ABSTRACT
Gastric cancer is a considerable health burden worldwide. DNA methylation, a major epigenetic phenomenon, is closely related to the pathogenesis of cancer. Neuronal pentraxin II (NPTX2) has been found to be hypermethylated in several cancers such as glioblastoma and pancreatic cancer. However, the roles of NPTX2 in gastric cancer have not been reported. To explore this issue, NPTX2 expression in gastric cancer cells was assessed by western blot and quantitative real-time polymerase chain reaction (qRT-PCR). The methylation analysis of NPTX2 was performed by qRT-PCR as well as methylation-specific PCR (MS-PCR). The effects of NPTX2 on gastric cancer cell proliferation, apoptosis and cell cycle were detected by colony formation, CCK-8 and flow cytometry assays, respectively. The interaction of NPTX2 with the p53 signaling pathway was evaluated by western blot. Our study found the down-regulated expression of NPTX2 in gastric cancer cells compared with human gastric mucosal cells. In addition, the hypermethylation of NPTX2 was observed in gastric cancer cells, which was correlated with the low expression of NPTX2. Moreover, NPTX2 inhibited gastric cancer cell proliferation, inhibited apoptosis and induced cell cycle arrest. Furthermore, NPTX2 enhanced the protein expression of p53, p21 and PTEN to activate the p53 signaling pathway. Therefore, NPTX2 hypermethylation caused the downregulation of NPTX2 expression, which could promote cell proliferation, inhibit apoptosis and cause cell cycle arrest in gastric cancer cells by suppressing the p53 signaling pathway. Therefore, NPTX2 may be crucial for the progression of gastric cancer.
Introduction
Gastric cancer is the fifth most commonly diagnosed cancer and the third leading cause of cancer death, with over 1 million new cases and 783,000 deaths in 2018 globally [Citation1]. The incidence of gastric cancer has declined in recent decades due to an increase in fruit and vegetable intake and a decrease in salt intake, Helicobacter pylori infection, and tobacco smoking [Citation2,Citation3]. However, gastric cancer remains to be a considerable health burden worldwide [Citation4]. The majority of gastric cancer patients are diagnosed with metastatic or locally advanced disease, with median survival time no more than 1 year [Citation5]. Thus, it is imperative to further investigate the underlying pathogenesis of gastric cancer, which will contribute to improving the patient outcome.
Epigenetics, mainly including DNA methylation, histone modification and chromatin remodeling, does not affect the DNA sequence but mediates gene expression and activity [Citation6,Citation7]. Epigenetics has been widely studied in many biological researches such as genomic imprinting, cloning and transgenic technologies, viral latency and cancer biology [Citation8]. DNA methylation is the most common epigenetic process and consequently has received more and more attention [Citation9]. It predominantly occurs at CpG islands through adding methyl groups to DNA molecules, thereby repressing gene transcription [Citation10]. Accumulating evidence shows that aberrant DNA methylation is closely associated with cancer development [Citation11]. Gastric cancer-related gene methylation has also been reported [Citation12]. For instance, E-cadherin methylation suppresses its protein expression and thus facilitates tumorigenesis processes of gastric cancer [Citation13]. Methylation of BNIP3, a key pro-apoptotic factor, inhibits BNIP3 expression and prevents cell apoptosis, thereby leading to lower chemosensitivity of patients with gastric cancer [Citation14]. FOXF2 methylation is related to poor survival in gastric cancer patients [Citation15]. Hence, the study of DNA methylation in gastric cancer can provide promising biomarkers for the disease diagnosis, prognosis and treatment [Citation16]. However, the related research has not been fully illustrated.
Neuronal pentraxin II (NPTX2) encodes a member of the neuronal pentraxin, synaptic protein family [Citation17]. NPTX2 is associated with excitatory synapse formation, host immunity and acute inflammation [Citation18]. NPTX2 plays key roles in depression, anxiety and Alzheimer’s disease [Citation19–21]. The effects of NPTX2 on cancers have also been fully studied. NPTX2 can regulate cellular metastasis and proliferation, thereby affecting the development of colorectal carcinoma, neuroblastoma and clear cell renal cell carcinoma [Citation22–24]. Additionally, NPTX2 hypermethylation functions as a valuable target for the prognosis, diagnosis and treatment of pancreatic cancer [Citation25–27]. NPTX2 hypermethylation has also been found in glioblastoma [Citation28]. However, whether NPTX2 is involved in the progression of gastric cancer and the underlying molecular mechanism remains unclear.
