ABSTRACT
Ultrasound (US) is reported to improve the delivery efficiency of drugs loading onto large nanoparticles due to the sonoporation effect. Microbubbles (MBs) can be used as contrast agents of US expanding and contracting under low-amplitude US pressure waves. Ultrasound-stimulated microbubbles (USMBs) therapy is a promising option for the treatment of various cancers as a radiosensitizer. However, its role in esophageal squamous cell carcinoma (ESCC) remains unknown. In our study, human ESCC cell lines (KYSE-410, KYSE-1140) were treated with radiation solely, US alone, or radiation in combination with US or USMBs. The migration and invasion abilities of ESCC cells were examined by wound healing and Transwell assays. ESCC cell apoptosis was assessed using flow cytometry analysis and TUNEL assays. The levels of proteins associated with cell apoptosis and angiogenesis were measured by western blot analysis. A tube formation assay was performed to detect the ESCC cell angiogenesis. We found that USMBs at high levels most effectively most efficiently enhanced the effect of radiation, and significant changes in the viability (48%-51%), proliferation (1%), migration (63%-71%), invasion (52%) and cell apoptosis (31%-50%) of ESCC cells were observed compared with the control group in vitro. The ESCC angiogenesis was inhibited by US or radiation treatment and further inhibited by a combination of radiation and US or USMBs. USMBs at high levels most effectively enhanced the inhibitory effect of radiotherapy on ESCC cell apoptosis. Overall, USMBs enhanced the radiosensitivity of esophageal squamous cell carcinoma cells.
Graphical abstract
USMBs treatment enhanced the anti-tumor effect of radiation on ESCC cell proliferation, migration, invasion, angiogenesis and apoptosis in vitro.
1USMBs enhance the radiation-induced inhibition on ESCC cell growth
2USMBs promote the radiation effect on ESCC cell apoptosis
3USMBs enhance radiation-caused suppression on ESCC cell migration and invasion
4USMBs enhance the suppression of radiation on ESCC angiogenesis

Introduction
Esophageal carcinoma (EC) is one of the major causes of cancer-related deaths globally [Citation1,Citation2]. Its incidence ranks seventh with 572,000 new cases and mortality ranks sixth with 509,000 deaths worldwide in 2018 [Citation3]. Esophageal carcinoma has two histological subtypes: esophageal squamous cell carcinoma (ESCC) and esophageal adenocarcinoma (EAC). It is estimated that about 85% of EC cases are ESCC globally [Citation4]. The main risk factors for ESCC are tobacco smoking and alcohol overconsumption [Citation5], and dietary and genetic factors are also involved [Citation6,Citation7]. The overall five-year survival rate of EC has been increasing in recent years to be approximately 20% [Citation8].
Radiotherapy (RT) is one of the most potent options for treatment of various cancers. It can be utilized alone or in cooperation with chemotherapy and surgery [Citation9]. It was calculated to be 52.3% for the recommended overall optimal radiotherapy utilization rate [Citation10]. The delivery of radiotherapy can be done outside the patients, such as external-beam radiation therapy, or EBRT by implanting radioactive sources in cavities or tissues (brachytherapy), or by systemic regulation of radiopharmaceutical agents [Citation11]. Numerous investigations have revealed that radiotherapy plays a critical role in the treatment of EC [Citation12,Citation13].
As the radiotherapy is applied, the survival cancer cells can develop the radioresistance against RT. Therefore, it is essential to investigate the potential radiosensitizers [Citation14–16]. Ultrasound-stimulated microbubbles (USMBs) are spheres filled with gas in micron size, and coated by protein, lipid, or biopolymer, which are common in medical ultrasound applications [Citation17–19]. The MBs with a median diameter of 3 µm can efficiently circulate within the blood after peripheral intravenous injection [Citation20,Citation21]. Moreover, MB are mechanically perturbed by the stimulation of US, which may cause side effects, such as changes of neighboring cell membranes, tissue damage, and hemorrhage, providing clues for the cancer treatment [Citation20,Citation22].
