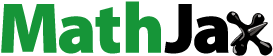
Abstract
In this paper, a miniaturized cell suspension concentration monitoring method was investigated. The sensing unit was a carbon screen-printed electrode (CSPE) in serial with a 433MHz vacuum-packaged surface acoustic wave resonator (SAWR). SAWR provided a stable and high operating frequency, which helps to keep the stability and sensitivity of the monitoring system. Living cells suspensions in different concentrations were prepared and dropcast on CSPE. Frequency responses of the sensor were recorded. Cell quantity variation within the same culture media volume changed the dielectric properties of CSPE and finally affected the SAWR frequency. SAWR frequency declined with the decrease of cell concentration. The proposed sensor provided high sensitivity and remarkable stability for the cell suspensions.
Introduction
Conventional methods, characterizing chemosensitivity and bio-behavior of the cells, become popular in cell pathology, chemotherapy, et al. Current methods usually need extensive experimental process and rigid laboratory conditions, exclusive of instrumentation and materials cost, such as radioactive therapy, fluorescent imaging method, et al.Citation1,2 It is difficult to conduct continuous sample monitoring for these methods, which makes it impossible to present the real-time changes of cell activities.
Cell-based sensor attracts much attention of many researchers in the past few decades. The living cells are used as sensing elements in these cases, which characterizes the agents effectively due to physiological or chemical reactions of the cells. This kind of sensor presents the advantages in sensitivity, simplicity, and low cost, etc.Citation3-6 The electrochemical impedance spectroscopy measurement has been applied in cell-based sensor research field successfully. It is first reported as electrical cell-substrate impedance sensing (ECIS) method for cell adhesion, spreading and motility monitoring.Citation7-9 The cells are cultured on 60-mm polystyrene culture dishes containing gold electrodes deposited by vacuum evaporation. Each dish contains one large (≈2 cm2) and 4 small electrodes (≈10–3cm2). The principles of this method lie in the attachment and spread of mammalian cells on the electrode surface. The cells can alternate the unrestricted current flow from the electrode into the bulk electrolyte and change the overall electrode impedance functionally. ECIS is utilized in drug screening,Citation10,11 toxicity evaluation,Citation12,13 and pharmacological apoptosis.Citation14 Liu et al measured the morphology changes of KYSE-30 cells adhesion, spreading, and proliferation by impedimetric analysis utilizing self-prepared micro-electrode array (MEA) device. Anti-cancer drug cisplatin has been used to cells. The results show that the cisplatin has chemosensitivity effects. Therefore, it is a promising method for cancer research.Citation15 The above-mentioned electrodes, such as small gold electrodes, MEA, et al, are relatively expensive, and not easy to prepare, which limits their applications in cell sensor research field. A disposable impedance sensor used for K562 leukemia cells adhesion and proliferation monitoring was proposed in 2007.Citation16 The screen-printed carbon electrode modified with polyaniline was prepared by electropolymerization as disposable impedance sensor. The electron transfer resistance of redox probe on electrode surface was affected by cells adhesion, which produced an impedance sensor for K562 leukemia cells with a semilogarithm linear range from 104 to 107 cells per ml and a limit of detection of 8.32×103 cells per ml at 10σ. The screen-printed carbon electrode as cell sensor carrier was simple, cheap and disposable and provided a convenient way for electrochemical cells sensor investigation. Usually, ECIS analysis technique demands instruments in bulky architecture and expensive devices, which hampered the prevalent use of this method. So it is of great importance to develop a reliable, miniaturized, and inexpensive way for real-time cell monitoring in vitro.
SAW device has attracted much attention due to its advantages including small size, high sensitivity, low cost and easily compatible with integrated circuit.Citation17-21 Some reports on chemical detections using SAW devices have been made.Citation22-24 The biosensor based on acoustic technique can potentially offer a repeatable, low cost and simple way to assay bacterial loads in complex liquids.Citation25 An ultra-high frequency SAW sensor (314.5MHz) for microbial count measurement in biological culture has been proposed in 2007.Citation26 A SAW resonator system (61MHz) and a pair of conductive electrodes in the feedback circuit with a RF amplifier have been proposed by Yao et al.Citation27-28 SAW impedance measurement has been applied in abundant analytical fields, such as urea determination,Citation29 pancreatic lipase detectionCitation30 and serum total salt concentration detection.Citation27
A high frequency SAWR for cell suspension concentration monitoring has been proposed in this study. living cells in different concentrations are dropcast on CSPE. The CSPE is serial with a 433MHz vacuum-packaged SAWR device. The principle unit is integrated on an electrical circuit board. Frequency information is sampled by an electrical counter and transmitted to a computer through a RS-232 cable. SAWR provides a higher operating frequency for the sensor, which can improve the sensitivity. The proposed sensor is miniaturized and relatively cheap comparing to previous studies.
