“Perfection is finally attained not when there is no longer anything to add but when there is no longer anything to take away.” Antoine de Saint-Exupery (1900-1944)
While Douglas Adams' answer to the ultimate question of life is 42Citation1, the real answer might be closer to 473 – the minimum number of genes required to sustain life under ideal environmental conditions.Citation2 The search for the minimum genome, began in earnest in the early 1980s when MorowitzCitation3 first proposed the mycoplasmas (owing to their parsimonious genomes) as an ideal model for understanding the basic principles of life. Indeed, the smallest known mycoplasma genome (Mycoplasma genitaliumCitation4; containing 525 genes) provided the most obvious starting point from which to determine the minimal genome. Comparison of the M. genitalium genome to that of H. influenzae (the first bacterial genome sequencedCitation5; 1815 genes), revealed a common core of 256 genes; representing a theoretical minimal genomeCitation6 which is less than half that of M. genitalium. Further experimental analysis involving global transposon mutagenesis of the M. genitalium genome predicted a minimal genome of 375 essential genes; significantly more than the theorized minimum, yet less than that found in nature, providing scope for the design and development of a minimal artificial genome.Citation7-9
Following the successful creation of JCVI-syn1.0,Citation10 Venter (our esteemed Associate Editor) and colleagues set out to design and build a significantly stripped back version of syn1.0, using a novel design-build-test (DBT) strategy. In all, 4 DBT cycles were required to generate JCVI-syn3.0 – a working approximation of a minimal synthetic cell.Citation2
The first DBT cycle involved the design of a hypothetical minimal genome (HMG), based on existing transposon mutagenesis data and the published literature.Citation11,12 The resulting HMG design contained 432 protein encoding genes and 39 RNA genes. Using the modular strategy described previously,Citation10 the HMG was divided into 8 overlapping segments, each of which has a corresponding syn1.0 segment. This approach allows designed synthetic segments to be mixed and matched with viable syn1.0 segments enabling assembly in any specified combination. However, this initial approach, being based on inadequate transposon mutagenesis data, had only limited success; with only one of the HMG segment designs producing viable cells.
The second DBT cycle involved a refined Tn5 strategy to improve the HMG design; generating ∼80,000 clones, each containing a Tn5 chromosomal insertion. Approximately 30,000 unique insertions were tagged in this initial screen and the identified genes were divided into 3 categories, classified as either essential (“e-genes”; of which there were 240), non-essential (“n-genes”; 432) or quasi-essential (“i-genes”; 229). The “i-gene” group was further subdivided as “in-genes” (those exhibiting a minimal growth defect when mutated) and “ie-genes” (those exhibiting severe growth defects). The reduced genome design (RGD1.0) removed ∼90% of the “n-genes,” resulting in a ∼50% reduction in the syn1.0 genome size. Using the same cloning strategy as before, the 8 segments of RGD1.0 were introduced into the seven-eighths syn1.0 background in yeast, before being transformed into M. capricolum. Interestingly, despite each designed segment supporting cell growth in a seven-eighths syn1.0 background, combining all 8 reduced RGD1.0 segments into a single genome failed to produce a viable cell when transplanted into M. capricolum. This limitation is due to the existence of “synthetic lethal pairs”Citation13; the combined loss of redundant genes for essential functions as a consequence of the modular cloning strategy employed. While, 2 segments each containing an “e-gene” will support growth in the context of the seven-eighths syn1.0 background, when the segments are combined both “e-genes” are excluded and the cell fails to grow (or in the case of “i-genes;” grows more slowly). Taking into account the existence of synthetic lethal pairs; 26 genes were added to RGD1.0 to give rise to RGD2.0. A third DBT cycle (with 7 RGD2.0 segments plus a syn1.0 segment with 31 genes deleted) gave rise to JCVI-syn2.0; the first synthetic minimized cell with a genome smaller than M. genitalium.