Therefore, we studied the expression and methylation of NPTX2 in gastric cancer and the roles of NPTX2 in the cell cycle, apoptosis and proliferation. To further investigate the possible molecular mechanism, we determined the interaction of NPTX2 with the p53 signaling pathway in gastric cancer cells.
Materials and methods
Cell culture
Gastric cancer cell lines (HGC-27, AGS, NCI-N87 and SNU-1) were purchased from the National Collection of Authenticated Cell Cultures (Shanghai, China). Human gastric mucosal cells (GES-1) were purchased from Procell Life Science & Technology Co., Ltd. (Wuhan, China). All cell lines were incubated in a complete culture medium, including RPMI 1640 medium (Gibco), 1% penicillin and streptomycin and 10% FBS (fetal bovine serum, Gibco), and maintained in a humidified incubator with 5% CO2 at 37°C.
Cell treatment
Human gastric mucosal cells (GES-1) and gastric cancer cell lines (HGC-27, AGS, NCI-N87 and SNU-1) were incubated in a complete cultural medium and treated with DNA methyltransferase inhibitor, 5-Aza-20-deoxycytidine (5Aza-Dc, Sigma) and histone deacetylase inhibitor, trichostatin A (TSA, Sigma), either alone or in combination. As previously described [Citation29], gastric cancer cells were continuously exposed to 1 μM 5Aza-Dc for 4 days or to 1 μM TSA for 24 hours. In the control group, cells were treated with PBS alone. In 5Aza-Dc+TSA group, cells were exposed to 1 μM 5Aza-Dc for 3 days and then treated with 0.5 μM TSA for additional 24 hours.
Cell transfection
NPTX2 expression plasmid (NPTX2) and control plasmid (control) were obtained from HANBIO (Shanghai, China). shRNA NPTX2 (shNPTX2) and control shRNA (shNC) were purchased from Sigma–Aldrich. These vectors were, respectively, transfected into GES-1, HGC-27 and NCI-N87 cells using Lipofectamine™ 3000 Reagent (Invitrogen) for 48 hours.
Quantitative real-time polymerase chain reaction (qRT-PCR)
Total RNA from gastric cancer cells was isolated using TRIzol reagent (Invitrogen) based on the manufacturer’s protocol and was reverse transcribed into cDNA (complementary DNA) using a PrimeScript™ RT Master Mix kit (Takara, Dalian, China). qRT-PCR was carried out using a TB Green® Premix Ex Taq™ RT-PCR Kit (Takara) and a StepOne Plus machine (Applied Biosystems). PCR cycling conditions were listed as the following: 95°C for 5 min, 35 cycles of 95°C for 20 s, 60°C for 20 s, 72°C for 20 s, and a final extension of 4 min at 72°C. β-actin was used as the internal control. NPTX2 relative mRNA expression was calculated using the 2−ΔΔCT method with the normalization to β-actin expression. The sequences of primers are as follows: NPTX2 forward: 5ʹ-CCT CCC ACT CCG CAC AAA C-3ʹ, reverse: 5ʹ-CAC CGC ATA GGA GAA GGG G-3ʹ; β-actin forward: 5ʹ-AGC CTC GCC TTT GCC GA-3ʹ, reverse: 5ʹ-CTG GTG CCT GGG GCG-3ʹ.
Methylation-specific PCR (MS-PCR)
MS-PCR was performed to assess the methylated and unmethylated levels of the promoters of NPTX2. DNA from gastric cancer cells was extracted using DNAzol™ Reagent kit (Thermo Fisher Scientific) following the manufacturer’s protocol. The extracted DNA was treated with bisulfite using an EZ DNA Methylation-Gold Kit (Zymo Research). The bisulfite-modified DNA was amplified with primers specific for unmethylated or methylated DNA. Primer sequence was taken as described previously [Citation25]: the unmethylated NPTX2 promoter primer forward: 5ʹ-AAGAAAGGGTGTGTGGATTTG-3ʹ, reverse: 5ʹ-CCACACTATCATCTCAAAAATC-3ʹ; the methylated NPTX2 promoter primer forward: 5ʹ-GAAAGGGCGCGCGGATTC-3ʹ, reverse: 5ʹ-CGCTATCGTCTCGAAAATCG-3ʹ. The conditions of PCR are as follows: 95°C for 5 min; 40 cycles of 95°C for 20 s; 60°C for 20 s; 72°C for 30 s; and a final extension of 5 min at 72°C. The products of MS-PCR (8 μL) were performed using horizontal gel electrophoresis on 3% agarose gels. The samples were visualized under UV transillumination by a SYBR Gold Nucleic Acid Gel Stain kit (Thermo Fisher Scientific) staining.
