ABSTRACT
Previous studies indicated that long non-coding RNAs (LncRNAs) were involved in the progression of multiple cancers including ovarian cancer (OV). LncRNA ELFN1-AS1 functioned as an oncogene in many cancers, but its potential roles in OV were largely unclear. In the current study, we were aimed at clarifying the biological roles and molecular mechanisms of ELFN1-AS1 in OV. We found that ELFN1-AS1 was significantly upregulated in OV tissues and cell lines. High expression of ELFN1-AS1 was associated with poor prognosis in OV patients. Knockdown of ELFN1-AS1 inhibited the proliferation, migration and invasion of SKOV3 cell lines and repressed tumor growth in xenografted ovarian models. Mechanistically, ELFN1-AS1 promoted the proliferation, migration and invasion of SKOV3 cells by sponging miR-497-3p. Additionally, CLDN4 was verified to be the target of miR-497-3p. Rescue experiments revealed that miR-497-3p inhibition could partly reverse the inhibitory effect of ELFN1-AS1 silencing on proliferation, migration and invasion of SKOV3 cell lines. Taken together, our findings indicated that ELFN1-AS1 acted as an oncogene in ovarian cancer through regulating the expression of CLDN4 by directly interacting with miR-497-3p. The results suggested that ELFN1-AS1 might act as a promising therapeutic target for OV.
KEYWORDS:
Introduction
Ovarian cancer (OV), one of the most common malignant tumor in women, is associated with serious social and medical burdens [Citation1,Citation2]. The current treatments for OV include radical operation and chemotherapy, but the prognosis of OV is still poor, and many patients undergoing radical OV resection still have a high rate of recurrence and metastasis [Citation3]. Actually, these currently available treatments can hardly inhibit the pathological process of OV development and progression [Citation4]. Therefore, it is essential to further clarify the key molecular mechanisms of OV, which might provide novel therapeutic targets for OV treatment.
Long non-coding RNAs (LncRNAs), a group of non-coding RNAs with length more than 200 nucleotides, play important roles in the processes of tumorigenesis including cell growth, apoptosis, migration, invasion, and metastasis [Citation5–9]. Furthermore, increasing studies indicated that many LncRNAs participated in the development and progression of OV [Citation10–12]. Li et al. found that SNHG14 facilitated the progression of OV through inhibiting the expression of miR-219a-5p [Citation13]. Yan and coworkers revealed that MLK7-AS1/miR-375/YAP1 axis played important roles in the pathological process of OV [Citation14]. Recently, ELFN1-AS1 was found to be involved in the pathogenesis of many cancers [Citation15–17]. For instance, Lei and coworkers found that ELFN1-AS1 was upregulated in colorectal cancer and inhibition of ELFN1-AS1 repressed the proliferation and migration of cancer cells [Citation18]. Furthermore, they found that ELFN1-AS1 exerted pro-proliferation, anti-apoptosis and pro-migration functions on colorectal cancer cells via miR-4644/TRIM44 axis [Citation18]. Similarly, Zhang and colleagues found that knockdown of ELFN1-AS1 inhibited tumor cell migration, invasion and proliferation in esophageal cancer by upregulating GFPT1 via sponging miR-183-3p [Citation19]. Regardless of the important functions of ELFN1-AS1 in the progression of other cancers, the potential roles and possible mechanisms of ELFN1-AS1 in OV were largely unclear.
In the current study, we found that ELFN1-AS1 was significantly upregulated in OV tissues and cell lines. Knockdown of ELFN1-AS1 inhibited the proliferation, migration and invasion of ovarian cancer cells in vitro and repressed OV progression in vivo. Furthermore, we verified that ELFN1-AS1 acted as an oncogene in OV through regulating the expression of CLDN4 by sponging miR-497-3p.
Materials and methods
Patients and tissues samples
Twenty OV tissues and paired adjacent normal tissues were collected from patients who had diagnosed as OV and underwent surgery at Jiangxi Maternal and Child Health Hospital (Jiangxi, China). Samples were immediately frozen in liquid nitrogen and stored at −80°C. All patients did not undergo radio-chemotherapy or other treatments prior to surgery. Besides, all patients had signed consent before this research, which was approved by the Ethics Committee of Jiangxi Maternal and Child Health Hospital.
