ABSTRACT
Five-Aminolevulinic acid (ALA), the universal precursor of all tetrapyrroles, has various applications in medicine and agriculture industries. Glutamyl-tRNA reductase (GluTR) as the first key enzyme of C5 pathway is feedback regulated by heme, and its N-terminus plays a critical role on its stability control. Here, the GluTR N-terminus was engineered by inserting different numbers of positively charged lysine and arginine residues. The results confirmed that insertion of lysine or arginine residues (especially one arginine residue) behind Thr2 significantly increased the stability of GluTR. By co-expression of the GluTR variant R1 and the glutamate-1-semialdehyde aminotransferase, ALA production was improved 1.76-fold to 1220 mg/L. The GluTR variant R1 constructed here could be used for engineering the C5 pathway to enhance ALA and other products.
Five-aminolevulinic acid (ALA) is a natural non-amino acid with 5 carbons. ALA is an important intermediate for biosynthesis of tetrapyrroles, such as heme, chlorophyll, cytochrome and vitamin B12.Citation1 In fact, ALA has been widely used as photodynamic therapy, plant growth regulator, herbicide and insecticide in medicine and agriculture industry.Citation2-5 There are 2 different pathways for ALA biosynthesis.Citation6 One pathway is the C4 pathway, in which glycine and succinyl-CoA are condensed to ALA catalyzed by ALA synthase (ALAS).Citation7 The other pathway is the C5 pathway, in which glutamate as carbon skeleton is transformed into ALA through 3 enzymatic reactions that are catalyzed by glutamyl-tRNA synthetase (GluRS), glutamyl-tRNA reductase (GluTR) and glutamate-1-semialdehyde aminotransferase (GSA-AT).Citation8
Many ALAS encoding sequences have been cloned, expressed and studied.Citation1 On this basis, the industrial workhorses Escherichia coliCitation9-16 and Corynebacterium glutamicumCitation17-18 have been used for ALA production by expressing ALAS with the C4 pathway. Specifically, after optimization of ALAS expression, cultivation process and ALA transport, the titer was eventually increased to 14.7 g/L.Citation19 Even so, due to the addition of precursor glycine and succinic acid, it is still more attractive to produce ALA directly from glucose with a metabolic engineering strategy.Citation20-21
Previously, it has been demonstrated that the key enzyme GluTR of the C5 pathway is feed-back inhibited by heme.Citation22-23 Further studies confirmed that N-terminal domain of GluTR plays an important effect on the protein stability and insertion of 2 lysine residues with positive charge to the third and forth positions at the N-terminus of GluTR improved protein stabilization.Citation24 Accordingly, overexpression of the GluTR mutant with GSA-AT substantially enhanced ALA production.Citation20 Then, based on the fact that ALA dehydratase is feedback inhibited by the intermediate protoporphyrinogen IX,Citation25 both cell growth and ALA production was further improved with addition of Fe2+ in the minimal medium.Citation26 However, the stability of GluTR was still a rate-limiting step for achieving high titer of ALA.
In nature, GluTR interacts with GSA-AT to form a tight complex to catalyze glutamyl-tRNA to ALA via the unstable intermediate GSA.Citation27 Clearly, N-terminus of GluTR involves in interaction with GSA-AT and previous studies have confirmed that the N-terminal peptide plays a critical role on regulating the stability of GluTR during catalysis process ().Citation28 Consequently, in order to further improve the stability of the enzyme, different numbers of lysine were introduced at the N-terminus of GluTR, respectively (). As shown in , compared with the wild-type GluTR (690 mg/L), all the variants with inserting different numbers of lysine resulted in higher accumulation of ALA. Especially, the variant K2 with introduction of 2 lysine residues produced a higher titer (1120 mg/L) which was in consistent with previous results.Citation24
Figure 1. The model complex of GluTR and GSA-AT. The V-shaped dimer protein is GluTR while the other dimer is GSA-AT.
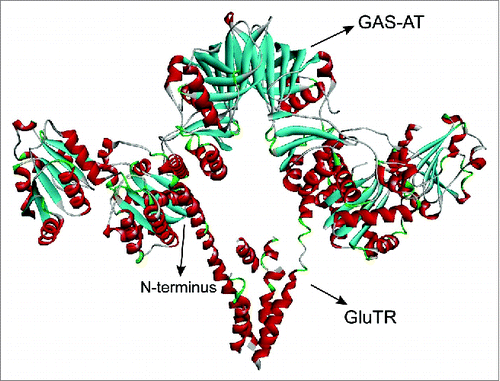
Figure 2. The illustration of GluTR variants with insertion of different numbers of lysine residues (A) and arginine residues (B). WT represented the wild-type GluTR.
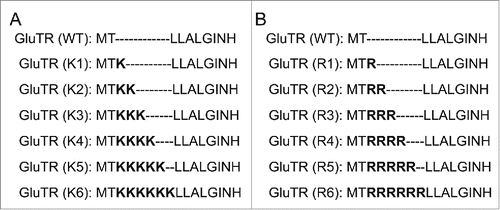
Figure 3. ALA production of the variants with inserting different lysine residues (A) and arginine residues (B).