Previous studies indicate that USMBs exert enhanced effect on the radiotherapy for cancer. For instance, USMBs are revealed to improve the prostate cancer treatment in cooperation with radiotherapy [Citation23]. USMBs are indicated to enhance the radiosensitivity of nasopharyngeal carcinoma by repressing the levels of ANG 2 and AT1R [Citation24]. The USMBs are performed to delineate response effects of blood vessel and tumor simultaneously as a diagnostic tool in radiation oncology, and provide a complement for radiation therapy [Citation25]. The USMBs treatment leads to a blood flow shutdown in the tumors, and USMBs treatments with anti-vascular impacts enhance the effects of aPD-1 therapy on tumor suppression [Citation26]. Ultrasound-mediated microbubble vascular disruption enhances the radiation response of prostate cancer in vivo [Citation27].
Microbubbles (MBs) produce oscillation or cavitation via exposure to ultrasound, which transfers mechanical stress to neighboring endothelial cell membranes. This effect perturbs cell membranes and sensitizes the endothelial cells to radiation treatment, leading to an enhanced ceramide-based radiation response that can be activated by even low radiation doses (2–6 Gy) [Citation28–30]. The effective perturbation of endothelial cells contributes to enhanced microvascular destruction that can promote the radiation response [Citation31].
Furthermore, magnetic resonance imaging helps focused ultrasound surgery find the threshold exposure dose for therapeutic purposes [Citation32]. Due to the increased permeability of endothelial cellular membranes induced by shear stress of microbubble oscillation, the delivery efficiency of molecular therapeutic targets can be improved [Citation33]. Ultrasound-targeted microbubble destruction facilitates the delivery of EGFR siRNA to squamous cell carcinoma in vivo, and significantly inhibits the tumor growth [Citation34–36].
Since the survival and clinical outcomes of treatment for ESCC are still unsatisfactory, it is imperative to improve the therapeutic means for ESCC. In our study, we hypothesized that USMBs functioned as radiosensitizer for ESCC cells. We aimed to investigate the effect of USMBs in terms of ESCC radiation response on ESCC cellular functions, which may provide clues to deal with the radioresistance in the ESCC treatment.
Materials and methods
Cell lines and cell culture
Human esophageal squamous cell carcinoma cell lines (KYSE-410, catalog number: JCRB1419; KYSE-1140, catalog number: JCRB1449) were purchased from Japanese Collection of Research Bioresources (JCRB) Cell Bank. Human Umbilical Vein Endothelial Cells (HUVECs) were provided by Shanghai Institutes for Biological Science (Shanghai, China). All these cell lines were incubated as a monolayer in 100-mm dishes in Dulbecco’s modified Eagle’s medium (DMEM; Invitrogen, Carlsbad, CA) with 10% fetal bovine serum (FBS; ScienCell, Carlsbad, CA, USA) and 1% penicillin/streptomycin (Biochrom) at 37°C in 5% CO2. About 6 × 105 cells were plated in a 25-cm2 flask about one day in advance of ultrasound exposure. Then, the microbubbles were added, and cells were instantly treated with ultrasound and then cultured for 24 h. Following these treatments, cells were collected for assays as described below. A volume of 0.6 mL per sample at a density of 2 × 106 cells/mL (culture passages 3 to 6) was prepared for the following US treatment.
Radiation
Radiotherapy was performed with an RS-2000 Pro X-ray irradiator (Rad Source Technologies, Inc., USA) with X-rays at an energy of 160 kVp and 20 mA at a dose rate of 100 cGy/min alone or in combination with US or high or low level of USMBs, determined by a Fricke dosimeter. The radiation treatment was conducted after US treatment within 2 min.
Ultrasound treatments
The ultrasound exposure was performed as previously described [Citation37]. KYSE-410 and KYSE‐1140 cells were treated with ultrasound at varying peak negative pressures (0, 1.3 and 1.7 MPa) immediately after the addition of microbubbles [Citation38]. The pressure was measured with a calibrated needle hydrophone at the transducer focus. The transducer was submerged in degased water and focused in three dimensions to the center of the sample chamber to produce −6 dB beam width of 3.3 mm and a focal length of 70 mm. ESCC cells were continuously stirred at 240 rpm to be adequately exposed to the ultrasound waves. 1-MHz transducer (Valpey Fisher, Hopkinton, MA, USA) with a power amplifier (ENI, Model 240 L RF) was used to generate 16 μs pulses for 1 min (1 kHz pulse repetition frequency, 1.6% duty cycle, 1.3 and 1.7 MPa peak negative pressures, acoustic intensity 3 W/cm2). After 6 h, the KYSE-410 and KYSE-1140 cells were measured by flow cytometry and western blot analyses. The schematic diagram of the ultrasound exposure system was shown in Supplementary .