Results and Discussion
Frequency responses of the serial SAWR sensor to cells suspensions in different concentrations under room temperature as the function of sampling time were shown in . The proposed sensor presented the discriminating ability of different cell suspension concentration. The SAWR frequency declined with the decrease of cell concentration, shown in . Linear fitting regression between SAWR frequency and cell suspension concentration were conducted, and the results were displayed in Equation (1). R = 0.95514 suggested that there was good linear relationship between these 2 parameters. After one step of transformation, Equation (2) was used for the characterization of cell suspension by SAWR frequency.
Figure 1. Frequency responses of Serial SAWR sensor: (a) to 1×106 cells/ml; (b) to 5×105 cells/ml; (c) to 2.5×105 cells/ml; (d) to 1.25×105 cells/ml; (e) to 1×105 cells/ml; (f) mean frequency as function of cell concentration.
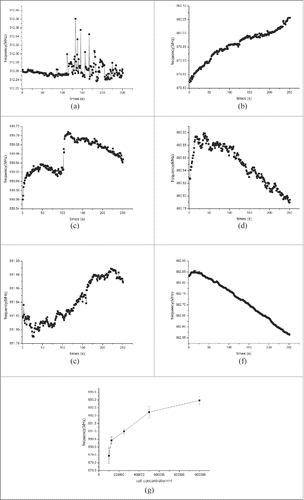
Cell suspension was dropcast between the working electrode and counter electrode. As the theoretical analysis, the Ce and Re of CSPE were modified. Because of the dielectric property of viable cells, the electric current between 2 electrodes was influenced by cell distribution. The larger cell concentration in culture media, the higher interelectrode resistance (Re) the CSPE presented. While interelectrode capacitance (Ce) change in this case could be almost ignored. So according to the equivalent circuit, a higher Re caused frequency to increase in SAWR oscillator, which can be a possible explanation for the results.
Methods
SAWR device and CSPE
The 433 MHz SAWR is used in our study. Two-port SAWR is fabricated on a ST-X cut quartz substrate with designed operating frequency of 433 MHz. The SAWR transducer is consisted of 30 pairs of electrodes in input and output interdigital transducers, 150 electrodes in each reflective array, an aperture width of 2000 μm and a periodicity of 7.29 μm. The electrode is made of aluminum film with thickness of 2000 Å. The standard photolithographic techniques with negative masks have been used to apply the SAWR pattern. A metal shell with drawn vacuum inside is used to make the SAWR device vacuum, which is shown in . In the serial topology, SAWR is utilized to perform the basic operating frequency for the sensor. Under vacuum condition, the device can provide a more stable basic frequency to improve the sensor's stability. Moreover, vacuum packaging can also prevent the device from corrosion and perturbation, and extend the sensor's lifespan.
Figure 2. SAWR device and CSPE: (a) SAWR and CSPE; (b) SEM image of CSPE; (c) cyclic voltammetry of CSPE.
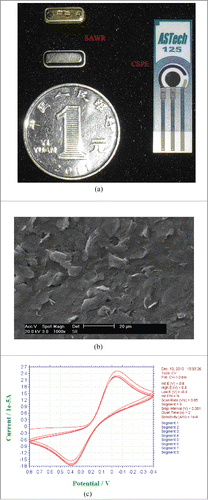
CSPE (ref. 110, 4 mm diameter) was purchased from ASTech (Jiangsu, China), which was all shown in . The potential values were referred to the screen-printed silver pseudo-reference electrode. The CSPE used in this paper is selected as the sensitive component, which has been widely used in electrochemical detection due to its easy fabrication, low cost and good stability. SEM (PHILIPS XL-30-ESEM, Netherlands) of CSPE was shown in , which indicated that the surface of CSPE was smooth and uniform. Cyclic-voltammetry scanning (CHI-1030 electrochemical workstation, USA) result of CSPE using 2mM K3[Fe(CN)6] solution was displayed in , which illuminated that this electrode was well-behaved in electrochemistry characteristic and fitted in our experiments. All other chemicals were of analytically pure grade or in good quality purchased from Sigma Aldrich.
