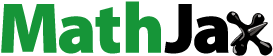
ABSTACT
This study aimed to investigate the dynamic changes that occur in the chemical composition of an Enterococcus faecalis (E. faecalis) biofilm under conditions of starvation and in an alkaline environment and to explore the function of chemical composition changes in the resistance of the E. faecalis biofilm to an extreme environment. This study established an in vitro E. faecalis biofilm model under starvation and in an alkaline environment. During the formation of the biofilm, the pH value and nutritional condition of the culture medium were changed, and the changes in chemical composition were observed using biochemical measures. The results showed that, when the pH value of the culture medium was 11, the percentage of water-insoluble polysaccharides in the biofilm was significantly lower than under other conditions. In addition, the percentage of water-soluble polysaccharides in culture medium with pH values of 9 and 11 gradually decreased. The level of the water-soluble polysaccharides in each milligram of dry weight of biofilm at pH 11 increased compared to that under other conditions. The results from this study indicate that the chemical composition of E. faecalis biofilm changed in extreme environments. These changes served as a defensive mechanism for E. faecalis against environmental pressures.
Introduction
Biofilm is well known to be an important concept in persistent root canal infections. Conceptually, the most dangerous pathogen is not a single individual but rather an entity of microbial coaggregates. After being stimulated by changes in external environmental pressures, this entity can produce a variety of response mechanisms in order to ensure survival and normal physiological activity. Periapical periodontitis is a type of common and frequent disease that endangers oral health in humans. Root canal therapy is the most effective treatment method because its purpose is to remove bacteria, bacterial decomposition products, and the metabolic products in the root canal. However, clinical studies have indicated that even a perfect root canal therapy still has a 20% to 30% failure rate. Post-treatment endodontic disease (PED) refers to nonhealing periapical lesions or new apical lesions in the diseased tooth after root canal therapy. According to Williams J et al.Citation1 Enterococcus faecalis is an important microorganism in persistent infection and reinfection of the root canal. The percentage of bacteria discovered in PED identified as E. faecalis reaches 24% to 77%, noted by Stuart C et al.Citation2 Therefore, this bacterium is considered dominant in root canals in PED cases.
E. faecalis in the root canal after root canal therapy faces an enormous environmental pressure - extremely poor nutrition and high alkalinity - which is severely challenging to the survival of E. faecalis. To date, studies in E. faecalis still have not involved reports of dynamic analyses of the chemical composition of biofilm under starvation and in an alkaline environment. Therefore, this study performed dynamic studies on the chemical composition of E. faecalis under starvation and in an alkaline environment to investigate the differences in the chemical composition of E. faecalis biofilm in these 2 types of extreme environment.
Materials and methods
Experimental bacterial strain: E. faecalis ATCC33186
The standard bacterial strain was provided by the Laboratory of Oral Microbiology in the Ninth People's Hospital of Shanghai JiaoTong University School of Medicine, China, and was stored at −80°C.
Preparation of bacterial biofilm in normal, starvation, and alkaline environments
The E. faecalis standard strain ATCC33186 was inoculated into tryptic soy broth (TSB) and incubated at 37°C overnight. The concentration of the cultured bacterial solution was adjusted to approximately 2 × 109 cfu/ml, and 80 μl was inoculated onto glass Petri dishes containing 20 ml of fresh TSB medium. After culture for 24 h, 48 h, and 72 h for formation of the biofilm, the supernatant in the Petri dishes was discarded, and the culture was washed 3 times with sterile PBS to remove bacteria floating on the surface. Biofilm bacterial cells were scraped off and collected into a 1.5-ml Eppendorf (Ep) tube. Preparation of the bacterial biofilm under starvation and in an alkaline environment was performed in E. faecalis liquid medium with or with 0.5% glucose and at pH 10, 10.5, or 11. The other steps were the same as for the biofilm under normal conditions.