Figure 1. USMBs enhance the repressive effect of radiotherapy on ESCC cell growth
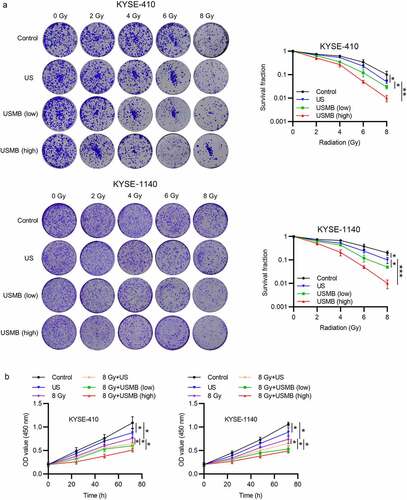
Microbubble preparation
Microbubbles were synthesized by ultrasonic dispersion of 1 mg/mL polyethylene glycol-40 stearate, 2 mg/mL 1-bisstearoyl phosphatidylcholine, 0.4 mg/mL 1,2-bisstearoyl-3-trifluoromethylpropane, and decafluorobutane (Avanti Polar Lipids Inc., Alabaster, AL, USA) in a water box. Subsequently, perfluoropropane was added in the solution which was shaken activated using Vialmix (Lantheus Medical Imaging Inc.) for 45 s until it formed a milky color. Microbubbles were mixed with 200 mg per mL of gross DMEM for 10 s and allowed it to stand for 2 min to reach a final concentration of 1 μL/mL [Citation39]. Subsequently, the clear liquid downside was removed, and the microbubble solution was gained (at a concentration of about 2.99 × 10 9 bubbles/mL with a diameter of about 2.5 μm). For the following experiments, the microbubble concentration was varied from 2.27 × 108 to 2.27 × 109 of the low concentration to the high concentration. The concentration was measured using the Accusizer 270A particle sizing instruments (Particle Sizing Systems, Santa Barbara, CA). Cells were treated with ultrasound within 30 s following the application of microbubbles. A final concentration of 1 μL/mL of culture medium was used in the assays.
CCK-8 assay
The viability of ESCC cell lines was measured using CCK-8 assay. The assay was performed using a CCK-8 kit (CCK-8, Dojindo, Japan). KYSE-410 and KYSE-1140 cell lines were plated into 96-well plates (2 × 104 cells/well) and treated with radiation (8 Gy), US, or radiation with USMBs (high or low concentration). Next, all the cell lines were cultured for 24, 48, 72 h. Then, each well was added with 10 μL of CCK-8 solution and cultured for 4 h. Microplate reader (BioTek Instruments, Winooski, VT, USA) was used to determine the absorbance at 450 nm.
Colony formation assay
KYSE-410 and KYSE-1140 cells (2 × 104 cells/well) were seeded into 6-well plates. The cells were then incubated in DMEM medium containing 10% FBS and the medium was changed every 3 days. Then, the cells were treated with different doses of radiation (0, 2, 4, 6, 8 Gy) in combination with US, or USMBs at high or low concentration. After incubation for 2 weeks, the cells were washed with PBS, fixated with 4% paraformaldehyde for 10 min at room temperature, and then stained with crystal violet (Sigma, NY, USA) for 5 min. Finally, the number of visible cell colonies was counted manually in five randomly chosen visual fields with a microscope (Olympus, Tokyo, Japan).
Flow cytometry analysis
The apoptosis of KYSE-410 and KYSE-1140 cells was assessed via the flow cytometry analysis. KYSE-410 and KYSE-1140 cells (2 × 104) were cultured in 6-well plates for 48 h and then washed with PBS and resuspended in a binding buffer. Then, the cells were treated with radiation (8 Gy), US, or radiation with USMBs (high or low concentration). Next, 5 μl of propidium iodide (PI) and 5 μl of Annexin V-fluorescein isothiocyanate were added into the buffer and incubated 15 min in dark. Afterward, the flow cytometry (BD Biosciences) was used to measure the ESCC cell apoptosis. A total of 10,000 events were collected for each group, and the proportion of early apoptosis cells (dyed with annexin V-FITC only) and the proportion of secondary necrosis cells (stained with annexin V-FITC and PI) of the total number of ESCC cells were calculated.
