Abstract
D-xylonate dehydratase YjhG from Escherichia coli can convert D-xylonate into 2-keto-3-deoxy- D-xylonate (KDX), and is a key enzyme in the biosynthesis of 1,2,4-butanetriol and other chemicals. However, the biochemical properties of YjhG still remain unknown. In this study, the activity assay method for YjhG was established based on semicarbazide method, in which KDX reacts with semicarbazide reagent, and is further quantified by high-resolution mass spectrometry. The effect of reaction conditions on YjhG activity was determined in vitro using purified His-tagged YjhG protein. This enzyme showed maximal activity at 30°C and pH 8.0. Bivalent metal ions such as Mg2+ and Mn2+ activated, whereas Ni2+ and Zn2+ inhibited the activity of YjhG. Under optimal conditions, the Km and Vmax values were 4.88 mM and 78.62 μM l−1h−1, respectively, when using D-xylonate as a substrate. Amino acids sequence alignments and catalytic properties analysis revealed that YjhG might be a member of IlvD/EDD family. Results obtained in this study may lay a foundation for further investigation on YjhG and will benefit its application in biosynthesis of related chemicals.
Introduction
Global environmental awareness motivates scientific research to produce chemicals through microbial fermentation using renewable biomass.Citation1,2 In the biosyntheses of valuable chemicals like ethylene glycolCitation3 and 1,2,4-butanetriol,Citation4 YjhG from Escherichia coli was used to catalyze the dehydration of D-xylonate to form 2-keto-3-deoxy-D-xylonate (KDX). However, biochemical properties of YjhG still remain unknown. In order to enhance the efficiency of bioconversions, it is essential to spend some effort to determine its enzymatic properties.
The activity assay of D-xylonate dehydratase is difficult due to the unstability of KDX, an α-keto acid.Citation5 KDX has to be converted into some more stable derivative for its detection. Dahms et al reported an assay method of D-xylonate dehydratase activity on the basis of the semicarbazide-based detection of KDX.Citation6 Although this method is the major way for detect D-xylonate dehydratase activity and has been widely used, it lacks further optimization and the semicarbazied derivative of KDX cannot be quantified up to now. The establishment and optimization of KDX quantification assay will provide a foundation for high-throughput screening of D-xylonate dehydratase with higher activity.
In this study, the activity assay method of D-xylonate dehydratase was established and the reaction mechanism of KDX with semicarbazide reagent was proposed and further validated by high-resolution mass spectrometry (HRMS). D-xylonate dehydratase YjhG from E. coli was purified as His6-tagged fusion protein to study its enzymatic characteristics in vitro. What's more, protein sequence alignment revealed that YjhG could be a novel member of IlvD/EDD family. These results will benefit applications of YjhG protein in biosynthesis of related chemicals.
Results and Disscussions
Amino acids analysis of YjhG
Previous studies had reported some D-xylonate dehydratase from different microorganisms such as halophilic archaeon Haloferax volcanii,Citation7 and Caulobacter crescentus.Citation8 Though YjhG from E. coli was used in biosynthesis of ethylene glycol1 and 1,2,4-butanetriol as a putative D-xylonate dehydratse (EC 4.2.1.82), the identity was very low (lower than 30%) between YjhG and above mentioned D-xylonate dehydratase based on amino acid sequence blasts result. YjhG shares 87% and 86% protein sequence identities with dihydroxyacid dehydratase of Trabulsiella guamensis (accession no. KFB98064.1) and dehydratase of Sodalis sp. (accession number AHF78763.1), respectively. PSI-BLAST results also showed that the amino acid sequence of YjhG was similar to IlvD/EDD family proteins. The IlvD/EDD protein family includes the archetype dihydroxyacid dehydratase (ILvD, EC 4.2.1.9) and phosphogluconate dehydratase (EDD, EC 4.2.1.12). Multiple sequence alignment of YjhG amino acid sequences with several IlvD/EDD proteins demonstrated that 2 consensus segments, which have been proposed as conserved motifs for this protein family, were conserved in YjhG with a few modifications (regions X and Y in , respectively). This further indicated YjhG could be a novel member of IlvD/EDD family.