Cell culture and cell transfection
Human normal fallopian tube epithelial cell line (FTE187) and three OV cell lines including HO8910, HO8910PM, and SKOV3 were obtained from the Chinese Academy of Sciences (Shanghai, China). HO8910 and HO8910PM were grown in RPMI-1640 medium with fetal bovine serum (FBS), while SKOV3 in Dulbecco modified Eagle medium (DEME) supplemented with 10% FBS in a humidified atmosphere of 5% CO2 incubator at 37°C. Human ovarian cancer cells were transfected with siRNA targeting ELFN1-AS1, a scrambled negative control, miR-497-3p inhibitor, and inhibitor NC which were purchased from GenePharma (Shanghai, China). Cell transfection was performed using the Lipofectamine 3000 (Invitrogen, Carlsbad, CA, USA) according to the manufacturer’s instructions and cells were harvested at 48 hours after transfection.
Cell proliferation assay
SKOV3 cells were seeded in 96-well plates (1.5 × 104 cells per well). Cell proliferation was assessed by CCK-8 kit (Dojindo) daily for 4 days after transfection. The optical density value (OD value) at the wavelength of 450 nm was measured using a microplate reader (Thermo Fisher Scientifc, Inc.). The experiment was repeated three times independently.
Cell migration and invasion assay
Transwell chambers was used to assess the migration and invasion ability of SKOV3 cells. The transwell inserts coated with Matrigel (BD Biosciences, Franklin Lakes, NJ, USA) were used in cell invasion assay. Briefly, transfected cells in the logarithmic growth phase were seeded to the upper chambers of transwell plates suspended in 200 μL serum-free DMEM medium. Then, 500 μL DMEM medium containing 10% FBS was added to the lower chamber and incubated at 37°C for 24 h. Then, cells were fixed with 4% paraformaldehyde followed by staining with 1% crystal violet. Cells were visualized and photographed using Olympus fluorescence microscope (Tokyo, Japan).
Real-time quantitative reverse transcription polymerase chain reaction (RT-qPCR)
Total RNAs from tissues and cells were extracted utilizing TRIzol reagent (Invitrogen, Carlsbad, CA, USA) with a RecoverAll™ Total Nucleic Acid Isolation kit (Ambion, Foster City, CA, USA). Reverse transcription reactions were performed using the Prime Script™ RT reagent kit according to the manufacturer’s instructions (Takara, Dalian, China). Subsequently, RT-qPCR analyses were performed with SYBR Premix Ex Taq (Takara Bio, Shiga, Japan). Relative expression levels of mRNA and lncRNA were calculated based on the comparative CT method. GAPDH and U6 were used for reference genes in the results analysis of RT-qPCR. The primers are shown in Table S1. All experiments were performed three times independently.
Western blot assay
Cells were lysed using RIPA lysis buffer (Beyotime, Shanghai, China) and the protein concentration was quantified with BCA Protein Assay Kit. Equal amounts of protein samples were separated at SDS-PAGE and transferred to PVDF membrane. After blocked with fat-free milk at room temperature for 1 h, membranes were incubated with primary antibodies against CLDN4 (Abcam) and GAPDH (CST) at 4°C for overnight. Subsequently, membranes were washed with TBST for three times and incubated the second antibody at room temperature for 1 h. Enhanced chemiluminescence kit (Thermo Fisher Scientific) was utilized to visualize the immunoreactive bands according to the instructions of the manufacturer.