Western blot
Gastric cell lysates were extracted using RIPA buffer containing 1% protease inhibitors and phosphatase inhibitors. Bicinchoninic acid method was used to detect the concentration of protein in cell lysates. Proteins (20 μg) were separated equally by 10% sodium dodecyl sulfate/polyacrylamide gel electrophoresis and then transferred to polyvinylidene difluoride membranes. The membranes, after blocking using 5% nonfat milk, were incubated with primary antibodies against NPTX2 (ab277523, dilution 1/1000), p53 (ab32389, dilution 1/1000), p21 (ab109199, dilution 1/1000), PTEN (ab267787, dilution 1/1000) and β-actin (ab8227, dilution 1/2000) at 4°C for overnight, washed with TBST with 0.1% Tween-20 and then cultured with horseradish peroxidase-conjugated secondary antibodies (Goat Anti-Rabbit IgG H&L, dilution 1/2000, ab205718) for 1 hour at room temperature. The above antibodies were obtained from Abcam. β-actin was applied to be a loading control. Protein bands were visualized by Pierce™ enhanced chemiluminescent substrate (Thermo Fisher Scientific). The semi-quantification of protein bands was analyzed using the ImageJ software.
Cell counting kit-8 (CCK8) assay
After transfection for 48 h, GES-1, HGC-27 and NCI-N87 cells were collected and inoculated in 96-well plates at the density of 1 × 104 cells/well. The cells were incubated in a complete culture medium for 24, 48 and 72 hours. Meanwhile, CCK-8 reagent was added to the gastric cancer cells at each time point. The mixture was then incubated for additional 4 hours and the absorbance value at 450 nm was obtained using a microplate reader (ELx808™, BioTek).
Colony formation assay
Forty-eight hours post transfection, GES-1, NCI-N87 and HGC-27 cells were harvested and resuspended in a fresh, complete medium at 200 cells/mL. The cell suspension (1 mL) was added to 24-well plates and incubated at 37°C for 7 days. The medium was changed every 3 days. After removing the culture medium, the cells were fixed at room temperature with 70% ethanol for 10 min, then stained at room temperature with 0.5% crystal violet solution for 10 min, and viewed by a bright-field microscopy. A colony with more than 50 cells is considered to be defined.
Flow cytometry
For apoptosis detection, transfected gastric cancer cells (NCI-N87 and HGC-27) were harvested and washed with PBS and then resuspended in 100 μL of 1× binding buffer solution (cell concentration: 1 × 106 cells/mL). Annexin V-FITC (5 µL) and propidium iodide (PI, 5 µL) were added to 100 μL of cell suspension for 15 min at room temperature in the dark. After 1× binding buffer (400 μL) was added to each tube, the sample was gently mixed and tested by flow cytometry.
For cell cycle detection, the transfected cells were collected and washed with PBS. Cold 70% ethanol was added dropwise to the cells. After being fixed by 70% ethanol at 4°C for 30 min, the cells were centrifuged for 6 min at 300 × g, washed twice with PBS and resuspended in PI staining solution (1 mL) for 30 min incubation at room temperature. PI staining solution contains 50 μg/mL PI, 100 μg/mL DNase-free RNase A (Sigma) in PBS and 0.1% (v/v) Triton X-100 (Sigma). After incubation, flow cytometry was utilized to measure the cell cycle.
Statistical analysis
All data from at least three repeated independent trials were presented as mean ± SD (standard deviation) and assessed using GraphPad Prism 7 software. The difference in variables between groups was analyzed by Student’s t-test or one-way ANOVA followed by Bonferroni’s multiple comparisons test. Statistical significance was considered as p < 0.05.
Results
To investigate the roles of NPTX2 in gastric cancer, we detected the expression and methylation of NPTX2 and explored the relationship between expression and methylation of NPTX2 in gastric cancer cells. Moreover, we determined the effects of NPTX2 on the proliferation, apoptosis and cell cycle of gastric cancer cells. Furthermore, we measured the relationship between NPTX2 and the p53 signaling pathway to study the mechanism of NPTX2 in gastric cancer cells.