Finally, a fourth round of Tn5 mutagenesis on syn2.0 stripped an additional 42 “n-genes” from RGD2.0, to give RGD3.0. As before, the 8 newly designed RGD3.0 segments were propagated in yeast before being transplanted into M. capricolum. One of these resulting viable transplants was chosen as the representative minimal synthetic cell and named JCVI-syn3.0. However, creation of the minimal genome is both a subjective and dynamic process; affected by intrinsic and extrinsic factors. While additional RGB cycles would likely strip the syn3.0 genome even further, growth rate would almost certainly be compromised. Furthermore, environmental factors, such as the nutritional quality of the surrounding medium will dictate the minimum genetic requirement. Therefore, while syn3.0 may not be the smallest possible synthetic construct, it is the most physiologically stable under the environmental conditions prescribed by Hutchison et al.Citation2
At the genomic level, all 4 DBT cycles successfully stripped 428 genes from the syn1.0 template, leaving a 531 kbp genome composed of 473 genes. A majority of the identified genes can be grouped into 4 main categories: dedicated to gene expression, genome preservation, membrane structure/function, and cytosolic metabolism. While almost half of the genome (48%; 229 genes) is dedicated to gene expression (195 genes) and genome preservation (34 genes), approximately equal numbers of genes are involved in directing cellular architecture (18%; 84 genes) and metabolism (17%; 81 genes). Significantly, the remaining 79 genes (17% of the genome) cannot easily be assigned to a functional category. Of these, 19 are “e-genes,” 36 are “i- or ie-genes” and 24 are “n- or in-genes.” While most of these genes can be expected to fall into one of the 4 major categories, described above, at least some are likely to direct previously undescribed biological functions.
Phenotypically, syn3.0 has a similar colony morphology to that of its progenitor cell, syn1.0, albeit with a smaller colony size, suggesting a slower growth rate. Indeed, syn3.0 grows approximately 3 times slower than syn1.0 (doubling time of ∼180 min as opposed to ∼60 min). Furthermore, unlike syn1.0 which grows as predominantly planktonic cells in static liquid culture, syn3.0 forms matted sediments under similar conditions. Additionally, microscopic analysis of syn3.0 under these conditions reveals the existence of long, segmented filamentous structures, together with large vesicular bodies.
Figure 1. Electron micrographs of clusters of JCVI-syn3.0 cells magnified ∼15,000 times. Courtesy of Tom Deerinck and Mark Ellisman of the National Center for Imaging and Microscopy Research at the University of California at San Diego.
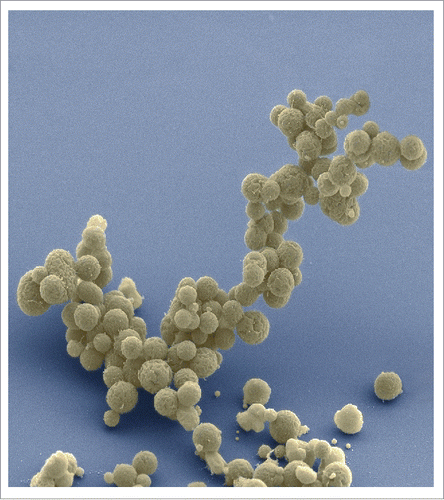
Having created the smallest synthetic cell – what next for this branch of synthetic biologyCitation14? Some have questioned the utility of the minimal genome approach, particularly against the backdrop of significant recent advances in CRISPR gene editing.Citation15 Why design synthetic life when it is possible, and in most cases more convenient, to edit existing natural genomes?Citation16 Venter's response to such queries is simple - “if you're trying to design life, CRISPRs aren't going to get you there”.Citation15 A significant aim of the JCVI minimal genome project is not simply to create life, but to improve it, through the design and delivery of safe and effective cellular chassis. These minimal biological frameworks, in which the function of each gene is known, will facilitate the development of improved whole-cell computational models.Citation17,18 Such models will enable efficient in silico design and testing (analogous to CAD based systems in traditional engineering), before undertaking wet-lab based development. The potential outputs from this approach include tailored microbes with myriad applications in industry, agriculture and medicine.Citation19-21 Moreover, this app-roach also paves the way for the development of microbes with altered or expanded genetic codes,Citation22,23 and as living repositories for big data storage.Citation24
The future is bright for synthetic biology, the future is synX.0!
Disclosure of potential conflicts of interest
No potential conflicts of interest were disclosed.
Acknowledgments
RDS is Co-ordinator of the EU FP7 project ClouDx-i.
References
- Adams D. The hitchhiker's guide to the galaxy. New York: Harmony Books, 1980.