Biofilm processing
Hydroxyapatite sheets were removed at experimentally designed observation time points and placed in test tubes containing 2 ml of normal saline. After being vortexed for 30 sec, the biofilm was stripped from the hydroxyapatite sheets, and the sheets were discarded. Another 3 ml of normal saline was added, followed by centrifugation at 4°C and 5500 rpm for 10 min. The supernatant was then collected. The precipitate was washed twice with 5 ml of distilled water, and the supernatant was again collected.
Determination of biochemical indicators
Dry weight
The measurement of the dry weight of the biofilm was performed using 0.5 ml from the 2.55 ml of the precipitate resuspension solution. The precipitate resuspension solution was placed on one piece of aluminum foil of pre-determined weight, and this piece of foil was then placed in a 100°C oven for 3 h. The weigh was then measured.
Total protein
The Coomassie Brilliant Blue method described by Bradford was performed. The biofilm obtained after sonication was diluted using PBS buffer to the appropriate fold. Three 1.5-ml centrifuge tubes were obtained, and 100 μl of PBS buffer was added to one tube as the blank control. The other 2 tubes received 100 μl of the sample dilution solution of the same concentration. All tubes received 1 ml of Bradford reagent (Bradford Protein Concentration Determination Reagent Kit, Sangon Biotech, Shanghai, China) and were mixed rapidly and thoroughly. The solution was allowed to sit at room temperature for 5 min; within the 5-min period, samples were mixed 1–2 times by inversion. After 5 min, the A595nm value of each tube was measured in a spectrophotometer. The mean A595nm value of 2 identical sample dilution solutions was calculated. The protein concentrations of the diluted samples were confirmed on a standard curve. The standard curve was as follows: Y = 0.0016X and R2 = 0.9993. The protein concentrations in the samples were calculated using the following formula:
Water-soluble polysaccharides (WSPs)
Three millimeters of supernatant from the 15-ml supernatant solution was collected and precipitated in cold 95% ethanol at −20°C for 18 h. After the sample was centrifuged at 4°C and 9500 rpm for 20 min, the supernatant was carefully discarded, and the precipitate was washed 3 times with cold 75% ethanol. The concentration of the WSP was measured using the phenol-sulfuric acid method (0.1% glucose was used for the standard curve).
Water-insoluble polysaccharides (alkaline soluble polysaccharides, ASPs)
One millimeter of supernatant from the 2.55 ml of the precipitate resuspension solution was placed in an Ep tube and dried for 3 h in a SpeedVac concentrator. The dried substance was weighed. ASPs (300 ml/mg) were extracted after adding 1 N NaOH 3 times (incubated at room temperature for 2 h). The supernatant was obtained after the tubes had undergone centrifugation at 13,000 rpm for 10 min. Polysaccharides were precipitated in cold 95% ethanol at −20°C for 18 h. After centrifugation at 4°C and 9500 rpm for 20 min, the supernatant was carefully removed and discarded, and the precipitate was washed 3 times with cold 70% ethanol. The concentration of the WSP was measured using the phenol-sulfuric acid method (0.1% glucose was used for the standard curve).
Intracellular polysaccharide (IPS)
One millimeter of supernatant from the 2.55 ml of the precipitate resuspension solution was placed in an Ep tube and dried for 3 h in a SpeedVac concentrator. The dried substance was weighed and dissolved in phosphate buffer. After 0.1% KI and 6.3 M HCl (1 ml/mg) had been added to the sample, the tube was heated in a boiling water bath for 2 h, and the sample was neutralized using 6.3 M NaOH. The concentration of the intracellular polysaccharides was measured using a spectrophotometer (a standard curve was plotted).
Statistical analysis
The statistical analysis in this study was performed using the SPSS 12.0 software (SPSS Inc., Chicago, IL, USA). The difference between the 2 groups was analyzed using a one-way analysis of variance (ANOVA). When there was a significant difference between the groups, the Tukey method was performed for pairwise examination. Significant difference was indicated when p < 0.05 (SPSS Inc.).