TUNEL assay
A TUNEL assay was used to assess the apoptosis of KYSE-410 and KYSE-1140 cells which were treated with radiation (8 Gy), US, or radiation with USMBs (high or low concentration). The cells were washed with PBS and treated with 4% paraformaldehyde for 15 min and 0.25% Triton‐X 100 for 20 min at 4°C. Subsequently, KYSE-410 and KYSE-1140 cells were subject to a TUNEL detection kit (Roche, Basel, Switzerland) and then were dyed using DAPI. The TUNEL staining of ESCC cells was observed and analyzed using an Eclipse 80i fluorescence microscopy (Nikon, Tokyo, Japan).
Western blot
All protein (20 μg) was collected from KYSE-410 and KYSE-1140 cells with radioimmunoprecipitation (RIPA) lysis buffer (Beyotime Biotechnology, Shanghai, China) containing protease inhibitor. A BCA protein assay kit (Pierce, Appleton, USA) was used to detect the concentrations of collected proteins. The proteins in the cells were isolated by SDS-PAGE and were subsequently electro-transferred to polyvinylidene fluoride (PVDF) membranes (Millipore, Boston, MA, USA). The membranes were blocked with 5% skim milk overnight at 4°C and cultured with primary antibodies, including Anti-Bcl-2 (#ab182858, 1/2000; Abcam), Anti-Bax (#ab182733, 1/2000; Abcam), Anti-Cleaved-caspase-3 (#ab2302, 1/500; Abcam), Anti-Ang 1 (#ab183701, 1/10,000; Abcam), Anti-VEGFA (#ab214424, 1/1000; Abcam), Anti-EGFR (#ab52894, 1/1000; Abcam). GAPDH served as an internal control. After washing three times with TBST, the membranes were then incubated with the second antibody at room temperature for 1 h and imaged by Gel imager (Gel Doc XR, Bio-Rad, Hercules, CA, USA). The detection system of enhanced chemiluminescence (ECL; Biomiga, Inc., San Diego, California, USA) was used to visualize the protein signals.
Wound healing assay
A wound healing assay was used to assess the migration ability of ESCC cells in vitro. The KYSE-410 and KYSE-1140 cells were plated into 6-well plates and treated with radiation (8 Gy), US, or radiation with USMBs (high or low concentration). When the cells reached the confluency of 80%, the plate was scratched by a sterile 10-μl pipette tip. Cell debris was removed by changing the medium. The images were photographed at 0 h and 24 h by a microscope.
Transwell assay
The invasion ability of KYSE-410 and KYSE-1140 cells was assessed by a Transwell assay. 2 × 104 cells were seeded into the chambers coated with Matrigel (BD Biosciences, Bedford, MA, USA) between the upper and lower chambers and treated with radiation (8 Gy), US, or radiation with USMBs (high or low concentration). The upper chambers were added with DMEM medium, while DMEM medium containing 10% FBS was added to the lower chambers. After 24 h, ESCC cells were fixed with methanol and then dyed with crystal violet. Then, stained ESCC cells were calculated under a light microscope (Olympus). A digital microscope was applied to take images. The number of invaded or migrated cells was counted in five randomly chosen visual fields.
Tube forming assay
To perform the tube formation assay, 96-well plates were pre-cooled with 50 μl Matrigel (BD Biosciences) per well, and then incubated for 1 h at room temperature. The ESCC cells were treated with radiation (8 Gy), US, or radiation with USMBs (high or low concentration). HUVECs (2 × 104) were suspended in 200 μl of conditioned medium of KYSE-410 and KYSE-1140 cells in indicated groups and then incubated at room temperature for 8 h. Finally, the tubes that formed were imaged and the number of tubes per photo was counted under a microscope.