Cell suspension
The human colorectal cells (purchased from ATCC, USA) are routinely maintained in minimum essential medium supplements with 5% fetal bovine serum together with penicillin and streptomycin. The cells are cultured on a 60mm diameter tissue culture dish in a humidified incubator at 37°C with 5% CO2.
We used microscope counting chamber to determine cell concentration. The result showed that the cell concentration was about 1×106 viable cells per ml. The cell suspension was diluted down to 5×105, 2.5×105, 1.25×105, 1×105 cells per ml for our investigation.
Model and principle and testing system
The cell suspension of 100 μl was dropcast on CSPE, covering the working electrode (WE), counter electrode (CE), and reference electrode (RE). The schematic figure of modified CSPE was shown in . The SAWR was used as frequency selective component to form a positive feed oscillating circuit with other discrete components. The equivalent circuit of CSPE in serial with SAWR was shown in . In the topological structure, C0 is the shunt static capacitance, Ls, Cs and Rs are motional inductance, motional capacitance, and motional resistance of SAWR respectively. A CSPE is added in serial with SAWR. Ce and Re are the equivalent capacity and resistor of the CSPE respectively. The viable cells dispensing in culture media modify the dielectric properties (Ce and Re) of CSPE, which affected the frequency of the serial SAWR resonator board as a result.
Figure 3. The testing system: (a) modified CSPE; (b) equivalent circuit of SAWR in serial with CSPE; (c) schematic diagram of detecting system.
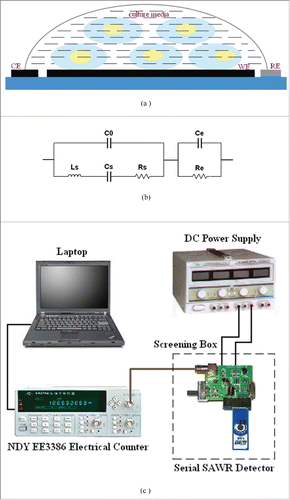
The SAWR and CSPE are connected in series to form a frequency selective network. Its resonance frequency F is defined as follows:(3)
(3) Here,
is the basic operating frequency of the sensor defined by SAWR.
is a phase parameter of the amplifier circuit.
is surface conductance of the CSPE. Interelectrode capacitance
, among which
is dielectric constant and
is the parasitic capacitance between connection wires. The SAWR is vacuum packaged and its equivalent parameter remains stable under detecting conditions. Resonance frequency of this design is mainly decided by
and
of the CSPE. Thus the substances spreading on the working electrode of CSPE, such as living cells, are more suitable for this design. The spreading of living cells in different concentrations on working electrode of CSPE lead to a different variance of
and
of CSPE emerges. As a result, a different resonance frequency
can be obtained. The design can also avoid the difficulty to measure a large resistance of the CSPE.
The schematic diagram of testing system is shown in . A positive feedback oscillation circuit is designed to generate an oscillator signal. The modified CSPE is serial with SAWR via a compatible interface, which locates on an integrated circuit board. The frequency signal is sampled by NDY EE3386 electrical counter (Nanjing NDY communication, Jiangsu, China). The sensing unit (including CSPE and SAWR) was placed in a screening box to avoid the electromagnetic interference, which was powered by DC power supply (Zhongce Electronic Co., Ltd, Zhejiang, China). The serial SAWR detector was shielded in a screening box to avoid the electro-magnetic interference.
Conclusions
A miniaturized cell suspension concentration monitoring system was proposed in this paper. The main sensitive component was a CSPE in serial with a vacuum-packaged 433MHz SAWR. The intestinal cells suspensions in different concentrations were prepared and dropcast on CSPE. The cell suspensions in different concentrations induced the changes of CSPE's Ce and Re, and affected the SAWR frequency. The SAWR frequency declined with the decrease of cell concentration. The results showed that the proposed sensor can provide good sensitivity and stability for cell suspensions. The cell suspension concentration detecting model was . SAWR can minimize the size of the detecting system if possible, and CSPE also enjoys some unique advantages, such as simplicity, low cost, portability and disposability, et al. Therefore, the proposed method is promising in cell-based sensor investigation.
