ABSTRACT
Siberian permafrost is a unique environment inhabited with diverse groups of microorganisms. Among them, there are numerous producers of biotechnologically relevant enzymes including lipases and esterases. Recently, we have constructed a metagenomic library from a permafrost sample and identified in it several genes coding for potential lipolytic enzymes. In the current work, properties of the recombinant esterases obtained from this library are compared with the previously characterized lipase from Psychrobacter cryohalolentis and other representatives of the hormone-sensitive lipase family.
The hormone-sensitive lipase (HSL) family (or Family IV) of lipolytic enzymes includes numerous members which, similar to other lipases and esterases, share characteristic α/β hydrolase fold and a catalytic triad including Ser-His-Asp/Glu residues.Citation1,2 The enzyme core is surrounded with loops and other additional structural elements and often covered with a lid which determines the availability of the active site.Citation3 Representatives of the HSL family are found in microorganisms from diverse habitats. It was shown that structural and functional characteristics of these enzymes are directly related with the thermal adaptation of their hosts.Citation4
Siberian permafrost is a unique environment with extreme conditions. It is inhabited by diverse groups of microorganisms.Citation5,6 Previously, we have expressed and characterized a cold-adapted lipase belonging to the HSL family from a permafrost bacterium Psychrobacter cryohalolentis K5T.Citation7 Recently, a number of lipases and esterases from this family were discovered using the metagenomic approach. Among them, the members of a new subfamily containing GTSAG sequence motif were identified.Citation8
In our current work, we have constructed a metagenomic DNA library from a 30,000-year-old permafrost sample.Citation9 Screening of the obtained fosmid library on tributyrin plated resulted in isolation of halo-producing clones and identification of ORFs coding for potential lipolytic enzymes.Citation10 Among them, several ORFs demonstrated homology to the members of the HSL family. Amino acid sequence of the first putative protein, PMGL1, was apparently shortened in comparison with homologs, while the adjacent PMGL2 possessed the length similar to the homologous lipases/esterases. We have cloned the gene coding for PMGL2 from the secondary library constructed in pUC19 plasmid and characterized the corresponding protein after expression in Escherichia coli cells.Citation10 This study demonstrated that PMGL2 is an esterase which is active in a broad temperature range, with a preference for mesophilic conditions.
Sequencing of another fosmid DNA from a positive clone allowed us to identify the gene consisting of 912 bp and encoding a putative lipase of 304 amino acid residues with predicted molecular weight of 32337.6 Da. According to the results of the BLAST search, its amino acid sequence displays maximum likelihood to the uncharacterized proteins from Phenylobacterium sp., Pseudomonas sp., Bradyrhizobium sp. and several uncultured microorganisms. Search in the ESTHER database demonstrated that a new protein (PMGL3) also belongs to the HSL family of lipolytic enzymes. Multiple alignment of Lip1Pc, PMGL2 and PMGL3 revealed only low level of sequence homology between them (22–28%) while the positions of 3 putative catalytic residues are well conserved (). In PMGL3, 2 adjacent carboxylic residues (Asp242 and Glu243) could be aligned with putative catalytic aspartates of Lip1Pc and PMGL2. To clarify their role, additional mutagenesis studies and/or structure determination of the protein are required.
Figure 1. Alignment of amino acid sequences of Lip1Pc from Psychrobacter cryohalolentis K5T, PMGL2 and PMGL3 from the permafrost metagenomic library. Conserved sequence motifs are underlined. Amino acid residues presumably comprising the catalytic triad are indicated by •.
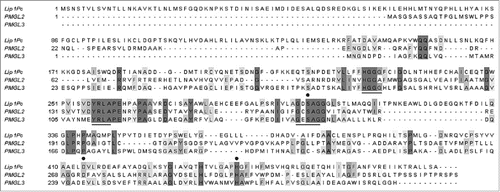
It should be mentioned that the sequence motif encompassing the putative catalytic serine residue differs in all 3 proteins. In Lip1Pc, it contains a typical aspartate residue (GDSAG), while in PMGL3 this aspartate is substituted for a glutamate (GESAG). In PMGL2, which belongs to the GTSAG subfamily, this motif nevertheless includes a cysteine residue instead of a threonine (GCSAG). The functional consequences of these differences currently are not clear and deserve special investigation.
