes involved in the esterification of the acyl-CoA to sterols or triacylglycerol might be good candidates to further manipulate the oleaginous nature of A. gossypii. Also, NADPH levels might not be sufficient to provide enough reducing power for an increased biosynthesis of fatty acids. Thus, a combined strategy aimed at increasing both the levels of acetyl-CoA and NADPH has been used for the production of fatty acid ethyl esters and polyhydroxybutyrate.Citation19-21
Many culture medium formulations for microbial fermentations are based on waste/low-cost sugars and it is therefore important to improve lipid accumulation from sugar-based media.Citation22 In this sense, the pox1Δ strains generated in our work were able to produce up to 9% of their CDW as lipids in rich glucose-rich medium (). Thus, although the total oil yield in A. gossypii growing in glucose-rich media is still low, it is significantly higher than that seen for some oleaginous yeasts such as engineered Y. lipolytica, which can accumulate up to 4.8 +/- 0.4% of its CDW as fatty acids in a similar rich medium (YPD).Citation23 Nonetheless, de novo lipid biosynthesis must be increased by optimized medium formulations and/or by additional metabolic engineering manipulations. For example, a wild-type strain of Y. lipolytica is able to attain 16.3% of its cell dry weight CDW as lipids under optimized conditions in glucose-based media and up to 88% of lipid content after extensive metabolic engineering.Citation24 Similarly, the oleaginous yeast Rhodosporidium toruloides accumulates up to 9.8% and 63% of its CDW as fatty acids in glucose-based media and in nitrogen-limited media respectively.Citation25 These comparisons suggest that oil production in A. gossypii from sugar-based media need to be further improved both by medium optimization (i.e. nitrogen-limited conditions) and by systems metabolic engineering.
Regarding the use of oil-containing media, the increased rate of lipid accumulation in such media suggests that the incorporation of extracellular lipids is a very active process in A. gossypii. This is worth noting within the context of the industrial process called oil biotransformation, which is the fermentative conversion of a cheap oil into a high-value oil. This process relies on cells’ ability to modify the extracellular fatty acids that have been incorporated into the cytosol. For example, the activity of fatty acid elongases can tune the length of fatty acids, while desaturases can catalyze the formation of double bonds within the acyl chain. Accordingly, we have recently characterized both the elongase and desaturase systems of A. gossypii.Citation26 Additionally, metabolic engineering has also been applied to both the elongase and desaturase systems with a view to generating novel A. gossypii strains that are able to accumulate polyunsaturated fatty acids, monounsaturated fatty acids, lubricants or components of waxes. Likewise, A. gossypii strains with optimized features for the production of biodiesel have also been generated. These strains lack both very long chain fatty acids and polyunsaturated fatty acids, which are undesired features in biodiesel blends.Citation26
Taking the above observations together, we propose A. gossypii as a novel and promising candidate for biolipid production that can offer an economically feasible alternative to some oleaginous yeasts. It has been demonstrated that modified strains of A. gossypii are able to accumulate high amounts of lipids and, additionally, it is possible to adapt the lipid profiles of such strains for them to become enriched in fatty acids of industrial relevance.Citation14,26 Nonetheless, more research is being carried out to increase lipid production from glucose-based media as well as to optimize the biotransformation of low-cost oils into high-value oils.
Disclosure of Potential Conflicts of Interest
No potential conflicts of interest were disclosed.
Acknowledgments
We thank MD Sánchez and S Domínguez for excellent technical help and N Skinner for correcting the manuscript.
Funding
This work was supported by grant BIO2011–23901 from the Spanish Ministerio de Economía y Competitividad. RLA and PL were recipients of FPU predoctoral fellowships from the Spanish Ministerio de Economía y Competitividad.
References
- Tuck CO, Pérez E, Horváth IT, Sheldon RA, Poliakoff M. Valorization of biomass: deriving more value from waste. Science (New York, NY) 2012; 337:695-9; http://dx.doi.org/10.1126/science.1218930
- Ageitos JM, Vallejo JA, Veiga-Crespo P, Villa TG. Oily yeasts as oleaginous cell factories. Appl Microbiol Biotechnol 2011; 90:1219-27; PMID:21465305; http://dx.doi.org/10.1007/s00253-011-3200-z
- Leber C, Da Silva NA. Engineering of Saccharomyces cerevisiae for the synthesis of short chain fatty acids. Biotechnol Bioeng 2014; 111:347-58; PMID:23928901; http://dx.doi.org/10.1002/bit.25021
- Lennen RM, Pfleger BF. Engineering Escherichia coli to synthesize free fatty acids. Trends Biotechnol 2012; 30:659-67; PMID:23102412; http://dx.doi.org/10.1016/j.tibtech.2012.09.006
- Tang X, Feng H, Chen WN. Metabolic engineering for enhanced fatty acids synthesis in Saccharomyces cerevisiae. Metab Eng 2013; 16C:95-102; http://dx.doi.org/10.1016/j.ymben.2013.01.003
- Wu H, Karanjikar M, San KY. Metabolic engineering of Escherichia coli for efficient free fatty acid production from glycerol. Metab Eng 2014; 25:82-91; PMID:25014174; http://dx.doi.org/10.1016/j.ymben.2014.06.009
- Jiménez A, Santos MA, Pompejus M, Revuelta JL. Metabolic engineering of the purine pathway for riboflavin production in Ashbya gossypii. Applied Environ Microbiol 2005; 71:5743-51; http://dx.doi.org/10.1128/AEM.71.10.5743-5751.2005
- Jiménez A, Santos MA, Revuelta JL. Phosphoribosyl pyrophosphate synthetase activity affects growth and riboflavin production in Ashbya gossypii. BMC Biotechnol 2008; 8:67; http://dx.doi.org/10.1186/1472-6750-8-67
- Mateos L, Jiménez A, Revuelta JL, Santos MA. Purine biosynthesis, riboflavin production, and trophic-phase span are controlled by a Myb-related transcription factor in the fungus Ashbya gossypii. Appl Environ Microbiol 2006; 72:5052-60; PMID:16820505; http://dx.doi.org/10.1128/AEM.00424-06
- Gattiker A, Rischatsch R, Demougin P, Voegeli S, Dietrich FS, Philippsen P, Primig M. Ashbya Genome Database 3.0: a cross-species genome and transcriptome browser for yeast biologists. BMC Genomics 2007; 8:9; PMID:17212814; http://dx.doi.org/10.1186/1471-2164-8-9
- Ledesma-Amaro R, Kerkhoven EJ, Revuelta JL, Nielsen J. Genome scale metabolic modeling of the riboflavin overproducer Ashbya gossypii. Biotechnol Bioeng 2014; 111:1191-9; PMID:24374726
- Santos MA, Mateos L, Stahmann KP, Revuelta JL. Insertional Mutagenesis in the Vitamin B2 Producer Fungus Ashbya gossypii. Methods in Biotechnology. Totowa, New Jersey: Taylor & Francis, 2004.