Luciferase reporter assay
The target miRNAs of ELFN1-AS1 were analyzed using bioinformatics online software Lncbase v2 [Citation20]. The results indicated that ELFN1-AS1 existed a putative target for miR-497-3p. Luciferase reporter assays were carried out to test the interaction between the ELFN1-AS1 and miR-497-3p using the Dual Luciferase Reporter Assay System (Promega, Madison, WI, USA). The ELFN1-AS1 mutant (ELFN1-AS1-mut) and ELFN1-AS1 wild (ELFN1-AS1-wt) vectors were synthesized by ELFN1-AS1 cDNA fragment insertion which contained mutated or wild binding sites of miR-497-3p into the pGL3 luciferase reporter vectors. Meanwhile, Targetscan suggested that CLDN4 was a downstream target for miR-497-3p [Citation21]. Furthermore, to assess the correlation between miR-497-3p and ELFN1-AS1 or CLDN4, SKOV3 cells were co-transfected with pGL3 reporter luciferase vector, which contains the 3ʹ-UTR sequence of CLDN4-mut and CLDN4-wt or ELFN1-AS1-wt and ELFN1-AS1-mut and miR-497-3p mimics or negative control. After transfection 48 h using Lipofectamine 2000 (Invitrogen), relative luciferase activities were assessed with the Dual-Luciferase Reporter Assay System (Promega, Madison WI, USA) following the instructions of manufacture.
In vivo tumor growth assay
The 4-week-old female nude mice were purchased from Experimental Animal Center of Nanchang University. The lentiviral viruses ELFN1-AS1 shRNA and shRNA-NC were obtained from KeyGEN BioTECH (Jiangsu China) and were infected into SKOV3 cells to generate the stably expressing cell lines. For subcutaneous tumorigenicity, cells were re-suspended at 1 × 108 cells/ml and a total of 100 μl suspended cells were subcutaneously injected into the right backs of mice (n = 6 for each group). Tumor size was measured every 7 days for 28 days. At the end of the experiment, the mice were killed and tumors were collected for further analysis. All the animal studies were approved under the Institutional Animal Care and Use Committee of Jiangxi Maternal and Child Health Hospital.
Statistical analysis
Data were presented as the mean ± standard deviation (SD). Statistical analysis was performed using SPSS 22.0 software (SPSS, Chicago, IL, USA). Student’s t-test was used to explore the statistically significant differences. P values <0.05 were considered to be statistically significant.
Results
ELFN1-AS1 was upregulated in OV and high expression of ELFN1-AS1 was associated with poor prognosis
We firstly examined the expression level of ELFN1-AS1 in TCGA database, the results indicated that ELFN1-AS1 was upregulated in many cancers including OV (,b)). Furthermore, we also explored the expression level of ELFN1-AS1 in OV tissues and OV cell lines. The results showed that the expression level of ELFN1-AS1 in OV tissues was higher than those in adjacent non-tumor tissues ()). In addition, ELFN1-AS1 was significantly upregulated in the OV cell lines as compared to the normal cell line (FTE187) ()). High expression of ELFN1-AS1 was associated with poor prognosis ()). In particular, the expression level of ELFN1-AS1 in SKOV3 was higher than those in other OV cells, so SKOV3 cell was used for subsequent experiments.
Figure 1. ELFN1-AS1 expression was upregulated in OV samples. (a) The expression profiles of ELFN1-AS1 in human cancers through analyzing TCGA database using GEPIA (http://gepia.cancer-pku.cn/index.html). (b) The expression level of ELFN1-AS1 in OV through analyzing TCGA database using GEPIA. (c) Expression levels of ELFN1-AS1 in 20 paired OV tissues and adjacent non-tumor tissues, *P < 0.05. (d) Expression levels of ELFN1-AS1 in OV cell lines, *P < 0.05. (e) High expression of ELFN1-AS1 was associated with poor prognosis in OV patients.
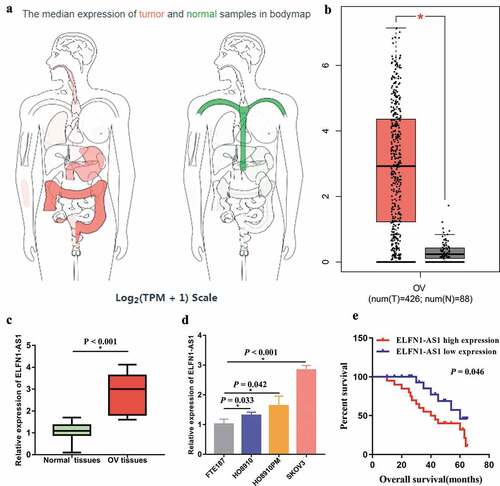
Knockdown of ELFN1-AS1 suppressed the proliferation, migration and invasion of SKOV3 cells
SKOV3 cells were transfected with si-ELFN1-AS1 or si-NC to investigate the effect of ELFN1-AS1 on cell proliferation, migration and invasion. RT-qPCR showed that ELFN1-AS1 expression was significantly reduced after transfecting si-ELFN1-AS1 ()). The results of CCK8 assay showed that the proliferation rate of OC cells was markedly inhibited after transfected with si-ELFN1-AS1 ()). Meanwhile, RT-qPCR showed that silencing of ELFN1-AS1 inhibited that the expression of cyclin D1 ()). Transwell assay showed that knockdown of ELFN1-AS1 inhibited the migration and invasion ability of SKOV3 cells (,e)).