Previously we have shown that the content of the selected amino acid residues in Lip1Pc reflects its cold-adapted phenotype.Citation7 Its primary sequence is characterized by overrepresentation of the residues with the polar side chains (S, T, N, Q) and decrease of proline and arginine content (). On the contrary, PMGL2 and PMGL3 contain less lysines, more prolines and arginines than Lip1Pc. At the same time, the content of glycine residues is higher in these proteins (9.2–9.3% in comparison with 5.6% in Lip1Pc). All three proteins have a high percentage of negatively charged residues that could be a result of their adaptation to the low water activity of permafrost soil.Citation11
Table 1. Selected amino acid residues content (%) in the protein sequences of Lip1Pc, PMGL2 and PMGL3.
To compare properties of the new protein with previously characterized enzymes, we have expressed PMGL3 in E. coli cells with C-terminal His-tag. Due to high expression yield and solubility, the protein was obtained with sufficient purity after a single round of nickel-affinity chromatography. The purified PMGL3 demonstrated the highest catalytic activity toward p-nitrophenyl butyrate (C4) and p-nitrophenyl octanoate (C8) at pH 8.5. Study of the temperature dependence of the esterase activity of PMGL3 using C4 substrate revealed that it possesses maximum activity at 30°C similar to the cold-adapted esterases from the Arctic sediment metagenome.Citation12 Activity at 10°C constituted 30% of the maximum and the same activity level was achieved at 40°C (). Further temperature increase resulted in the rapid loss of the activity. Thus, the temperature pattern of PMGL3 activity has a sharp maximum similar to other esterases previously isolated from metagenomic libraries (EstKT7, E25 and E40).Citation8,13,14 With C8 substrate, the temperature optimum of PMGL3 was slightly higher (35°C, ). The maximum activity of PMGL2 with C4 substrate was achieved at 45°C and Lip1Pc possessed a temperature optimum at 25ºC. In contrast to PMGL2 and PMGL3, the latter enzyme demonstrated high level of activity at the temperature range 5–40°C (60–95% of the maximum) (). Similar activity pattern was also observed for the cold-active carboxyl esterase from Oleispira antarctica,Citation15 lipases EML1Citation16 and EstKT4.Citation8 The esterase activity of the 3 enzymes was confirmed by the appearance of clear halos around the colonies of the expressing strains on tributyrin agar plates ().
Figure 2. Temperature dependence of Lip1Pc (-▴-), PMGL2 (-▪-) and PMGL3 (-•-) esterase activity. Enzyme activities were determined with p-nitrophenyl butyrate (A) and p-nitrophenyl octanoate (B) as a substrate after 15 min incubation at various temperatures. Activity values obtained at 25ºC (for Lip1Pc), 30ºC (for PMGL2) and 45°C (for PMGL3) were taken as 100%. C - E. coli clones expressing Lip1Pc (1), PMGL2 (2) and PMGL3 (3) on tribytyrin agar plate. Four, clone transformed with empty vector (negative control). After spotting the overnight cultures of the respective strains the plate was incubated at 30ºC for 24 h.
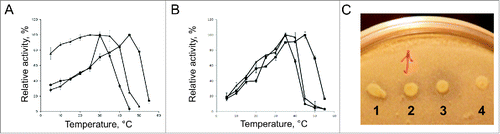
Analysis of the remaining lipolytic activity after initial incubation at different temperatures revealed that after 1 h at 30°C all 3 enzymes demonstrated relatively good stability () while treatment at 40°C resulted in the loss of 50% of the activity by PMGL3 and 85% by Lip1Pc. Incubation at 50°C led to almost complete inactivation of Lip1Pc and PMGL3, while PMGL2 still preserved 65% of its activity (). Consequently, Lip1Pc and PMGL3 can be attributed to cold-adapted enzymes and PMGL2 demonstrates mesophilic behavior. The results of the study demonstrate the presence of the lipolytic enzymes with different activity patterns in permafrost environment and its significance as a unique genetic reservoir with considerable biotechnological potential.
Disclosure of potential conflicts of interest
No potential conflicts of interest were disclosed.
Funding
The work was supported by Russian Scientific Foundation grant No. 14-14-01115.
References
- Arpigny J, Jaeger K. Bacterial lipolytic enzymes: classification and properties. Biochem J 1999; 343:177-183; PMID:10493927; https://doi.org/10.1042/bj3430177
- Casas-Godoy L, Duquesne S, Bordes F, Sandoval G, Marty A. Lipases: An Overview. In: Sandoval G, ed. Lipases and Phospholipases: Humana Press, 2012:3-30.