- Wendland J, Ayad-Durieux Y, Knechtle P, Rebischung C, Philippsen P. PCR-based gene targeting in the filamentous fungus Ashbya gossypii. Gene 2000; 242:381-91; PMID:10721732; http://dx.doi.org/10.1016/S0378-1119(99)00509-0
- Ledesma-Amaro R, Santos MA, Jiménez A, Revuelta JL. Strain design of Ashbya gossypii for single-cell oil production. Appl Environ Microbiol 2014; 80:1237-44; PMID:24317081; http://dx.doi.org/10.1128/AEM.03560-13
- Ratledge C. Fatty acid biosynthesis in microorganisms being used for single cell oil production. Biochimie 2004; 86:807-15; PMID:15589690; http://dx.doi.org/10.1016/j.biochi.2004.09.017
- Courchesne NM, Parisien A, Wang B, Lan CQ. Enhancement of lipid production using biochemical, genetic and transcription factor engineering approaches. J Biotechnol 2009; 141:31-41; PMID:19428728; http://dx.doi.org/10.1016/j.jbiotec.2009.02.018
- Black PN, Faergeman NJ, DiRusso CC. Long-chain acyl-CoA-dependent regulation of gene expression in bacteria, yeast and mammals. J Nutr 2000; 130:305S-9S; PMID:10721893
- Feddersen S, Neergaard TB, Knudsen J, Faergeman NJ. Transcriptional regulation of phospholipid biosynthesis is linked to fatty acid metabolism by an acyl-CoA-binding-protein-dependent mechanism in Saccharomyces cerevisiae. Biochem J 2007; 407:219-30; PMID:17593018; http://dx.doi.org/10.1042/BJ20070315
- Centeno-Leija S, Huerta-Beristain G, Giles-Gomez M, Bolivar F, Gosset G, Martinez A. Improving poly-3-hydroxybutyrate production in Escherichia coli by combining the increase in the NADPH pool and acetyl-CoA availability. Antonie Van Leeuwenhoek 2014; 105:687-96; PMID:24500003; http://dx.doi.org/10.1007/s10482-014-0124-5
- de Jong BW, Shi S, Siewers V, Nielsen J. Improved production of fatty acid ethyl esters in Saccharomyces cerevisiae through up-regulation of the ethanol degradation pathway and expression of the heterologous phosphoketolase pathway. Microb Cell Fact 2014; 13:39; PMID:24618091; http://dx.doi.org/10.1186/1475-2859-13-39
- Kocharin K, Siewers V, Nielsen J. Improved polyhydroxybutyrate production by Saccharomyces cerevisiae through the use of the phosphoketolase pathway. Biotechnol Bioeng 2013; 110:2216-24; PMID:23456608; http://dx.doi.org/10.1002/bit.24888
- Huang C, Chen XF, Xiong L, Chen XD, Ma LL, Chen Y. Single cell oil production from low-cost substrates: the possibility and potential of its industrialization. Biotechnol Adv 2013; 31:129-39; PMID:22960618; http://dx.doi.org/10.1016/j.biotechadv.2012.08.010
- Zhang B, Chen H, Li M, Gu Z, Song Y, Ratledge C, Chen YQ, Zhang H, Chen W. Genetic engineering of Yarrowia lipolytica for enhanced production of trans-10, cis-12 conjugated linoleic acid. Microb Cell Fact 2013; 12:70; PMID:23866108; http://dx.doi.org/10.1186/1475-2859-12-70
- Blazeck J, Hill A, Liu L, Knight R, Miller J, Pan A, Otoupal P, Alper HS. Harnessing Yarrowia lipolytica lipogenesis to create a platform for lipid and biofuel production. Nat Commun 2014; 5:3131; PMID:24445655; http://dx.doi.org/10.1038/ncomms4131
- Zhu Z, Zhang S, Liu H, Shen H, Lin X, Yang F, Zhou YJ, Jin G, Ye M, Zou H, et al. A multi-omic map of the lipid-producing yeast Rhodosporidium toruloides. Nat Commun 2012; 3:1112; PMID:23047670; http://dx.doi.org/10.1038/ncomms2112
- Ledesma-Amaro R, Santos MA, Jiménez A, Revuelta JL. Tuning single-cell oil production in Ashbya gossypii by engineering the elongation and desaturation systems. Biotechnol Bioeng 2014; 111:1782-91; PMID:24668265; http://dx.doi.org/10.1002/bit.25245