Establishment of YjhG activity assay method
YjhG is composed of 655 amino acid residues and have a molecular weight of 70 KDa just as shown in SDS-PAGE (). The purified YjhG enzyme was used for D-xylonate dehydratation, and semicarbazide method was used for product detection. Firstly, the protocol described by Dahms et al.Citation6 was used, but no absorbance could be detected after 30 min reaction. We presumed the low YjhG activity caused low KDX concentration which couldn't be monitored by spectrophotometer. Then the dilution procedure of reaction mixture was omitted, resulting in a detectable absorbance value. For further confirmation, the wavelength scanning (200–400 nm) was applied to reaction mixture (). Tough the change of absorption was found, absorbance value was still too low to be measured accurately. Optimization of enzyme reaction time was needed for an appropriate product amount. If the reaction time is too short, the reaction process is insufficient to accumulate detectable product. Conversely, the enzyme will lose its activity, causing the unauthentic activity assay. The relationship of product amount with reaction time was demonstrated in . In order to reach an obvious absorbance reading, the reaction time was chosen as 4 h. The wavelength scanning (200–400 nm) of a 4 h reaction sample was demonstrated in . 4 h was within the period of product linear growth, so this reaction time was appropriate for authentic activity assay and the amount of semicarbazide reagent was adequate for KDX detection.
Figure 2. SDS-PAGE analysis showing expression and purification of YjhG from E. coli. M - protein maker. 1 - control sample. 2 - soluble cell extract. 3 - purified protein sample.
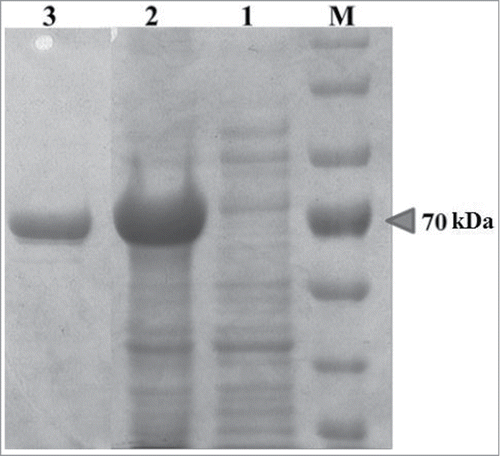
Figure 3. HRMS assay of semicarbazide derivative of D-xylonate dehydration product (KDX). (A) Wavelength scanning (200–400 nm) of 30 min reaction mixture, control sample (○), reaction sample (•). (B) Wavelength scanning (200–400 nm) of 4 h reaction mixture. (C) The reaction of KDX derivatization. (D) MS ion chromatogram for the KDX derivative assay. (E) Mass spectra of peak at 1.8 min from the MS ion chromatogram.
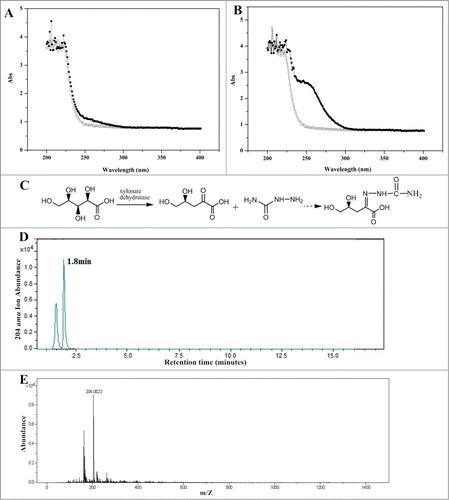
The product of D-xylonate dehydration, KDX, is a kind of α-keto acid, and is not commercially available because it is unstable.Citation5 Furthermore, it is difficult to separate the product from the reaction mixture using conventional methods such as preparative HPLC because its structure is relatively similar to the substrate. In order to detect KDX, its chemical conversion to more stable derivative was employed. The semicarbazide method was used for derivatization with the reaction mechanism as shown in , which was speculated from reactions of other keto acids with semicarbazide reagent. The molecular formula of derivative was estimated to be C6H11N3O5 with a molecular weight of 204.06 Da. HRMS (ESI−) analysis showed a new peak with a mass of 204.06 Da in reaction sample. MS ion chromatogram and mass spectra of peak at 1.8 min were shown in , respectively. What's more, there wasn't corresponding peak in control sample (YjhG was denatured). HRMS detection further confirmed the feasibility of semicarbazide-based method.