- Secundo F, Carrea G, Tarabiono C, Gatti-Lafranconi P, Brocca S, Lotti M, Jaeger K-E, Puls M, Eggert T. The lid is a structural and functional determinant of lipase activity and selectivity. J Mol Catal B-Enzym 2006; 39:166-70; https://doi.org/10.1016/j.molcatb.2006.01.018
- Mandrich L, Pezzullo M, Del Vecchio P, Barone G, Rossi M, Manco G. Analysis of thermal adaptation in the HSL enzyme family. J Mol Biol 2004; 335:357-69; PMID:14659763; https://doi.org/10.1016/j.jmb.2003.10.038
- Rivkina E, Laurinavichius K, McGrath J, Tiedje J, Shcherbakova V, Gilichinsky D. Microbial life in permafrost. Adv Space Res 2004; 33:1215-21; PMID:15806703; https://doi.org/10.1016/j.asr.2003.06.024
- Vishnivetskaya TA, Petrova MA, Urbance J, Ponder M, Moyer CL, Gilichinsky DA, Tiedje JM. Bacterial community in ancient Siberian permafrost as characterized by culture and culture-independent methods. Astrobiology 2006; 6:400-14; PMID:16805696; https://doi.org/10.1089/ast.2006.6.400
- Novototskaya-Vlasova K, Petrovskaya L, Rivkina E, Dolgikh D, Kirpichnikov M. Characterization of a cold-active lipase from Psychrobacter cryohalolentis K5T and its deletion mutants. Biochemistry (Moscow) 2013; 78:385-94; https://doi.org/10.1134/S000629791304007X
- Jeon JH, Lee HS, Kim JT, Kim SJ, Choi SH, Kang SG, Lee JH. Identification of a new subfamily of salt-tolerant esterases from a metagenomic library of tidal flat sediment. Appl Microbiol Biotechnol 2012; 93:623-31; PMID:21720822; https://doi.org/10.1007/s00253-011-3433-x
- Rivkina E, Petrovskaya L, Vishnivetskaya T, Krivushin K, Shmakova L, Tutukina M, Meyers A, Kondrashov F. Metagenomic analyses of the late Pleistocene permafrost–additional tools for reconstruction of environmental conditions. Biogeosciences 2016; 13:2207-19; https://doi.org/10.5194/bg-13-2207-2016
- Petrovskaya LE, Novototskaya-Vlasova KA, Spirina EV, Durdenko EV, Lomakina GY, Zavialova MG, Nikolaev EN, Rivkina EM. Expression and characterization of a new esterase with GCSAG motif from a permafrost metagenomic library. FEMS Microbiol Ecol 2016; 92:fiw046; PMID:26929439; https://doi.org/10.1093/femsec/fiw046
- Karan R, Capes MD, DasSarma S. Function and biotechnology of extremophilic enzymes in low water activity. Aquat Biosys 2012; 8:1; https://doi.org/10.1186/2046-9063-8-4
- Jeon JH, Kim J-T, Kang SG, Lee J-H, Kim S-J. Characterization and its potential application of two esterases derived from the arctic sediment metagenome. Marine Biotechnol 2009; 11:307-16; https://doi.org/10.1007/s10126-008-9145-2
- Li PY, Ji P, Li CY, Zhang Y, Wang GL, Zhang XY, Xie BB, Qin QL, Chen XL, Zhou BC, et al. Structural basis for dimerization and catalysis of a novel esterase from the GTSAG motif subfamily of the bacterial hormone-sensitive lipase family. J Biol Chem 2014; 289:19031-41; PMID:24867954; https://doi.org/10.1074/jbc.M114.574913
- Li PY, Chen XL, Ji P, Li CY, Wang P, Zhang Y, Xie BB, Qin QL, Su HN, Zhou BC, et al. Interdomain hydrophobic interactions modulate the thermostability of microbial esterases from the hormone-sensitive lipase family. J Biol Chem 2015; 290:11188-98; PMID:25771540; https://doi.org/10.1074/jbc.M115.646182
- Lemak S, Tchigvintsev A, Petit P, Flick R, Singer AU, Brown G, Evdokimova E, Egorova O, Gonzalez CF, Chernikova TN. Structure and activity of the cold-active and anion-activated carboxyl esterase OLEI01171 from the oil-degrading marine bacterium Oleispira antarctica. Biochem J 2012; 445:193-203; PMID:22519667; https://doi.org/10.1042/BJ20112113
- Jeon JH, Kim JT, Kim YJ, Kim HK, Lee HS, Kang SG, Kim SJ, Lee JH. Cloning and characterization of a new cold-active lipase from a deep-sea sediment metagenome. Appl Microbiol Biotechnol 2009; 81:865-74; PMID:18773201; https://doi.org/10.1007/s00253-008-1656-2