Statistical analysis
All experiments in our study were performed in triplicate. The data were presented as the mean ± SD. The statistical analyses were performed with GraphPad Prism 7 (Graph-Pad Software, Inc., La Jolla, CA). Differences between the two groups were analyzed by Student’s t-test and differences among multiple groups were evaluated by one-way ANOVA. p < 0.05 was regarded as statistically significant.
Result
USMBs are promising radiosensitizers for the treatment of various cancers. This study hypothesized that USMBs may enhance the radiation response of ESCC cells in vitro. The cellular functions including proliferation, migration, invasion, angiogenesis and apoptosis of ESCC cells were explored via a series of functional assays under the combined treatment of USMBs with radiation.
USMBs enhance the repressive effect of radiotherapy on ESCC cell growth
To detect the response of ESCC cells to radiotherapy combined with US or USMBs, the ESCC cell lines were exposed to different doses of radiation (0, 2, 4, 6, 8 Gy) with US or low or high level of USMBs (Pneg = 1.3 MPa). Colony formation assay results showed that proliferation of ESCC cells was inhibited by the increased radiation levels, and radiation at 8 Gy exerted the most significant inhibitory effects. The 10% proliferation was observed in KYSE-410 and 19% in KYSE-1140 cells, approximately five-ten-fold decrease in ESCC cell proliferation. Application of US further suppressed the proliferation of radiation exposed ESCC cells. Moreover, cells treated with USMBs showed suppressed proliferation ability compared with cells treated with US (5% proliferation), and high dose of USMBs (1% proliferation) exerts more significant effects than low dose of USMBs (3% proliferation) on proliferation of both KYSE-410 and KYSE-1140 cells (). The viability of ESCC cells was measured by a CCK-8 assay, and the result indicated that cell viability of 88% or 89% was observed in the US group, and 76% or 74% in radiation (8 Gy) treatment group in KYSE-410 and KYSE-1140 cells, and radiation showed better suppressive effect. Moreover, US further reduced the viability of ESCC cells exposed to 8 Gy radiation to 64%-66%. The lowest cell viability was observed in the group of USMBs (high level) in combination with 8 Gy radiation (48%-51%), while the USMBs at low level (53%-60%) presented better suppressive effect compared with the US in combination with radiation (8 Gy) group ().
USMBs promote the ESCC cell apoptosis induced by radiation
Flow cytometry analysis and TUNEL assay showed that ESCC cell apoptosis was increased approximately by 17%-25% after radiation exposure (8 Gy) or by 8%-15% under the treatment of US, and the enhancement of radiation on ESCC apoptosis was better than US. The combination of radiation and US showed stronger promotive effects (20%-34%) on ESCC apoptosis than either US alone or radiation alone. USMBs with radiation most efficiently promoted cell apoptosis in a concentration dependent way. An apoptosis rate of 22%-39% was observed in the 8 Gy+ USMB (low level) group, while 31%-50% apoptosis was detected in the 8 Gy+ USMB (high level) group (. The levels of apoptosis-associated proteins were detected by western blot, which revealed that expression of Cleaved caspase 3 and Bax was increased by radiation or US treatment and further elevated by the combination of radiation and US or USMBs, radiation (8 Gy) exerted more effective enhancement on the expression of Cleaved caspase 3 and Bax compared with US alone, and USMBs induced elevated expression of Cleaved caspase 3 and Bax compared with US on radiation (8 Gy)-exposed ESCC cells, while the levels of Bcl-2 showed opposite changes in expression (.