Expression of NPTX2 in gastric cancer cells
The expression level of NPTX2 in gastric cancer cells was examined by qRT-PCR and western blot. As shown in ), NPTX2 mRNA expression was significantly down-regulated in gastric cancer cell lines (NCI-N87, HGC-27, AGS and SNU-1) in comparison with human gastric mucosal cells (GES-1). In addition, compared with GES-1 cells, NPTX2 protein level was decreased in gastric cancer cells as confirmed by western blot ()). Thus, NPTX2 expression was down-regulated in gastric cancer cells.
Figure 1. Expression of NPTX2 in gastric cancer cells. (a) The mRNA expression of NPTX2 was determined by qRT-PCR in gastric cancer cell lines (NCI-N87, HGC-27, AGS and SNU-1) and normal human gastric mucosal cells (GES-1). (b) The protein expression of NPTX2 was measured by western blot. **p < 0.01 versus GES-1 cells
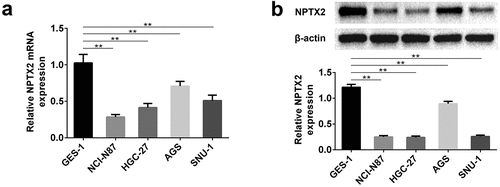
Methylation analysis of NPTX2 in gastric cancer cells
MS-PCR was applied to measure NPTX2 promoter methylation in gastric cancer cells. The analysis of MS-PCR indicated that the promoter of NPTX2 was highly hypermethylated in all four gastric cancer cell lines compared with human gastric mucosal cells (GES-1) ()). To further study the relationship between NPTX2 methylation and NPTX2 expression, we examined the expression of NPTX2 in GES-1 cells and gastric cancer cells treated with or without demethylation. As shown in ), the demethylation of NPTX2 induced by DNA methyltransferase inhibitor 5Aza-Dc obviously up-regulated NPTX2 expression in GES-1 cells and four gastric cancer cell lines compared with the control group. In addition, histone deacetylase inhibitor TSA acted synergistically with 5Aza-Dc to enhance the expression of NPTX2. Collectively, the demethylation of NPTX2 was associated with the high expression of NPTX2. Furthermore, Cancer Cell Line Encyclopedia database was applied to further validate the relationship between methylation and mRNA expression of NPTX2, and the results showed that NPTX2 methylation was negatively correlated with NPTX2 expression ()).
Figure 2. Methylation analysis of NPTX2 in gastric cancer cells. (a) The methylation of NPTX2 promoter in human gastric mucosal cells (GES-1) and gastric cancer cells (NCI-N87, HGC-27, AGS and SNU-1) was assessed by MS-PCR. (b) After GES-1 and gastric cancer cells were treated with 5Aza-Dc and TSA, either alone or in combination, the mRNA expression of NPTX2 was detected by qRT-PCR.(c)NPTX2 methylation was negatively correlated with NPTX2 expression *p < 0.05, **p < 0.01 versus control or 5Aza-Dc
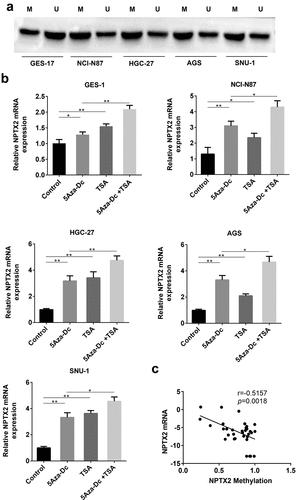
Effect of NPTX2 on the proliferation of gastric cancer cells
To assess the effect of NPTX2 on gastric cancer cell proliferation, CCK8 and colony formation assays were performed in the transfected cells. As shown in ), the protein expression of NPTX2 in GES-1, NCI-N87 and HGC-27 cells was obviously up-regulated by NPTX2 plasmid compared with the control, and shNPTX2 considerably down-regulated the protein expression of NPTX2 compared with shNC. CCK8 assay revealed that the overexpression of NPTX2 suppressed the proliferation of GES-1, NCI-N87 and HGC-27 cells compared with the control, while shNPTX2 promoted the cell proliferation comparison with shNC ()). Colony formation assay suggested that the number of colonies in the NPTX2 overexpression group was lower than that in the control group and yet shNPTX2 induced a greater number of colony formation in comparison to shNC ()). Therefore, NPTX2 suppressed cell proliferation in gastric cancer.