Figure 2. Knockdown of ELFN1-AS1 inhibits SKOV3 cell proliferation, migration and invasion. (a) RT-qPCR analysis was performed to assess the silence efficiency of si-ELFN1-AS1 after transfected with si-ELFN1-AS1 and si-NC, *P < 0.05. (b) The proliferation of SKOV3 cells after transfection was detected by CCK8 assay, *P < 0.05. (c) The expression of cyclin D1 after transfection was detected by RT-qPCR, *P < 0.05. (d,e) The migration and invasion of SKOV3 cells after transfection was detected by transwell invasion and migration assays, *P < 0.05.
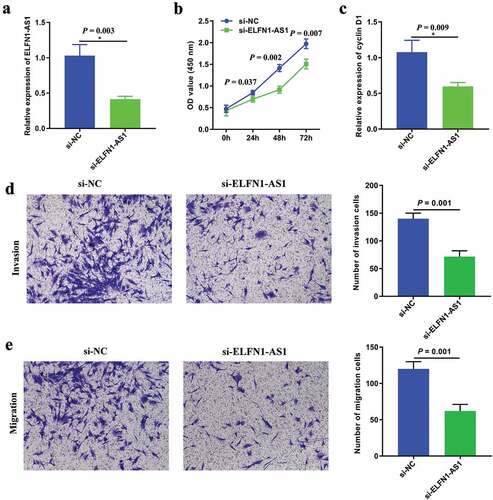
Knockdown of ELFN1-AS1 inhibits OV tumor growth
To further explore whether knockdown of ELFN1-AS1 inhibited the progression of OV in vivo, SKOV3 cells with stable transfected sh-ELFN1-AS1 or sh-NC were injected subcutaneously into nude mice. As shown in ), the expression level of ELFN1-AS1 was significantly lower in tumor tissues of sh-ELFN1-AS1 group when compared with those in sh-NC group. Moreover, the growth rate of tumors grown from sh-ELFN1-AS1-transfected cells was significantly slower than those of tumors grown in sh-NC-transfected group ()). Additionally, the average tumor weight in the sh-ELFN1-AS1 group was significantly lower than those in the sh-NC group in 4 weeks ()).
Figure 3. Knockdown of ELFN1-AS1 inhibits OV tumor growth in vivo. (a) RT-qPCR analysis was performed to assess the silence efficiency of sh-ELFN1-AS1 after transfected with sh-ELFN1-AS1 and sh-NC, *P < 0.05. (b) Tumor volume in the nude mice was calculated every 7 days for 28 days in sh-NC and sh-ELFN1-AS1 groups (n = 6 for each group), *P < 0.05. (c) Tumor weight was removed and measured in the excised tumors from sh-NC and sh-ELFN1-AS1 groups (n = 6 for each group), *P < 0.05.