Results
and present the analytic results of the biochemical composition of the E. faecalis biofilm under the different conditions of the culture media. From the indicators of the total amount of biofilm formation, the biofilm formation was lowest in the 0.5% glucose group, which was the E. faecalis biofilm cultured under starvation conditions. The total amount of the E. faecalis biofilm in the alkaline environment in the other 3 groups gradually decreased with the increase in the pH value. At pH 11, the alkaline condition was the strongest, and the total amount of biofilm formation reached the lowest level. The levels of total proteins and ASP were also the lowest in the starvation condition; these levels in the 3 groups of different alkalinities showed a gradual decrease with the increase in the pH value. At pH 11, when the alkaline condition was the strongest, the total proteins and ASP reached the lowest levels. The lowest values of WSP and IPS also occurred in the E. faecalis biofilm under the conditions of starvation; however, under alkaline conditions, these levels gradually decreased between pH 9 and pH 10 and increased at pH 11.
Table 1. Biochemical analysis of S. mutans biofilm formed in different sucrose concentrations.
presents the results of the analysis of the dry weight, WSP, ASP, and IPS. The results showed that, in every milligram of dry weight of biofilm, the E. faecalis biofilm in the starvation condition had the lowest WSP. In the biofilm of the 3 groups under alkaline conditions, the level of the WSP gradually decreased in the pH 9 and pH 10 groups; however, the level of WSP in each milligram of dry weight of biofilm increased. The ASP level in the pH 11 group was the lowest, followed by the pH 10 group. The ASP levels in the pH 9 group and the starvation group were both 0.23. The level of IPS was the lowest in the starvation group, followed by the pH 9 and pH 10 groups. The level in the pH 11 group was slightly higher than the levels for the pH 9 and pH 10 groups.
shows the results of the analysis for the WSP, ASP, and IPS levels in each unit weight of protein. The results show that in each milligram of biofilm protein, the levels of WSP, ASP, and IPS in the E. faecalis biofilm under the condition of starvation were still the lowest. In the alkaline condition, the WSP level decreased with the increase in pH, whereas the ASP and IPS levels decreased with the increase in pH. Biofilm protein can reflect the number of bacteria in the biofilm, and the levels of WSP, ASP, and IPS in the unit weight of protein can also reflect the relation of these polysaccharides with the bacteria.
shows the results of the analysis of the biochemical composition of E. faecalis biofilm under the different conditions, and these results reflect the changes in the percentage of chemical composition under these different conditions. As shown in the figure, protein content remained relatively stable in the starvation environment, the pH 9 group, and the pH 10 group, whereas it significantly decreased in the pH 11 group, indicating that the number of bacteria had decreased. The percentage of IPS and WSP in the starvation environment, the pH 9 group, the pH 10 group, and the pH 11 group gradually increased. The percentages of ASP among the starvation environment, the pH 9 group, and the pH 10 group showed small differences; however, this percentage was significantly decreased in the pH 11 group.
Discussion
E. faecalis is normal flora in the human intestinal tract. It was first discovered and reported in 1899. Beginning in the 1950s, E. faecalis has been reported to occur in the oral cavity. However, because the percentage of E. faecalis was small (less than 1% of the oral microflora), this occurrence did not receive much attention. However, with ongoing in-depth study, researchers have gained new understanding of the importance of E. faecalis. In particular, the high detection rate of E. faecalis in PED made it the most well-known and important microorganism in persistent infection and reinfection in the root canal.
LoveCitation3 suggested that E. faecalis could enter dentinal tubules and adhere to dentin collagen in the deep dentinal tubules that could not be reached by chemical and mechanical treatment, disinfectants, and sealants; therefore, E. faecalis could not be completely removed. Gunnel et al.Citation4 suggested that E. faecalis used adhesion molecules to form aggregations; the bacterium secreted some proteins and polysaccharides, including Enterococcus surface protein (Esp), collagen-binding protein from E. faecalis (Ace), and serine proteinase (SP) and exerted the pathogenic functions through these molecules. The study of Haas et al.Citation5 confirmed that cell density signals and the virulence factor of related genes of E. faecalis regulated each other and played a role in the pathogenesis of E. faecalis.