Figure 3. Effect of NPTX2 on the proliferation of gastric cancer cells. (a) After human gastric mucosal cells (GES-1) and gastric cancer cells (NCI-N87 and HGC-27) were, respectively, transfected with NPTX2 plasmid (NPTX2), control plasmid (control), shRNA NPTX2 (shNPTX2) and control shRNA (shNC), the protein expression of NPTX2 was examined by western blot. (b) The cell viability of the transfected cells was determined by CCK-8 assay. (c) The proliferation of the transfected cells was measured by colony formation assay. *p < 0.05, **p < 0.01 versus control or shNC
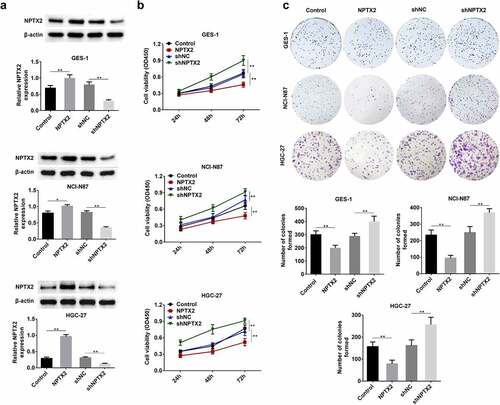
Effect of NPTX2 on gastric cancer cell apoptosis
The effect of NPTX2 on cell apoptosis in gastric cancer cells was studied using Annexin V-FITC/PI staining kit and analyzed by flow cytometry. As shown in , NPTX2 overexpression plasmid promoted apoptosis in NCI-N87 and HGC-27 cells in comparison to the control plasmid. Nevertheless, shNPTX2 inhibited apoptosis as compared to shNC. Hence, NPTX2 induced gastric cancer cell apoptosis.
Figure 4. Effect of NPTX2 on gastric cancer cell apoptosis. After gastric cancer cells (NCI-N87 and HGC-27) were, respectively, transfected with NPTX2 plasmid (NPTX2), control plasmid (control), shRNA NPTX2 (shNPTX2) and control shRNA (shNC), the apoptosis of gastric cancer cells was determined by flow cytometry using Annexin V-FITC/PI staining. **p < 0.01 versus control or shNC
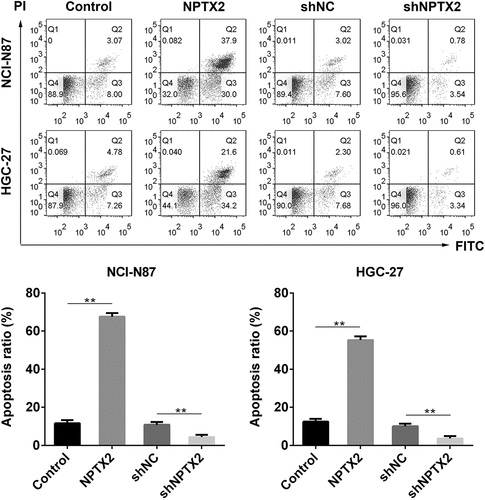
Effect of NPTX2 on cell cycle in gastric cancer cells
The effect of NPTX2 on cell cycle in gastric cancer cells was measured by flow cytometry. Compared with the control, NPTX2 overexpression plasmid increased G1-phase cells in NCI-N87 and in HGC-27 cells and decreased S-phase cells (). In addition, shNPTX2 decreased the percentage of NCI-N87 and HGC-27 cells in the G1-phase, while increased cells in the S-phase as compared to shNC (). Thus, NPTX2 in gastric cancer cells induced cell cycle arrest.