Enzymatic characterization of YjhG
The activity of YjhG was increased in the presence of bivalent metal ions such as Mn+ and Mg2+ (), as observed for dehydratases such as dihydroxy-acid dehydratase from E. coli.Citation9 Mg2+ has the highest catalytic role in this enzyme, which is in accordance with its observed catalytic role for other dehydratase.Citation10 The effects of Mg2+ and Mn2+ concentration on the enzyme activities were shown in , respectively. Co2+ and Cu2+ did not have significant influence on the enzyme activities, whereas Ni2+ and Zn2+ decreased enzyme activity. Ni2+ and Zn2+ belong to heavy mental ions which may denature protein. This result was different from D-gluconate dehydratase, whose activity was enhanced by Co2+ and Ni2+, but decreased by Zn2+ and Cu2+. Interestingly, Cu2+ had a negative effect on the activity of dihydroxy-acid dehydratase from E. coli, which might be in the same family with YjhG.Citation9
Figure 5. Catalytic properties of YjhG. (A) Effect of bivalent cations on the activity of YjhG. 100 % relative activity is 0.002 U as an absolute value. (B) Effect of Mg2+ concentration on the activity of YjhG. (C) Effect of Mn2+ concentration on the activity of YjhG. (D) Effect of temperature on the activity of YjhG. 100 % relative activity is 0.09 U as an absolute value. (E) Effect of buffer on the activity of YjhG. 100 % relative activity is 0.1 U as an absolute value. (F)Effect of pH on the activity of YjhG using 0.1 M Mes (▪), 0.1 M Hepes (•) and 0.1 M Bicine buffer (▴).
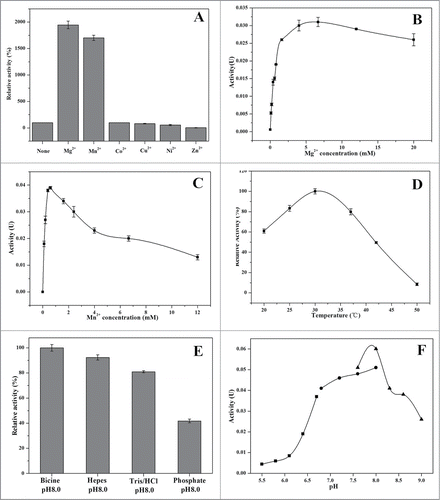
The effects of thiol compounds and sugars on the YjhG activity were given in . Unlike the D-gluconate dehydratase purified from Clostridium pasteurianum,Citation11 thiol compounds inhibited the enzyme activity of D-xylonate dehydratase.
Table 1 Effect of thiol reagents and sugars on YjhG activity
Purified YjhG enzyme displayed fairly stable activity between 20 and 60°C (). The enzyme activity was almost undetectable above 60°C, and the enzyme was denatured to precipitation above 70°C. The optimum temperature of YjhG was 30°C, which was near to the one of dihydroxy-acid dehydratase from E. coli.Citation12
The effects of buffers on YjhG were shown in . The lowest enzyme activity was observed in potassium phosphate buffer. This might be caused by the chelation of phosphates with cations like Mg2+ and Ca2+, while Mg2+ was of great importance in this reaction. With respect to pH optimization over the range pH 5.5–9.0, purified enzyme showed the optimal activity at pH 8.0 (). This optimum pH was the same as that of dihydroxy-acid dehydratase from E. coli.Citation12
The dependence of enzyme reaction rate on D-xylonate concentration followed Michaelis-Menten kinetics. Under optimal reaction conditions, the Km and Vmax values using D-xylonate as a substrate were 4.88 mM and 78.62 μM l−1h−1, respectively. Turnover number (kcat) was calculated to be 0.33 min−1, which yielded a kcat/Km value of 0.068 mM−1min−1. When using D-xylonate as the substrate, Km values of D-xylonate dehydratase from Haloferax volcanii and Pseudomonas sp are 0.55 mM and 2.98 mM, respectively.
Conclusion
YjhG activity assay method was established with modification of semicarbazide-based method and optimization of enzyme reaction time. In addition, the semicarbazide derivative of KDX was firstly deduced and further proved by HRMS detection. Properties of YjhG were systematically studied in vitro. This enzyme showed maximal activity at 30°C and pH 8.0. Some bivalent metal ions such as Mg2+ and Mn2+ activated, whereas others like Ni2+ and Zn2+ inhibited the activity. Under optimal reaction conditions, the Km and Vmax values were 4.88 mM and 78.62 μM l−1h−1, respectively, when using D-xylonate as a substrate. In addition, amino acids alignments analysis revealed that YjhG might be a member of IlvD/EDD family. These results lay a foundation for further investigation on YjhG. However, there is still much work to do for a complete understanding of this enzyme in the future. The enzyme activity is low when using D-xylonate as substrate, which restricts the biosynthesis of some chemicals. Consequently, gene modification can be used to improve the activity. Structure analysis is also worth doing for a complete understanding of YjhG.