Figure 2. USMBs promote the ESCC cell apoptosis induced by radiation
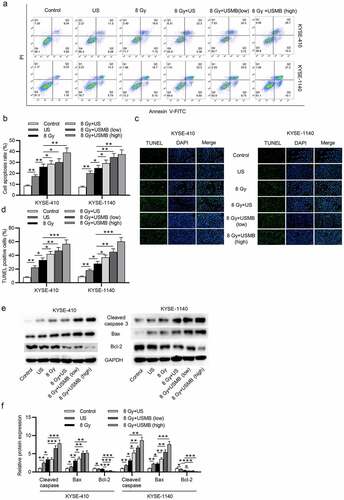
USMBs facilitate the inhibitory effect of radiation on cell migration and invasion in ESCC
Wound healing assay was used to detect the ESCC cell migration. The results revealed that the migration capacity of ESCC cells was reduced by 11%-12% after US treatment or around 26% under radiation exposure (8 Gy), and successively decreased by 38%-43% under the combination of US and radiation treatment. USMBs enhanced the effect of radiation on cell migration in a concentration-dependent way. High level of USMBs in combination with radiation (8 Gy) reduced ESCC cell migration by 63%-71% (. The ESCC cell invasion was examined by a Transwell assay, which showed that US treatment inhibited the invasion of ESCC cells by 15%-16% and radiation exposure (8 Gy) suppressed ESCC cell invasion by 26%-28%. Radiations showed stronger suppression compared with US alone. The combination of US and radiation showed a better suppressive effect (36%-39%) than either US alone or radiation alone. However, the ESCC cell invasion was most effectively reduced by 52% after the treatment of high levels of USMBs and radiation (8 Gy), corresponding to a two-fold decrease in invasion. Although low levels of USMBs and radiation showed enhanced inhibition on cell invasion compared with US and radiation group, the high levels of USMBs showed stronger synthetic effect in combination with radiation (.
Figure 3. USMBs facilitate the inhibitory effect exerted by radiation on migration and invasion of ESCC cells
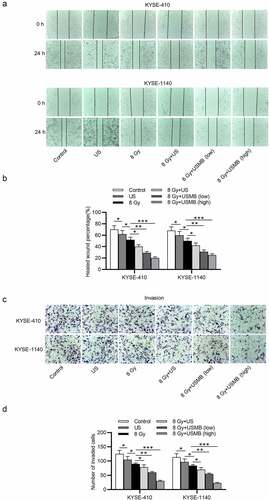
USMBs enhance the suppressive effect of radiation on angiogenesis of ESCC cells
The angiogenesis of HUVECs co-incubated with the conditioned medium of KYSE-410 and KYSE-1140 cells was assessed by a tube formation assay, and the result showed that the number of tubes was reduced by radiation exposure (29%-35%) or US treatment (11%-19%). The application of US (46%-55%) or USMBs further enhanced the suppressive effect of radiation on HUVEC angiogenesis, and USMBs at high levels group (72%-73%) showed most significant inhibition compared with low levels of USMBs (54%-68%) (. Previous studies suggest that angiogenesis is closely associated with the cancer progression [Citation40]. Angiopoietins (Ang) 1, vascular endothelial growth factor A (VEGFA) and epidermal growth factor receptor (EGFR) are factors closely related to angiogenesis. Western blot analysis was used to measure the levels of angiogenesis-associated proteins (Ang 1, VEGFA, EGFR) in ESCC cells, and the results revealed that levels of Ang1, VEGFA and EGFR were reduced by the treatment of US or the radiation exposure (8 Gy), and they were more downregulated after radiation treatment than US. The protein levels were further decreased by the combination of radiation and US or increased level of USMBs, and USMBs with radiation treatment showed better inhibitory effect than US with radiation while high levels of USMBs showed enhanced inhibition compared with low levels of USMBs (.
Figure 4. USMBs enhance the suppressive effect of radiation on angiogenesis of ESCC cells
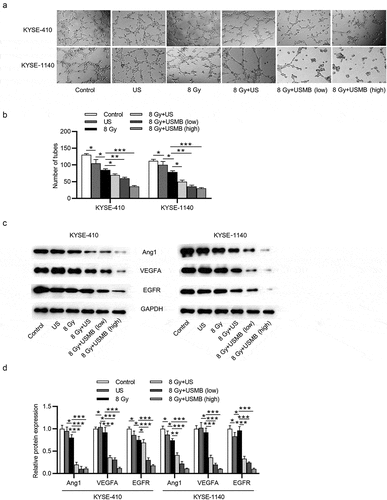
Discussion
Radiotherapy is one of the most effective and practical methods for cancer treatment. Palliative radiotherapy is frequently used to relieve pain or mass effect in the treatment of primary tumors or metastatic deposits. In the present study, radiotherapy was revealed to suppress ESCC cell proliferation, migration, invasion and angiogenesis and facilitate the ESCC cell apoptosis.