Disclosure of Potential Conflicts of Interest
No potential conflicts of interest were disclosed.
Funding
The Project was supported by the Natural Science Foundation of Zhejiang province, China (Grant No. LQ14F020014, LY15F020034, LQ13F020013, LY15C140005), Key Laboratory of Forestry Intelligent Monitoring and Information Technology Research of Zhejiang Province (project number: 100151402, 100151401), National Natural Science Foundation of China (Grant No. 61272313, 61302185), Scientific Research Foundation and Talent Development Foundation of Zhejiang A & F University (Grant No. 2012FK028, 2012FR031).
References
- Liu Y, Peterson DA, Kimura H, Schubert D. Mechanism of cellular 3-(4, 5-dimethylthiazol-2-yl)-2, 5-diphenyltetrazolium bromide (MTT) reduction. J Neurochem 1997; 69:581-93; PMID:9231715; http://dx.doi.org/10.1046/j.1471-4159.1997.69020581.x
- Mosmann T. Rapid colorimetric assay for cellular growth and survival: application to proliferation and cytotoxicity assays. J Immunol Methods 1983; 65:55-63; PMID:6606682; http://dx.doi.org/10.1016/0022-1759(83)90303-4
- Bousse L. Whole cell biosensors. Sens Actuators B: Chem 1996; 34:270-5; http://dx.doi.org/10.1016/S0925-4005(96)01906-5
- Pancrazio JJ, Whelan JP, Borkholder DA, Ma W, Strenger DA. Development and application of cell-based biosensors. Ann Biomed Eng 1999; 27:697-711; PMID:10625143; http://dx.doi.org/10.1114/1.225
- Wang, P, Xu, G, Liu, Q, Xu, Y, Li, Y, Li, R. Cell-based biosensors and its application in biomedicine. Sens Actuators: Chem 2005; 10:576-84.
- Yu J, Jha SK, Xiao L, Liu Q, Wang P, Sury C, Yang M. AlGaN/GaN heterostructures for non-invasive cell electrophysiological measurements. Biosens Bioelectron 2007; 23:513-9; PMID:17766103; http://dx.doi.org/10.1016/j.bios.2007.06.014
- Giaever I, Keese CR. Monitoring fibroblast behavior with an applied electric field. Proc Natl Acad Sci USA 1984; 81:3761-4; PMID:6587391; http://dx.doi.org/10.1073/pnas.81.12.3761
- Giaever I, Keese CR. Use of electric fields to monitor the dynamical aspect of cell behavior in tissue culture. Chemtech 1986; 22:116-25.
- Giaever I, Keese CR. A morphological biosensor for mammalian cells. Nature 1993; 366:591-2; PMID:8255299; http://dx.doi.org/10.1038/366591a0
- Asphahani F, Zhang M. Cellular impedance biosensors for drug screening and toxin detection. Analyst 2007; 132:835-41; PMID:17710258; http://dx.doi.org/10.1039/b704513a
- Yin HY, Wang FL, Wang AL, Cheng J, Zhou YX. Bioelectrical Impedance Assay to Monitor Changes in Aspirin-Treated Human Colon Cancer HT-29 Cell Shape during Apoptosis. Anal Lett 2007; 40:85-94; http://dx.doi.org/10.1080/00032710600952424
- Ceriotti L, Ponti J, Broggi F, Kob A, Drechsler S, Thedinga E, Colpo P, Sabbioni E, Ehret R, Rossi F. Real-time assessment of cytotoxicity by impedance measurement on a 96-well plate. Sensors and Actuators B: Chemical 2007; 123: 769-78; http://dx.doi.org/10.1016/j.snb.2006.10.024
- Curtis TM, Widder MW, Brennan LM, Schwager SJ, van der Schalie WH, Fey Julien, Salazar Noe. A portable cell-based impedance sensor for toxicity testing of drinkingwater. Lab Chip 2009; 9:2176-83; PMID:19606294; http://dx.doi.org/10.1039/b901314h
- Kloß D, Fischer M, Rothermel A, Simon JC, Robitzki AA. Drug testing on 3D in vitro tissues trapped on a microcavity chip. Lab Chip 2008; 8:879-884.