Materials and Methods
Expression and purification of recombinant YjhG
The D-xylonate dehydratase gene (yjhG) was PCR-amplified from E. coli with primers yjhG-F (5′-CATGCCATGGCTATGTCTGTTCGCAATATTTTTGC-3′) and yjhG-R (5′-CCGGAATTCTCAGTTTTTATTCATAAAATCGCG-3′), and then cloned into plasmid pET30a with NcoI and EcoRI restriction sites. The recombinant plasmid was transformed into E. coli BL21 (DE3) and the strain was cultivated at 37°C, 180 rpm. 0.5 mM IPTG was added into the culture to induce the expression of plasmid gene at an OD600 ∼ 0.6.
Cells were harvested by centrifugation (5,000 ×g, 15 min, 4°C) and washed twice with 0.1 M Bicine (pH 8.0). Cell pellets were resuspended in 0.1 M Bicine (pH 8.0) and then disrupted by sonication. Crude extracts were centrifuged (20,000 ×g, 5 min, 4°C) to remove cell debris. The His6-tagged YjhG proteins were purified from the supernatant using Ni-NTA His·Bind Column (Novagen) according to the manufacturer's instruction. Samples selected with the purification procedure were monitored by SDS-PAGE. The samples containing D-xylonate dehydratase were pooled, concentrated and desalted using a super filter (Millipore MW 30 KD). Protein concentrations were determined by the method of Bradford using a calibration curve constructed with bovine serum albumin.
Establishment of YjhG activity assay method
The YjhG activity assay was conducted according to the previous semicarbazide-based method.Citation6 Modification with enzyme reaction time adapted to YjhG assay. Thus, different reaction times were analyzed with a range of 0–36 h to evaluate appropriate enzyme reaction time. The wavelength scanning (200–400 nm) was used to detect semicarbazide derivative of KDX (a peak of 250 nm). To further confirm the identity of KDX semicarbazide derivative, an enzyme reaction was performed and incubated with semicarbazide reagent at 30°C for 15 min. The control reaction was conducted using denatured enzyme. Final samples were detected by HRMS (ESI−).
Enzyme activity assay of YjhG
YjhG dehydratase activity was determined using modified semicarbazide-based method.Citation6,13 The standard assay method was performed as follows: the reaction mixture of total volume of 150 µl was incubated at 30°C in 33 mM Bicine buffer (pH 8.0), with 10 mM D-xylonate, 7 mM MgCl2 and enzyme solution. After 4 h, the enzyme reaction was quenched by adding 1 ml of semicarbizide solution (1.0 w/w semicarbazide hydrochloride and 1.5 w/w sodium acetate trihydrate dissolved in distilled water) and the mixture was incubated at 30°C for 15 min. Finally, the absorbance was read at 250 nm (molar absorption coefficient ϵ = 10200 M−1cm−1) in a 1 cm quartz cuvette against a reagent blank. Controls are necessary to correct for the absorbance of protein and other reaction components. The enzyme activity (1 unit) was defined as the amount of enzyme producing 1 µmol of 2-keto-3-deoxy-D-xylonate (KDX) per hour from D-xylonate under optimal assay conditions (30°C and Bicine buffer pH 8.0). All enzyme activities were determined in triplicate.
Enzyme characterization
The effects of bivalent metal ions (Zn2+, Mg2+, Cu2+, Co2+, Mn2+ and Ni2+) on YjhG were determined by measuring its enzyme activity in the presence of 5 mM bivalent ions.
The effects of thiol compounds (2-mercaptoethanol and dithiothreitol) and sugars (D-xylose, D-glucose, D-fructose, D-arabinose and D-mannose) on YjhG were determined by measuring its enzyme activity in the presence of 1 mM reagents.
The effects of buffers on YjhG were determined by measuring its enzymatic activity. The buffers used in this study were listed as followed: Bicine (pH 8.0), potassium phosphate (pH 8.0), Tris/HCl (pH 8.0) and Hepes (pH 8.0).