Current studies on the mechanisms underlying the defense against environmental pressure by E. faecalis are mostly focused on pressure-associated proteins. Flahaut et al.Citation6 studied protein synthesis by E. faecalis under alkaline environmental pressure. They found that preculture of E. faecalis in an environment with a pH of 10.5 allowed the bacterium to develop the ability to tolerate a pH of 11.9. They also demonstrated the expression of 2 pressure-related proteins, DnaK and GroEL. Evans et al.Citation7 blocked the protein synthesis of E. faecalis in an alkaline environment and showed that the ability of the bacteria to defend against an alkaline environment was not affected. However, after the activity of the proton pump was blocked, the ability to defend against calcium hydroxide decreased significantly.
Furthermore, the effects of changes in the integrity and composition of the bacterial cell membrane on the proton permeability and the proton-translocating H+-translocating ATPase (H+-ATPase) activity of Streptococcus mutans have been reported. According to Fozo et al.Citation8 in order to respond to environmental acidification, the percentage of unsaturated fatty acid in the cell membrane of S. mutans increased. This increase in fatty acid was speculated to reduce the proton permeability of the bacterial cell membrane. When the fabM gene that synthesizes the unsaturated fatty acid was inactivated, the sensitivity of the bacteria to low pH and intracellular pH values could not be reduced. Compared to wild-type strains, mice infected with S. mutans with an inactivated fabM gene had a reduced incidence of dental caries.
Hasona et al. studied the effect of synthesis of cell membrane proteins of bacteria on the tolerance of pressure. Mutants lacking the signal recognition particle (SRP) or the membrane-localization molecule yidC through induction were grown in environments with different types of pressure. The results showed that compared to the wild-type strain, mutant strains had reduced tolerance to many environmental pressures. Researchers have suggested that this reduced tolerance is associated with the influence of the best insertion of membrane proteins by yidC and SRP defects. Notably, mutations in the SRP-related genes also resulted in a reduction in the ability of the bacterium to form a biofilm, which provided evidence for the close association between the tolerance of bacteria to pressure and the ability to form a biofilm, for a review see refs.Citation9,10
The surface antigen BrpA has also been reported to play a role in the formation of bacterial biofilm, cell autolysis, cell division, and pressure tolerance, noted by Wen Z et al..Citation11 The synthesis of protein-related bacterial cell wall synthesis, pressure tolerance, and adhesion was blocked in mutants with an inactivated brpA gene. Although the function of the brpA gene is still not clear, the increase in autolysis in mutant strains with brpA gene inactivation suggests that this gene plays a role in the regulation of bacterial cell membrane integrity. These results also explain why mutants with brpA gene inactivation were more sensitive to acids and oxidative stress.
After a series of root canal therapy steps such as root canal disinfection and root canal preparation, conditions of starvation and alkalinity provide a harsh living environment for E. faecalis. The results in this study indicate that starvation was one of the most important factors affecting the formation of the E. faecalis biofilm; compared to the strong alkaline environment, starvation could better reduce the ability of E. faecalis to form a biofilm. With the increased alkalinity of the living environment, E. faecalis also exhibited less ability to form a biofilm. Similarly, the chemical composition and the percentage of each component of the E. faecalis biofilm that was formed in the culture medium showed changes in all groups. ASP is considered a factor highly associated with biofilm formation and pathogenicity. In the pH 11 group, the percentage of ASP was significantly lower than that in the other groups; this result also explained the significant reduction in the pathogenicity from other aspects. In addition, the levels of WSP in the pH 9 and pH 10 groups gradually decreased, whereas the level of WSP in each milligram of biofilm increased in the group in the pH 11 environment. These results suggested that WSP might play a very important role for E. faecalis in its defense against strong alkaline environments.
In previous studies and our experiments, the same findings are reported: the responses of the bacteria to environmental pressures were closely associated with the formation of the biofilm. We believe that with the rapid development of novel study measures and molecular biology technology, more in-depth studies will be conducted on the characteristics of the root canal infection and the mechanism underlying the defense of pathogenic bacteria such as E. faecalis against environmental pressure. These studies are expected to produce new methods for more effective control of E. faecalis and to provide a theoretical basis for the control of PED, thus increasing the successful rate of root canal therapy and reducing the incidence of PED.