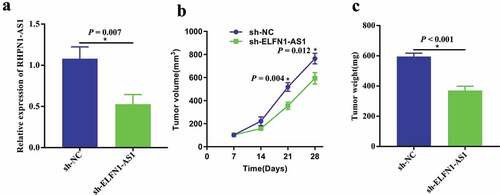
ELFN1-AS1 targeted miR-497-3p in SKOV3 cells
By using LncBase v.2 and RNAhybrid, we predicted that there are several miRNAs that potentially bind to ELFN1-AS1. Of these, we identified that miR-6870-5p, miR-4640-5p, miR-8055, miR-4270, miR-559, miR-6839-3p, miR-183-3p, miR-6089, miR-497-3p, miR-6839-3p, miR-6856-5p, and miR-4644 may be the most potential binding targets for ELFN1-AS1. Accordingly, RT-qPCR revealed that miR-497-3p exhibited the greatest change, so we selected miR-497-3p for further analysis (Figure S1). The above results have been incorporated into the revised manuscript. According to the result of Lncbase v2, there existed the potential binding sites between ELFN1-AS1 and miR-497-3p ()). Furthermore, the dual-luciferase reporter assays were carried out to predict the relationship between ELFN1-AS1 and miR-497-3p. The results showed that the luciferase activity greatly decreased when co-transfected with ELFN1-AS1-wt and miR-497-3p mimics, whereas the luciferase activity did not change significantly in ELFN1-AS1-mut and miR-497-3p mimics group ()). In addition, miR-497-3p expression was significantly upregulated in SKOV3 cells after the downregulation of ELFN1-AS1 ()). Moreover, we detected the expression level of miR-497-3p in OC samples and adjacent noncancerous tissues by qRT-PCR assay. Compared with the adjacent non-tumor samples, the expression level of miR-497-3p was significantly decreased in OV tissues ()). In addition, the Pearson correlation analysis indicated the expression of miR-497-3p was inversely correlated with ELFN1-AS1 expression in OV tissues ()).
Figure 4. ELFN1-AS1 targeted miR-497-3p in SKOV3 cells. (a) Lncbase v2 suggested that there existed predicted complementary sequences between miR-497-3p and ELFN1-AS1. (b) Luciferase reporter assay detected that co-transfection with ELFN1-AS1-wt and miR-497-3p mimic greatly reduced the luciferase activities compared with ELFN1-AS1-mut and miR-497-3p mimic, *P < 0.05. (c) miR-497-3p expression was significantly upregulated in OV cells after inhibition of ELFN1-AS1, *P < 0.05. (d) The expression level of miR-497-3p in OV tissues significantly lower than those in the adjacent non-tumor tissues, *P < 0.05. (e) The expression of miR-497-3p was inversely correlated with ELFN1-AS1 expression in OV tissues.
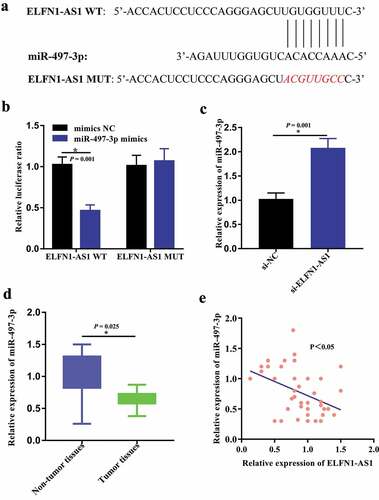
CLDN4 is a direct target of miR-497-3p in SKOV3 cells
According to Targetscan, CLDN4 may be a direct target of miR-497-3p ()), which was further verified by dual-luciferase reporter assays in SKOV3 cells ()). Furthermore, RT-qPCR and western blot indicated that the expression level of CLDN4 was inhibited after miR-497-3p overexpression (–e)). Additionally, further evidence showed that CLDN4 was upregulated in OV through analyzing TCGA database ()) and RT-qPCR results ()) in our clinical specimens.
Figure 5. CLDN4 was a direct target of miR-497-3p in SKOV3 cells. (a) The binding sites between CLDN4 and miR-497-3p predicted by Targetscan. (b) The binding relationship of CLDN4 to miR-497-3p validated by dual-luciferase reporter gene assay, *P < 0.05. The expression level of CLDN4 was detected using RT-qPCR (c) and western blot (d,e), *P < 0.05. (f) The expression level of CLDN4 in OV through analyzing TCGA database using GEPIA, *P < 0.05. (g) The expression level of CLDN4 in OV tissues using RT-qPCR, *P < 0.05.