Figure 5. Effect of NPTX2 on cell cycle in gastric cancer cells. After gastric cancer cells (NCI-N87 and HGC-27) were, respectively, transfected with NPTX2 plasmid (NPTX2), control plasmid (control), shRNA NPTX2 (shNPTX2) and control shRNA (shNC), the gastric cancer cell cycle was detected by flow cytometry. *p < 0.05, **p < 0.01 versus control or shNC
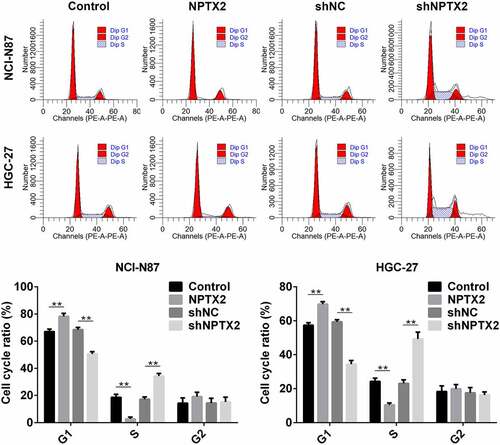
Effect of NPTX2 on the p53 signaling pathway in gastric cancer cells
To further investigate the molecular mechanism of NPTX2 in gastric cancer cells, the interaction between NPTX2 and p53 signaling pathway was assessed using western blot. Compared with the control, the expressions of key proteins (p53, p21 and PTEN) in the p53 signaling pathway were up-regulated by NPTX2 overexpression plasmid (). Additionally, shNPTX2 considerably down-regulated the protein expression of PTEN, p53 and p21 in NCI-N87 and HGC-27 cells compared with shNC (). Hence, NPTX2 could activate the p53 signaling pathway, and the related pathway mechanism diagram is shown in .
Figure 6. Effect of NPTX2 on the p53 signaling pathway in gastric cancer cells. After gastric cancer cells (NCI-N87 and HGC-27) were, respectively, transfected with NPTX2 plasmid (NPTX2), control plasmid (control), shRNA NPTX2 (shNPTX2) and control shRNA (shNC), the expression of key proteins (PTEN, p53 and p21) in the p53 signaling pathway was measured by western blot. **p < 0.01 versus control or shNC
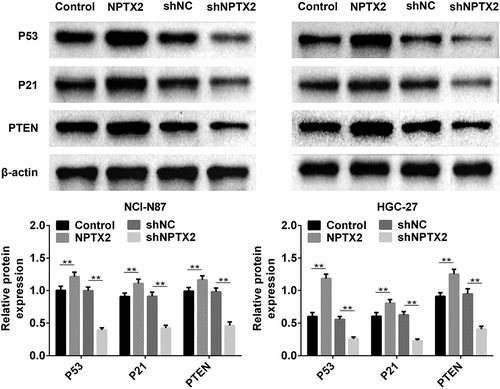
Discussion
Our study indicated that the expression of NPTX2 was down-regulated in gastric cancer cells compared with human gastric mucosal cells. The hypermethylation of NPTX2 promoter was observed in gastric cancer cells, and its methylation was negatively correlated with NPTX2 expression. In addition, downregulated expression of NPTX2 caused by hypermethylation had the promoting roles in gastric cancer cells through activating the p53 signaling pathway.
Despite a decline in gastric cancer incidence and the advances in therapeutic methods, patients with gastric cancer still have poor outcome [Citation2,Citation4]. DNA methylation, as an important epigenetic factor, is crucial for genomic imprinting, gene expression and genomic stability [Citation8]. Aberrant DNA methylation is closely related to the progression of cancers [Citation11,Citation12]. Thus, the study of DNA methylation in gastric cancer can provide new insight for improving the prognosis of the patients. NPTX2 methylation has been observed in several cancers such as pancreatic cancer and glioblastoma [Citation25–28]. However, the roles of NPTX2 in gastric cancers have not been reported. Thus, we detected the expression of NPTX2 and found its lower expression in gastric cancer cells in comparison with human gastric mucosal cells. Additionally, our work revealed that NPTX2 suppressed cell proliferation but induced apoptosis and cell cycle arrest in gastric cancer cells. Xu et al. suggest that NPTX2 is overexpressed in colorectal cancer and interacts with FZD6 to promote cellular metastasis and proliferation in colorectal cancer [Citation22]. However, Zhang et al. show that NPTX2 can function as a tumor suppressor because it suppresses the invasion and proliferation of pancreatic cancer cells [Citation27]. Collectively, it can be inferred that NPTX2 may have oncogenic or tumor suppressive functions in different cancers. The contradictory effects of NPTX2 may be associated with the cancer cell heterogeneity as well as the complexity of biological processes. Therefore, NPTX2 may be explored as a potential target for gastric cancer therapy.