Conclusion
This study involved 24 h of observation on the formation of E. faecalis biofilm in culture medium in different environments and obtained, for the first time, the distribution pattern and 3-dimensional structure of the bacterial component of E. faecalis biofilm in an extremely alkaline environment. These results indicate that starvation was one of the most important factors affecting the formation of E. faecalis biofilm. When the alkalinity was higher, E. faecalis showed a lessened ability to form biofilm. WSP played a very important role in defense against an alkaline environment by E. faecalis. Unfortunately, clear images of the 3-dimensional structure of E. faecalis biofilm in the starvation environment were not obtained. The ability of E. faecalis to form a biofilm in this environment was poor. In future studies, we will further explore and optimize conditions to determine the reasons for these results.
Disclosure of potential conflicts of interest
No potential conflicts of interest were disclosed.
Funding
This work was supported by the National Natural Science Foundation of China (81000427 and 81370024).
References
- Williams J, Trope M, Caplan D, Shugars DC. Detection and quantitation of E. faecalis by real-time PCR (qPCR), reverse transcription-PCR (RT-PCR), and cultivation during endodontic treatment. J Endod 2006; 32:715-21; PMID:16861068; http://dx.doi.org/10.1016/j.joen.2006.02.031
- Stuart C, Schwartz S, Beeson T. Enterococcus faecalis: its role in root canal treatment failure and current concepts in retreatment. J Endod 2006; 32(2):93-8; PMID:16427453; http://dx.doi.org/10.1016/j.joen.2005.10.049
- Love R. Enterococcus faecalis–a mechanism for its role in endodontic failure. Int Endod J 2001; 34(5):399-405; PMID:11482724; http://dx.doi.org/10.1046/j.1365-2591.2001.00437.x
- Gunnel S, Gunnar B. Endodontic infections: concepts, paradigms and perspectives. Endod Topics 2004; 91:27-36.
- Haas W, Shepard B, Gilmore M. Two-component regulator of Enterococcus faecalis cytolysin responds to quorum-sensing autoinduction. Nature 2002; 415; (6867):84-7; PMID:11780122; http://dx.doi.org/10.1038/415084a
- Flahaut S, Hartke A, Giard J. Alkaline stress response in Enterococcus faecalis: Adaptation, cross-protection, and changes in protein synthesis. Appl Environ Microbiol 1997; 2:812-4.
- Evans M, Davies J, Sundqvist G, Figdor D. Mechanisms involved in the resistance of Enterococcus faecalis to calcium hydroxide. Int Endod J 2002; 35:221-8; PMID:11985673; http://dx.doi.org/10.1046/j.1365-2591.2002.00504.x
- Fozo E, Quivey R. Shifts in the membrane fatty acid profile of Streptococcus mutans enhance survival in acidic environments. Appl Environ Microbiol 2004; 70:929-36; PMID:14766573; http://dx.doi.org/10.1128/AEM.70.2.929-936.2004
- Hasona A, Crowley P, Levesque C. Streptococcal viability and diminished stress tolerance in mutants lacking the signal recognition particle pathway or YidC2. Proc Natl Acad Sci USA 2005; 102:17466-71; PMID:16293689; http://dx.doi.org/10.1073/pnas.0508778102
- Hasona A, Zuobi-Hasona K, Crowley P. Membrane composition changes and physiological adaptation by Streptococcus mutans signal recognition particle pathway mutants. J Bacteriol 2007; 189:1219-30.; PMID:17085548; http://dx.doi.org/10.1128/JB.01146-06
- Wen Z, Baker H, Burne R. Influence of BrpA on critical virulence attributes of Streptococcus mutans. J Bacteriol 2006; 188:2983-92.; PMID:16585759; http://dx.doi.org/10.1128/JB.188.8.2983-2992.2006