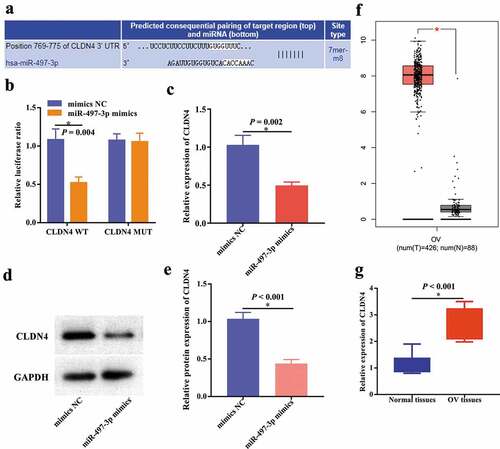
Inhibition of miR-497-3p attenuates the effects of ELFN1-AS1 knockdown in SKOV3 cells
To further explore whether ELFN1-AS1 promoted cell proliferation, invasion and migration via regulating miR-497-3p/CLDN4 axis in OV, we co-transfected si-ELFN1-AS1 and miR-497-3p inhibitor into SKOV3 cells. RT-qPCR and western blot indicated that knockdown of ELFN1-AS1 inhibited the expression of CLDN4, which can be partly reversed by miR-497-3p inhibition (–c)). Furthermore, CCK8 and transwell assays revealed that miR-497-3p inhibition could partly reverse the inhibitory effect of ELFN1-AS1 silencing on proliferation, migration and invasion in ovarian cancer cells (–g)). Taken together, these findings revealed that inhibition of miR-497-3p attenuates the effects of ELFN1-AS1 knockdown in SKOV3 cells.
Figure 6. Inhibition of miR-497-3p antagonized the effects of ELFN1-AS1 knockdown on SKOV3 cells. The inhibition effect of ELFN1-AS1 on CLDN4 expression was rescued by miR-497-3p inhibition detected using RT-qPCR. (a) and western blot (b,c), *P < 0.05. The inhibition effect of ELFN1-AS1 on cell proliferation and cyclin D1 expression was partly reversed by miR-497-3p inhibition detected using CCK8 (d) and RT-qPCR (e), *P < 0.05. The inhibition effect of ELFN1-AS1 on cell migration and invasion was partly reversed by miR-497-3p inhibition detected using transwell assay, *P < 0.05 (f,g).
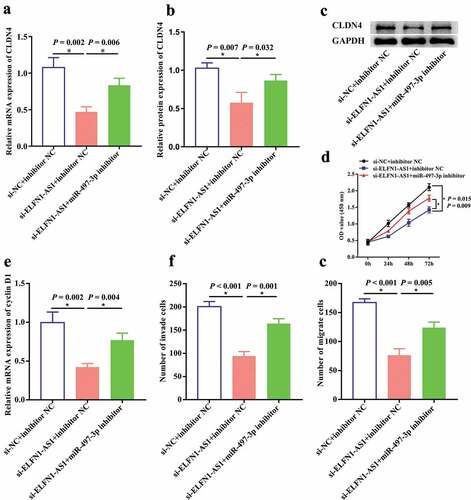
Discussion
LncRNAs have been verified to play important roles in the cancer cell proliferation, migration, invasion and metastasis [Citation10,Citation22,Citation23]. As a kind of lncRNA, ELFN1-AS1 was found to be an oncogene and participated in the progression of various cancers including colorectal cancer and esophageal cancer [Citation18,Citation19]. Regardless of the important functions of ELFN1-AS1 in tumor progression, the potential roles and possible mechanisms of ELFN1-AS1 in OV were largely unclear. The current study demonstrated that ELFN1-AS1 was significantly upregulated in OV and high expression of ELFN1-AS1 was associated with poor prognosis in OV patients. Moreover, knockdown of ELFN1-AS1 inhibited the proliferation, migration and invasion of ovarian cancer cells in vitro and OV tumor growth in vivo.