- Liu Q, Yu J, Xiao L, Tang J, Zhang Y, Wang P, Yang M. Impedance studies of bio-behavior and chemosensitivity of cancer cells by micro-electrode arrays. Biosens Bioelectron 2009; 24:1305-10; PMID:18783935; http://dx.doi.org/10.1016/j.bios.2008.07.044
- Ding L, Du D, Wu J, Ju H. A disposable impedance sensor for electrochemical study and monitoring of adhesion and proliferation of K562 leukaemia cells. Electrochem Commu 2007; 9:953-8; http://dx.doi.org/10.1016/j.elecom.2006.11.040
- Wohltjen H, Dessy R. Surface Acoustic Wave Probe for Chemical Analysis. I. Introduction and Instrument Description. Anal Chem 1979; 51:1458-64.
- Hoyt AE, Ricco AJ, Bartholomew JW, Osbourn GC. SAW sensors for the room-temperature measurement of CO2 and relative humidity. Anal Chem 1998; 70:2137-45; http://dx.doi.org/10.1021/ac971095z
- Caliendo C, Fratoddi I, Russo MV. Sensitivity of a platinum-polyyne-based sensor to low relative humidity and chemical vapors. Appl Phys Lett 2002; 80:4849-51; http://dx.doi.org/10.1063/1.1489095
- Shen CY, Huang CP, Huang WT. Gas-detecting properties of surface acoustic wave ammonia sensors. Sens Actuators: Chem 2004; 101:1-7; http://dx.doi.org/10.1016/j.snb.2003.07.016
- Atashbar MZ, Sadek AZ, Wlodarski W, Sriram S, Bhaskaran M, Cheng CJ, Kaner RB, Zadeh KK. Layered SAW gas sensor based on CSA synthesized polyaniline nanofiber on AlN on 64 YX LiNbO3 for H2 sensing. Sens Actuators: Chem 2009; 138:85-9; http://dx.doi.org/10.1016/j.snb.2009.01.072
- Leidl A, Hartinger R, Ruth M, Endres HE. A new SO2 sensor system with SAW and IDC elements. Sens Actuators: Chem 1996; 34:339-42; http://dx.doi.org/10.1016/S0925-4005(96)01825-4
- Du XS, Ying ZH, Jiang YD, Liu ZX, Yang TJ, Xie GZ. Synthesis and evaluation of a new polysiloxane as SAW sensor coating for DMMP detection. Sens Actuators: Chem 2008; 134:409-13; http://dx.doi.org/10.1016/j.snb.2008.05.016
- Mashat LA, Tran HD, Wlodarski W, Karner RB, Zadeh KK. Polypyrrole nanofiber surface acoustic wave gas sensors. Sens Actuators: Chem 2008; 134:826-31; http://dx.doi.org/10.1016/j.snb.2008.06.030
- Gardner J, Cole M, Dowson C, Newton P, Sehra G. Smart acoustic sensor for the detection of bacteria in milk. Proc Int Conf Biomed Eng 2005; 458:168.
- Chang KS, Chang CK, Chen CY. A surface acoustic wave sensor modified from a wireless transmitter for the monitoring of the growth of bacteria. Sens Actuators: Chem 2007; 125:207-13; http://dx.doi.org/10.1016/j.snb.2007.02.007
- Yao S, Chen K, Zhu F, Shen D, Nie L. Surface acoustic wave sensor system for the determination of total salt content in serum. Anal Chim Acta 1994; 287:65-73; http://dx.doi.org/10.1016/0003-2670(94)85102-6
- Yao S, Chen K, Nie L. Application of a surface acoustic wave sensor system for the determination of non-aqueous solutions and phase transitions in lipid multibilayers. Anal Chim Acta 1994; 289:47-55; http://dx.doi.org/10.1016/0003-2670(94)80007-3
- Liu D, Chen K, Ge K, Nie L, Yao S. Study on the urease activity in different plant seeds and clinical analysis of urea in human body fluids by a surface acoustic wave enzyme sensor system. Fresenius J Anal Chem 1996; 354:114-7; http://dx.doi.org/10.1007/s002169600019
- Ge K, Liu D, Chen K, Nie L, Yao S. Assay of pancreatic lipase with the surface acoustic wave sensor system. Anal Biochem 1995; 226:207-11; PMID:7793619; http://dx.doi.org/10.1006/abio.1995.1215