The temperature profile of the enzyme activity was determined between 20 and 50°C. The effect of pH on YjhG activity was determined at 30°C in 33 mM Mes buffer (pH 5.5–6.7), 33 mM Hepes buffer (pH 6.8–8.0) and 33 mM Bicine buffer (pH 7.6–9.0) by semicarbazide-based method.
Kinetic analysis was performed on pure enzyme using the standard assay with 0–14 mM D-xylonate. All experiments were performed in triplicate, and the apparent Vmax and Km value were calculated by fitting the initial-rate data to the Michaelis-Menten equation with a non-linear regression analysis program (Origin 8.5).
Disclosure of Potential Conflicts of Interest
No potential conflicts of interest were disclosed.
Funding
This work was financially supported by National Natural Science Foundation of China (21376255, 21202179); Key Program of the Chinese Academy of Sciences (KGZD-EW-606-1); 863 Program (No.SS2013AA050703-2); Qingdao Institute of Bioenergy and Bioprocess Technology Director Innovation Foundation for Young Scientists.
Reference
- Yim, H., Haselbeck R, Niu W, Pujol-Baxley C, Burgard A, Boldt J., Khandurina J, Trawick JD, Osterhout RE, Stephen R. Metabolic engineering of Escherichia coli for direct production of 1,4-butanediol. Nat Chem Bio 2011; 7:445–452; PMID:21602812; http://dx.doi.org/10.1038/nchembio.580
- Saxena R, Adhikari D, Goyal H. Biomass-based energy fuel through biochemical routes: a review. Renew Sust Energ Rev 2009; 13:167–178; http://dx.doi.org/10.1016/j.rser.2007.07.011
- Liu H, Ramos KRM, Valdehuesa KNG, Nisola GM, Lee WK, Chung WJ. Biosynthesis of ethylene glycol in Escherichia coli. Appl Microbiol Biotechnol 2012; 97:1–9.
- Valdehuesa KNG, Liu H, Ramos KRM, Park SJ, Nisola GM, Lee WK, Chung WJ. Direct bioconversion of d-xylose to 1, 2, 4-butanetriol in an engineered Escherichia coli. Process Biochem 2014; 49:25–32.
- Portsmouth D. Synthesis and properties of 3,6-dideoxyhexulosonic acids and related compounds: A convenient preparation of 3-deoxy-d-erythro-hexulosonic acid (3-deoxy-2-keto-d-gluconic acid). Stereoselectivity of nucleophilic addition to triose carbonyl. Carbohydr Res 1968; 8:193–204; http://dx.doi.org/10.1016/S0008-6215(00)80155-X
- Dahms AS, Donald A. d-xylo-aldonate dehydratase. Methods Enzymol 1982; 90:302–305; PMID:7154961
- Johnsen U, Dambeck M, Zaiss H, Fuhrer T, Soppa J, Sauer U, Schönheit P. D-xylose degradation pathway in the halophilic archaeon Haloferax volcanii. J Biol Chem 284:27290–27303; PMID:19584053
- Stephens C, Christen B, Fuchs T, Sundaram V, Watanabe K, Jenal U. Genetic analysis of a novel pathway for D-xylose metabolism in Caulobacter crescentus. J Bacteriol 2007; 189:2181–2185.
- Myers JW. Dihydroxy acid dehydrase: an enzyme involved in the biosynthesis of isoleucine and valine. J Biol Chem 1961; 236:1414–1418; PMID:13727223
- De Rosa M, Gambacorta A, Nicolaus B, Giardina P, Poerio E, Buonocore V. Glucose metabolism in the extreme thermoacidophilic archaebacterium Sulfolobus solfataricus. Biochem J 1984; 224:407–414; PMID:6440533
- Bender R, Gottschalk G. Purification and Properties of d-Gluconate Dehydratase from Clostridium pasteurianum. Eur J Biochem 1973; 40:309–321; PMID:4772682
- Duan X, Yang J, Ren B, Tan G., Ding H. Reactivity of nitric oxide with the [4Fe-4S] cluster of dihydroxyacid dehydratase from Escherichia coli. Biochem J 2009; 417:783–789; PMID:18945212
- MacGee J, Doudoroff M. A new phosphorylated intermediate in glucose oxidation. J Biol Chem 1954; 210:617–626; PMID:13211598