Previous studies indicated that LncRNAs functioned as ceRNA against miRNAs in OV [Citation24,Citation25]. In the current study, bioinformatics analysis together with subsequent experiments showed that miR-497-3p was a potential target for ELFN1-AS1. Moreover, we found that miR-497-3p was downregulated in OV and miR-497-3p inhibition could partly reverse the inhibitory effect of ELFN1-AS1 silencing on proliferation, migration and invasion in ovarian cancer cells. Actually, previous studies also revealed that miR-497 was associated with the onset and development of cancers and some LncRNAs regulated the progression of cancers through targeting miR-497 [Citation26,Citation27]. Cui et al. revealed that CDKN2B-AS1 acted as an oncogene in laryngeal squamous cell cancer through regulating miR-497/CDK6 pathway [Citation26]. Cui and colleagues found that TTN-AS1 was upregulated in colorectal cancer and TTN-AS1 promoted the proliferation and invasion of colorectal cancer cells via miR-497/PI3K/Akt/mTOR axis [Citation27]. Gao and coworkers investigated that inhibition of UCA1 attenuated thyroid cancer cell proliferation and metastasis by sponging miR-497-3p [Citation28]. In the current study, our findings indicated that miR-497-3p might be a potential tumor suppressor in OV, which may be attributed to the upregulated expression of ELFN1-AS1. Our results found that CLDN4, a member of the claudin family, was a direct target of miR-497-3p in SKOV3 cells. Actually, previous studies suggested that many claudins were significant prognostic biomarkers for several cancers including ovarian cancer [Citation29]. Of these, Claudin-3 and claudin-4 were correlated with poor clinical outcomes in ovarian cancer [Citation30]. Our study indicated that the expression level of CLDN4 was upregulated in OV and lncRNA ELFN1-AS1 promoted OV cell proliferation and metastasis via miR-497-3p/CLDN4 pathway.
Conclusion
To sum up, our findings indicated that ELFN1-AS1 promoted cell proliferation, migration and invasion through miR-497-3p/CLDN4 pathway in OV. Also, we found that ELFN1-AS1 silencing suppressed the progression of OV in vitro and vivo. Therefore, ELFN1-AS1 might serve as a promising diagnostic biomarker and therapeutic target for OV.
Disclosure statement
All the authors had no conflicts of interest or financial ties to disclose.
Supplementary material
Supplemental data for this article can be accessed here.
Additional information
Funding
References
- Jayson GC, Kohn EC, Kitchener HC, et al. Ovarian cancer. Lancet. 2014;384(9951):1376–1388.
- Lheureux S, Gourley C, Vergote I, et al. Epithelial ovarian cancer. Lancet. 2019;393(10177):1240–1253.
- Jelovac D, Armstrong DK. Recent progress in the diagnosis and treatment of ovarian cancer. CA Cancer J Clin. 2011;61(3):183–203.
- Dizon DS. PARP inhibitors for targeted treatment in ovarian cancer. Lancet. 2017;390(10106):1929–1930.
- Zhang M, Song Y, Yu L. LncRNA PTCSC3 suppressed cervical carcinoma cell invasion and proliferation via regulating miR-574-5p. Am J Transl Res. 2019;11(11):7186–7194.
- Yuan L, Ma T, Liu W, et al. LINC00994 promoted invasion and proliferation of gastric cancer cell via regulating miR-765-3p. Am J Transl Res. 2019;11(10):6641–6649.
- Yin X, Zhang J, Li C, et al. LncRNA HOXA11-AS accumulation-induced microRNA-761 downregulation regulates cell growth by targeting TRIM29 in papillary thyroid cancer. Am J Transl Res. 2019;11(11):6826–6837.
- Yang Q, Sun J, Ma Y, et al. LncRNA DLX6-AS1 promotes laryngeal squamous cell carcinoma growth and invasion through regulating miR-376c. Am J Transl Res. 2019;11(11):7009–7017.
- Lei X, Yang S, Yang Y, et al. Long noncoding RNA DLX6-AS1 targets miR-124-3p/CDK4 to accelerate Ewing’s sarcoma. Am J Transl Res. 2019;11(10):6569–6576.
- Wang JY, Lu AQ, Chen LJ. LncRNAs in ovarian cancer. Clin Chim Acta. 2019;490:17–27.
- Liu Y, Sun J, Yu J, et al. LncRNA CACS15 accelerates the malignant progression of ovarian cancer through stimulating EZH2-induced inhibition of APC. Am J Transl Res. 2019;11(10):6561–6568.
- Li Y, Jiao Y, Hao J, et al. Long noncoding RNA TP73-AS1 accelerates the epithelial ovarian cancer via epigenetically repressing p21. Am J Transl Res. 2019;11(4):2447–2454.