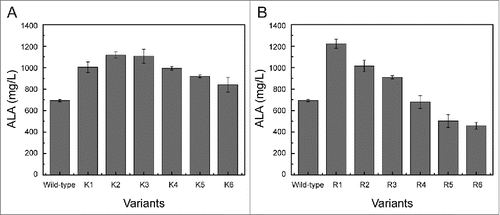
To further investigate the potential reasons, the structure of the variant K2 was simulated and compared with the wild-type GluTR. Clearly, insertion of 2 lysine residues generated more hydrogen bonds (), suggesting that the added positive amino acid residues might weaken the feedback-regulation caused by heme. Thus, the other positively charged amino acid residue arginine was also investigated (). As expected, insertion of arginine with different numbers also resulted in similar results while the variant R1 produced the highest titer (1220 mg/L, ), which was 176.8% of that of the wild-type GluTR. At the same time, the expression level of GluTR with different arginine residues was investigated with SDS-PAGE analysis (). With the increase of arginine residues, the protein expression level was consistently enhanced. In combination with ALA production and structure analysis (), it could be speculated that insertion of more than one arginine residues might weaken the interaction with GSA-AT or decrease the activity although with more stability. As a result, the variant R1 could be used to further optimize the heme biosynthesis pathway for enhancing ALA production.
Disclosure of potential conflicts of interest
No potential conflicts of interest were disclosed.
Funding
This study was funded by the National Natural Science Foundation of China (31200020), the Program for Changjiang Scholars and Innovative Research Team in University (No. IRT_15R26), and the 111 Project.
References
- Kang Z, Zhang J, Zhou J, Qi Q, Du G, Chen J. Recent advances in microbial production of δ-aminolevulinic acid and vitamin B12. Biotechnol Adv 2012; 30:1533-42; PMID:22537876; https://doi.org/10.1016/j.biotechadv.2012.04.003
- Bhowmick R, Girotti AW. Cytoprotective induction of nitric oxide synthase in a cellular model of 5-aminolevulinic acid-based photodynamic therapy. Free Radic Biol Med 2010; 48:1296-301; PMID:20138143; https://doi.org/10.1016/j.freeradbiomed.2010.01.040
- Sakamoto FH, Torezan L, Anderson RR. Photodynamic therapy for acne vulgaris: a critical review from basics to clinical practice: part II. Understanding parameters for acne treatment with photodynamic therapy. J Am Acad Dermatol 2010; 63:195-211; PMID:20633797; https://doi.org/10.1016/j.jaad.2009.09.057
- Sasikala C, Ramana CV, Rao PR. Five-Aminolevulinic acid: a potential herbicide/insecticide from microorganisms. Biotechnol Progress 1994; 10:451-9; https://doi.org/10.1021/bp00029a001
- Watanabe K, Tanaka T, Hotta Y, Kuramochi H, Takeuchi Y. Improving salt tolerance of cotton seedlings with 5-aminolevulinic acid. Plant Growth Regul 2000; 32:97-101; https://doi.org/10.1023/A:1006369404273
- Sasaki K, Watanabe M, Tanaka T, Tanaka T. Biosynthesis, biotechnological production and applications of 5-aminolevulinic acid. Appl Microbiol Biotechnol 2002; 58:23-9; PMID:11831472; https://doi.org/10.1007/s00253-001-0858-7
- Liu S, Zhang G, Li X, Zhang J. Microbial production and applications of 5-aminolevulinic acid. Appl Microbiol Biotechnol 2014; 98:7349-57; PMID:25022665; https://doi.org/10.1007/s00253-014-5925-y
- Beale SI. Biosynthesis of the tetrapyrrole pigment precursor, delta-aminolevulinic acid, from glutamate. Plant Physiol 1990; 93:1273-9; PMID:1062668; https://doi.org/10.1104/pp.93.4.1273
- van der Werf MJ, Zeikus JG. Five-Aminolevulinate production by Escherichia coli containing the Rhodobacter sphaeroides hemA gene. Appl Environ Microbiol 1996; 62:3560-6; PMID:8837411
- Choi C, Hong BS, Sung HC, Lee HS, Kim JH. Optimization of extracellular 5-aminolevulinic acid production from Escherichia coli transformed with ALA synthase gene of Bradyrhizobium japonicum. Biotechnol Lett 1999; 21:551-4; https://doi.org/10.1023/A:1005520007230
- Xie L, Hall D, Eiteman MA, Altman E. Optimization of recombinant aminolevulinate synthase production in Escherichia coli using factorial design. Appl Microbiol Biotechnol 2003; 63:267-73; PMID:14661117; https://doi.org/10.1007/s00253-003-1388-2