The p53 signaling pathway responds to extrinsic and intrinsic stresses which can affect the fidelity of DNA replication and cell division [Citation30]. The tumor suppressor p53, a transcription factor, can regulate the target gene expression, which is critical for cell senescence, apoptosis, growth inhibition and cell cycle arrest [Citation31,Citation32]. p21, as a key target gene of p53, has been proven to control cell cycle arrest in responding to DNA damage [Citation33]. PTEN is considered as a major component of the p53 response upon DNA damage [Citation34]. P53 can increase the expression of PTEN, and PTEN prevents the p53 degradation pathway through antagonizing AKT active [Citation35]. Our study demonstrated that the upregulated expression of NPTX2 enhanced the protein expression of p53, p21 and PTEN and thus activated the p53 signaling pathway, and consequently induced cell cycle arrest, apoptosis and proliferation inhibition. In addition, knockdown of NPTX2 suppressed the activity of the p53 signaling pathway, thereby promoting cellular proliferation and inhibiting apoptosis and cell cycle arrest. Moreover, the hypermethylation of NPTX2 promoter in gastric cancer cells was observed, and its hypermethylation caused the low expression of NPTX2 in gastric cancer cells. Therefore, the downregulated expression of NPTX2 caused by hypermethylation could regulate the biological processes of gastric cancer cells by regulating the p53 signaling pathway.
Conclusion
NPTX2 hypermethylation promoted cell proliferation but inhibited apoptosis and cell cycle arrest in gastric cancer cells by suppressing the p53 signaling pathway. Thus, NPTX2 may be critical for the development of gastric cancer. These findings are useful for understanding the underlying pathogenesis of gastric cancer and provide a potential marker for the therapy of gastric cancer.
Highlight
1. Expression of NPTX2 in gastric cancer cells.
2. Methylation analysis of NPTX2 in gastric cancer cells.
3. Effect of NPTX2 on the proliferation of gastric cancer cells.
4. Effect of NPTX2 on gastric cancer cell apoptosis.
5. Effect of NPTX2 on cell cycle in gastric cancer cells.
Authors’ contributions
Guofeng Xu and Linfeng Fan designed the study and supervised the data collection. Shufeng Zhao analyzed and interpreted the data. Canhui OuYang prepared the manuscript for publication and reviewed the draft of the manuscript. All authors have read and approved the manuscript.
Disclosure statement
No potential conflict of interest was reported by the authors.
Availability of data and materials
All data generated or analyzed during this study are included in this published article.
References
- Bray F, Ferlay J, Soerjomataram I, et al. Global cancer statistics 2018: GLOBOCAN estimates of incidence and mortality worldwide for 36 cancers in 185 countries. CA Cancer J Clin. 2018;68(6):394–424.
- Nagaraja AK, Kikuchi O, Bass AJ. Genomics and targeted therapies in gastroesophageal adenocarcinoma. Cancer Discov. 2019;9(12):1656–1672.
- Ushijima T, Sasako M. Focus on gastric cancer. Cancer Cell. 2004;5(2):121–125.
- Van Cutsem E, Sagaert X, Topal B, et al. Gastric cancer. Lancet. 2016;388(10060):2654–2664.
- Shah MA. Update on metastatic gastric and esophageal cancers. J Clin Oncol. 2015;33(16):1760–1769.
- Ebrahimi V, Soleimanian A, Ebrahimi T, et al. Epigenetic modifications in gastric cancer: focus on DNA methylation. Gene. 2020;742:144577.
- Kobayashi H, Akasaka J, Koike N, et al. Preeclampsia as a parental epigenetic disease. Clin Exp Obstet Gynecol. 2018;45(4):493–502.
- Choi IS, Wu TT. Epigenetic alterations in gastric carcinogenesis. Cell Res. 2005;15(4):247–254.
- Choi SJ, Jung SW, Huh S, et al. Alteration of DNA methylation in gastric cancer with chemotherapy. J Microbiol Biotechnol. 2017;27(8):1367–1378.
- Parry A, Rulands S, Reik W. Active turnover of DNA methylation during cell fate decisions. Nat Rev Genet. 2021;22(1):59–66.
- Klutstein M, Nejman D, Greenfield R, et al. DNA methylation in cancer and aging. Cancer Res. 2016;76(12):3446–3450.
- Tahara T, Arisawa T. DNA methylation as a molecular biomarker in gastric cancer. Epigenomics. 2015;7(3):475–486.
- Tamura G. Alterations of tumor suppressor and tumor-related genes in the development and progression of gastric cancer. World J Gastroenterol. 2006;12(2):192–198.
- Sugita H, Iida S, Inokuchi M, et al. Methylation of BNIP3 and DAPK indicates lower response to chemotherapy and poor prognosis in gastric cancer. Oncol Rep. 2011;25(2):513–518.