- Li L, Zhang R, Li SJ. Long noncoding RNA SNHG14 promotes ovarian cancer cell proliferation and metastasis via sponging miR-219a-5p. Eur Rev Med Pharmacol Sci. 2019;23(10):4136–4142.
- Yan H, Li H, Li P, et al. Long noncoding RNA MLK7-AS1 promotes ovarian cancer cells progression by modulating miR-375/YAP1 axis. J Exp Clin Cancer Res. 2018;37(1):237.
- Dong L, Ding C, Zheng T, et al. Extracellular vesicles from human umbilical cord mesenchymal stem cells treated with siRNA against ELFN1-AS1 suppress colon adenocarcinoma proliferation and migration. Am J Transl Res. 2019;11(11):6989–6999.
- Liu JX, Li W, Li JT, et al. Screening key long non-coding RNAs in early-stage colon adenocarcinoma by RNA-sequencing. Epigenomics. 2018;10(9):1215–1228.
- Polev DE, Karnaukhova IK, Krukovskaya LL, et al. ELFN1-AS1: a novel primate gene with possible microRNA function expressed predominantly in human tumors. Biomed Res Int. 2014;2014:398097.
- Lei R, Feng L, Hong D. ELFN1-AS1 accelerates the proliferation and migration of colorectal cancer via regulation of miR-4644/TRIM44 axis. Cancer Biomark. 2020;27(4):433–443.
- Zhang C, Lian H, Xie L, et al. LncRNA ELFN1-AS1 promotes esophageal cancer progression by up-regulating GFPT1 via sponging miR-183-3p. Biol Chem. 2019. In press.
- Paraskevopoulou MD, Vlachos IS, Karagkouni D, et al. DIANA-LncBase v2: indexing microRNA targets on non-coding transcripts. Nucleic Acids Res. 2016;44(D1):D231–D238.
- Agarwal V, Bell GW, Nam J-W, et al. Predicting effective microRNA target sites in mammalian mRNAs. eLife. 2015;4. DOI:10.7554/eLife.05005.
- Tripathi MK, Doxtater K, Keramatnia F, et al. Role of lncRNAs in ovarian cancer: defining new biomarkers for therapeutic purposes. Drug Discov Today. 2018;23(9):1635–1643.
- Liu E, Liu Z, Zhou Y. Carboplatin-docetaxel-induced activity against ovarian cancer is dependent on up-regulated lncRNA PVT1. Int J Clin Exp Pathol. 2015;8(4):3803–3810.
- Li W, Ma S, Bai X, et al. Long noncoding RNA WDFY3-AS2 suppresses tumor progression by acting as a competing endogenous RNA of microRNA-18a in ovarian cancer. J Cell Physiol. 2020;235(2):1141–1154.
- Liu X, Wen J, Wang H, et al. Long non-coding RNA LINC00460 promotes epithelial ovarian cancer progression by regulating microRNA-338-3p. Biomed Pharmacothe. 2018;108:1022–1028.
- Cui X, Yu T, Shang J, et al. Long non-coding RNA CDKN2B-AS1 facilitates laryngeal squamous cell cancer through regulating miR-497/CDK6 pathway. Onco Targets Ther. 2019;12:8853–8862.
- Cui Z, Han B, Wang X, et al. Long non-coding RNA TTN-AS1 promotes the proliferation and invasion of colorectal cancer cells by activating miR-497-mediated PI3K/Akt/mTOR signaling. Onco Targets Ther. 2019;12:11531–11539.
- Gao H, Yang JY, Tong LX, et al. Long noncoding RNA UCA1 promotes proliferation and metastasis of thyroid cancer cells by sponging miR-497-3p. Eur Rev Med Pharmacol Sci. 2020;24(2):728–734.
- Li J, Chigurupati S, Agarwal R, et al. Possible angiogenic roles for claudin-4 in ovarian cancer. Cancer Biol Ther. 2009;8(19):1806–1814.
- Shang X, Lin X, Alvarez E, et al. Tight junction proteins claudin-3 and claudin-4 control tumor growth and metastases. Neoplasia. 2012;14(10):974–985.