- Choi HP, Hong JW, Rhee KH, Sung HC. Cloning, expression, and characterization of 5-aminolevulinic acid synthase from Rhodopseudomonas palustris KUGB306. FEMS Microbiol Lett 2004; 236:175-81; PMID:15251194; https://doi.org/10.1111/j.1574-6968.2004.tb09644.x
- Fu W, Lin J, Cen P. Five-Aminolevulinate production with recombinant Escherichia coli using a rare codon optimizer host strain. Appl Microbiol Biotechnol 2007; 75:777-82; PMID:17333171; https://doi.org/10.1007/s00253-007-0887-y
- Fu W, Lin J, Cen P. Enhancement of 5-aminolevulinate production with recombinant Escherichia coli using batch and fed-batch culture system. Bioresour Technol 2008; 99:4864-70; PMID:17993272; https://doi.org/10.1016/j.biortech.2007.09.039
- Fu WQ, Lin JP, Cen PL. Expression of a hemA gene from Agrobacterium radiobacter in a rare codon optimizing Escherichia coli for improving 5-aminolevulinate production. Appl Biochem Biotechnol 2010; 160:456-66; PMID:18800199; https://doi.org/10.1007/s12010-008-8363-4
- Lin J, Fu W, Cen P. Characterization of 5-aminolevulinate synthase from Agrobacterium radiobacter, screening new inhibitors for 5-aminolevulinate dehydratase from Escherichia coli and their potential use for high 5-aminolevulinate production. Bioresour Technol 2009; 100:2293-7; PMID:19095441; https://doi.org/10.1016/j.biortech.2008.11.008
- Ramzi AB, Hyeon JE, Kim SW, Park C, Han SO. Five-Aminolevulinic acid production in engineered Corynebacterium glutamicum via C5 biosynthesis pathway. Enzyme Microb Technol 2015; 81:1-7; PMID:26453466; https://doi.org/10.1016/j.enzmictec.2015.07.004
- Yu X, Jin H, Liu W, Wang Q, Qi Q. Engineering Corynebacterium glutamicum to produce 5-aminolevulinic acid from glucose. Microb Cell Fact 2015; 14:183; PMID:26577071; https://doi.org/10.1186/s12934-015-0364-8
- Yang P, Liu W, Cheng X, Wang J, Wang Q, Qi Q. A new strategy for production of 5-aminolevulinic acid in recombinant Corynebacterium glutamicum with high yield. Appl Environ Microbiol 2016; 82:2709-17; PMID: 26921424; https://doi.org/10.1128/AEM.00224-16
- Kang Z, Wang Y, Gu P, Wang Q, Qi Q. Engineering Escherichia coli for efficient production of 5-aminolevulinic acid from glucose. Metab Eng 2011; 13:492-8; PMID:21620993; https://doi.org/10.1016/j.ymben.2011.05.003
- Kang Z, Wang Y, Wang Q, Qi Q. Metabolic engineering to improve 5-aminolevulinic acid production. Bioeng Bugs 2011; 2:342-5; PMID:22008939; https://doi.org/10.4161/bbug.2.6.17237
- Wang L, Elliott M, Elliott T. Conditional stability of the HemA protein (glutamyl-tRNA reductase) regulates heme biosynthesis in Salmonella typhimurium. J Bacteriol 1999; 181:1211-9; PMID:9973348
- Jones AM, Elliott T. A purified mutant HemA protein from Salmonella enterica serovar Typhimurium lacks bound heme and is defective for heme-mediated regulation in vivo. FEMS Microbiol Lett 2010; 307:41-7; PMID: 20412302; https://doi.org/10.1111/j.1574-6968.2010.01967.x
- Wang L, Wilson S, Elliott T. A mutant HemA protein with positive charge close to the N terminus is stabilized against heme-regulated proteolysis in Salmonella typhimurium. J Bacteriol 1999; 181:6033-41; PMID:10498716
- Zhang J, Kang Z, Chen J, Du G. Optimization of the heme biosynthesis pathway for the production of 5-aminolevulinic acid in Escherichia coli. Sci Rep 2015; 5:8584; PMID:25716896; https://doi.org/10.1038/srep08584
- Zhang J, Kang Z, Ding W, Chen J, Du G. Integrated optimization of the in vivo heme biosynthesis pathway and the in vitro iron concentration for 5-aminolevulinate production. App Biochem Biotechnol 2015; 178:1252-62; PMID:26637361; https://doi.org/10.1007/s12010-015-1942-2
- Moser J, Schubert WD, Beier V, Bringemeier I, Jahn D, Heinz DW. V-shaped structure of glutamyl-tRNA reductase, the first enzyme of tRNA-dependent tetrapyrrole biosynthesis. Embo J 2001; 20:6583-90; PMID:11726494; https://doi.org/10.1093/emboj/20.23.6583
- Luer C, Schauer S, Mobius K, Schulze J, Schubert WD, Heinz DW, Jahn D, Moser J. Complex formation between glutamyl-tRNA reductase and glutamate-1-semialdehyde 2,1-aminomutase in Escherichia coli during the initial reactions of porphyrin biosynthesis. J Biol Chem 2005; 280:18568-72; PMID:15757895; https://doi.org/10.1074/jbc.M500440200