- Higashimori A, Dong Y, Zhang Y, et al. Forkhead box F2 suppresses gastric cancer through a novel FOXF2-IRF2BPL-β-Catenin signaling axis. Cancer Res. 2018;78(7):1643–1656.
- Koch A, Joosten SC, Feng Z, et al. Analysis of DNA methylation in cancer: location revisited. Nat Rev Clin Oncol. 2018;15(7):459–466.
- Hsu YC, Perin MS. Human neuronal pentraxin II (NPTX2): conservation, genomic structure, and chromosomal localization. Genomics. 1995;28(2):220–227.
- Swanson A, Wolf T, Sitzmann A, et al. Neuroinflammation in Alzheimer’s disease: pleiotropic roles for cytokines and neuronal pentraxins. Behav Brain Res. 2018;347:49–56.
- Tang CZ, Zhang DF, Yang JT, et al. Overexpression of microRNA-301b accelerates hippocampal microglia activation and cognitive impairment in mice with depressive-like behavior through the NF-κB signaling pathway. Cell Death Dis. 2019;10(4):316.
- Chang S, Bok P, Tsai CY, et al. NPTX2 is a key component in the regulation of anxiety. Neuropsychopharmacology. 2018;43(9):1943–1953.
- Xiao MF, Xu D, Craig MT, et al. NPTX2 and cognitive dysfunction in Alzheimer’s disease. eLife. 2017;6:e23798.
- Xu C, Tian G, Jiang C, et al. NPTX2 promotes colorectal cancer growth and liver metastasis by the activation of the canonical Wnt/β-catenin pathway via FZD6. Cell Death Dis. 2019;10(3):217.
- Bartolini A, Di Paolo D, Noghero A, et al. The neuronal pentraxin-2 pathway is an unrecognized target in human neuroblastoma, which also offers prognostic value in patients. Cancer Res. 2015;75(20):4265–4271.
- Von Roemeling CA, Radisky DC, Marlow LA, et al. Neuronal pentraxin 2 supports clear cell renal cell carcinoma by activating the AMPA-selective glutamate receptor-4. Cancer Res. 2014;74(17):4796–4810.
- Singh N, Rashid S, Rashid S, et al. Clinical significance of promoter methylation status of tumor suppressor genes in circulating DNA of pancreatic cancer patients. J Cancer Res Clin Oncol. 2020;146(4):897–907.
- Zhang L, Gao J, Li Z, et al. Neuronal pentraxin II (NPTX2) is frequently down-regulated by promoter hypermethylation in pancreatic cancers. Dig Dis Sci. 2012;57(10):2608–2614.
- Zhang L, Gao J, Li L, et al. The neuronal pentraxin II gene (NPTX2) inhibit proliferation and invasion of pancreatic cancer cells in vitro. Mol Biol Rep. 2011;38(8):4903–4911.
- Shukla S, Pia Patric IR, Thinagararjan S, et al. A DNA methylation prognostic signature of glioblastoma: identification of NPTX2-PTEN-NF-κB nexus. Cancer Res. 2013;73(22):6563–6573.
- Sato N, Fukushima N, Maitra A, et al. Discovery of novel targets for aberrant methylation in pancreatic carcinoma using high-throughput microarrays. Cancer Res. 2003;63(13):3735–3742.
- Harris SL, Levine AJ. The p53 pathway: positive and negative feedback loops. Oncogene. 2005;24(17):2899–2908.
- Issaeva N. p53 signaling in cancers. Cancers (Basel). 2019;11:3.
- Khatoon E, Deka M, Saikia KK, et al. Clinical significance of β-catenin, hTERT, p53, and Wnt7A as biomarkers for ovarian cancer. Eur J Gynaecological Oncol. 2020;41(2):181–187.
- Karimian A, Ahmadi Y, Yousefi B. Multiple functions of p21 in cell cycle, apoptosis and transcriptional regulation after DNA damage. DNA Repair (Amst). 2016;42:63–71.
- Nakanishi A, Kitagishi Y, Ogura Y, et al. The tumor suppressor PTEN interacts with p53 in hereditary cancer (review). Int J Oncol. 2014;44(6):1813–1819.
- Trotman LC, Pandolfi PP. PTEN and p53: who will get the upper hand? Cancer Cell. 2003;3(2):97–99.