- Hutchison CA, 3rd, Chuang RY, Noskov VN, Assad-Garcia N, Deerinck TJ, Ellisman MH, Gill J, Kannan K, Karas BJ, Ma L, et al. Design and synthesis of a minimal bacterial genome. Science 2016; 351:aad6253; PMID:27013737; http://dx.doi.org/10.1126/science.aad6253
- Morowitz HJ. The completeness of molecular biology. Israel J Med Sci 1984; 20:750-3; PMID:6511349
- Fraser CM, Gocayne JD, White O, Adams MD, Clayton RA, Fleischmann RD, Bult CJ, Kerlavage AR, Sutton G, Kelley JM, et al. The minimal gene complement of Mycoplasma genitalium. Science 1995; 270:397-403; PMID:7569993; http://dx.doi.org/10.1126/science.270.5235.397
- Fleischmann RD, Adams MD, White O, Clayton RA, Kirkness EF, Kerlavage AR, Bult CJ, Tomb JF, Dougherty BA, Merrick JM, et al. Whole-genome random sequencing and assembly of Haemophilus influenzae Rd. Science 1995; 269:496-512; PMID:7542800; http://dx.doi.org/10.1126/science.7542800
- Mushegian AR, Koonin EV. A minimal gene set for cellular life derived by comparison of complete bacterial genomes. Proc Natl Acad Sci U S A 1996; 93:10268-73; PMID:8816789; http://dx.doi.org/10.1073/pnas.93.19.10268
- Hutchison CA, Peterson SN, Gill SR, Cline RT, White O, Fraser CM, Smith HO, Venter JC. Global transposon mutagenesis and a minimal Mycoplasma genome. Science 1999; 286:2165-9; PMID:10591650; http://dx.doi.org/10.1126/science.286.5447.2165
- Glass JI, Assad-Garcia N, Alperovich N, Yooseph S, Lewis MR, Maruf M, Hutchison CA, 3rd, Smith HO, Venter JC. Essential genes of a minimal bacterium. Proc Natl Acad Sci U S A 2006; 103:425-30; PMID:16407165; http://dx.doi.org/10.1073/pnas.0510013103
- Sleator RD. The story of Mycoplasma mycoides JCVI-syn1.0: the forty million dollar microbe. Bioengineered Bugs 2010; 1:229-30; PMID:21327053
- Gibson DG, Glass JI, Lartigue C, Noskov VN, Chuang RY, Algire MA, Benders GA, Montague MG, Ma L, Moodie MM, et al. Creation of a bacterial cell controlled by a chemically synthesized genome. Science 2010; 329:52-6; PMID:20488990; http://dx.doi.org/10.1126/science.1190719
- Suzuki Y, Assad-Garcia N, Kostylev M, Noskov VN, Wise KS, Karas BJ, Stam J, Montague MG, Hanly TJ, Enriquez NJ, et al. Bacterial genome reduction using the progressive clustering of deletions via yeast sexual cycling. Gen Res 2015; 25:435-44; PMID:25654978; http://dx.doi.org/10.1101/gr.182477.114
- Forster AC, Church GM. Towards synthesis of a minimal cell. Mol Systems Biol 2006; 2:45; PMID:16924266; http://dx.doi.org/10.1038/msb4100090
- Dobzhansky T. Genetics of Natural Populations. Xiii. Recombination and Variability in Populations of Drosophila Pseudoobscura. Genetics 1946; 31:269-90
- Sleator RD. The synthetic biology future. Bioengineered 2014; 5:69-72; PMID:24561910; http://dx.doi.org/10.4161/bioe.28317
- Callaway E. 'Minimal' cell raises stakes in race to harness synthetic life. Nature 2016; 531:557-8; PMID:27029256; http://dx.doi.org/10.1038/531557a
- Urnov F. Genome editing: The domestication of Cas9. Nature 2016; 529:468-9; PMID:26819037; http://dx.doi.org/10.1038/529468a
- Sleator RD. Digital biology: a new era has begun. Bioengineered 2012; 3:311-2; PMID:23099453; http://dx.doi.org/10.4161/bioe.22367
- Karr JR, Sanghvi JC, Macklin DN, Gutschow MV, Jacobs JM, Bolival B, Jr., Assad-Garcia N, Glass JI, Covert MW. A whole-cell computational model predicts phenotype from genotype. Cell 2012; 150:389-401; PMID:22817898; http://dx.doi.org/10.1016/j.cell.2012.05.044
- Sleator RD. Designer probiotics: Development and applications in gastrointestinal health. World J Gastrointest Pathophysiol 2015; 6:73-8; PMID:26301121
- Sleator RD, Hill C. Designer probiotics: a potential therapeutic for Clostridium difficile? J Med Microbiol 2008; 57:793-4; PMID:18480340; http://dx.doi.org/10.1099/jmm.0.47697-0
- Sleator RD. Under the microscope: From pathogens to probiotics and back. Bioengineered 2015; 6:275-82; PMID:26339986; http://dx.doi.org/10.1080/21655979.2015.1089368
- Sleator RD. The genetic code. Rewritten, revised, repurposed. Artificial DNA, PNA & XNA 2014; 5:e29408; http://dx.doi.org/10.4161/adna.29408
- Sleator RD. Genetics just got SEXY: Sequences encoding XY. Bioengineered 2014; 5:214-5; PMID:24847983; http://dx.doi.org/10.4161/bioe.29306
- Sleator RD, O'Driscoll A. Digitizing humanity. Artificial DNA, PNA & XNA 2013; 4:37-8; http://dx.doi.org/10.4161/adna.25489