To overcome the radioresistance obtained by survival ESCC cells in the process of radiotherapy, it is imperative to develop effective radiosensitizers. In the present study, we investigate the effect of USMBs on the radioresistance of ESCC cells. The findings revealed that US and USMBs can both enhance the inhibitory effect of radiotherapy on proliferation, migration, invasion and angiogenesis of tumor cells, and USMBs exhibited more effective suppression on ESCC cells exposed to radiation than US. Thus, due to the high efficiency, the application of USMBs at high concentration is recommended to enhance the radiation response of ESCC.
USMBs have been investigated to function as radiosensitizers in nasopharyngeal carcinoma [Citation24]. It is also reported that USMBs can improve the inhibitory effect of radiation on cancer development [Citation25,Citation41]. The surrounding cells and the adjacent microvessels can be damaged by oscillation and cavitation of microbubbles [Citation27]. The released energy can induce gene expression changes, cell death, and vascular shutdown on those surrounding cells at the physiological levels [Citation25,Citation42]. Moreover, previous studies indicate that USMBs can trigger the apoptosis of cancer cells in vitro [Citation24,Citation43,Citation44]. In the present study, the ESCC cell apoptosis was promoted by either US treatment alone or radiation alone, and the application of high levels of USMBs in combination with radiation showed the most efficient promotion of cell apoptosis. Radiation showed stronger promotive effect on ESCC cell apoptosis compared with US. High levels of USMBs were demonstrated to be more effective in inducing radiosensitivity compared with USMBs at low levels. Therefore, the combination of USMBs with radiation treatment may provide us with a valuable and promising cancer treatment modality. In terms of tumor control, the application of USMBs potentially enhances the effect of lower radiation doses comparable to that of higher radiation doses.
The abnormally developed angiogenesis can cause various health risks, cancer included [Citation40], for example, pancreatic cancer [Citation45], breast cancer [Citation46], colon cancer [Citation47]. Thus, tumor blood vessels are critical therapeutic targets for radiation treatment of cancers [Citation48,Citation49]. Tumor progression can be inhibited by therapeutic methods targeting angiogenesis before clinical manifestation [Citation50]. In our study, we used HUVEC experimental model system and demonstrated that angiogenesis of ESCC cells was inhibited by radiation alone or US treatment alone. The most effective inhibition on ESCC angiogenesis was observed in the USMBs (high level) in radiation treated ESCC cells. This result was in consistent with the findings of previous studies [Citation51].
The findings of this study may expand our knowledge of the function and application of USMBs in ESCC treatment. However, this is a preliminary study using only in vitro data of ESCC cells, and future study is required to explore the effect of USMBs on radiation in ESCC tumor models in vivo. The underlying mechanism of how USMBs enhance radiation response on ESCC cellular functions also needs further investigation.
Conclusion
In conclusion, this study is the first exploration of the application of USMBs in combination with radiation therapy of ESCC. USMBs at high levels most efficiently enhanced the suppressive effects of radiation on ESCC cell proliferation, viability, migration, invasion and angiogenesis and the promoting effect on ESCC cell apoptosis. The synergistic effect of radiation and USMBs presented significantly enhanced suppression on ESCC cellular functions compared with radiation alone. Therefore, USMBs are promising target agents for the improvement of radiation response of ESCC cells.
Disclosure of potential conflicts of interest
No potential conflict of interest was reported by the author(s).
Supplemental Material
Download ()Acknowledgements
Not applicable.
Supplementary material
Supplemental data for this article can be accessed here
References
- Fitzmaurice C, Abate D, Abbasi N, et al. Global, regional, and national cancer incidence, mortality, years of life lost, years lived with disability, and disability-adjusted life-years for 29 cancer groups, 1990 to 2017: a systematic analysis for the global burden of disease study. JAMA Oncol. 2019 Dec 1;5(12):1749–1768. PubMed PMID: 31560378; PubMed Central PMCID: PMCPMC6777271 National Council for Scientific and Technological Development. Dr Haro reports being a contractor for Eli Lilly and Company and receiving personal fees from Lundbeck. Mr Hidru reports receiving grants, personal fees, nonfinancial support, and other support from Adigrat University. Dr Iseh reports receiving nonfinancial support from the Usmanu Danfodiyo University Teaching Hospital and the Institute of Human Virology, Nigeria. Dr Jakovljevic reports receiving grants from the Ministry of Education, Science and Technological Development of the Republic of Serbia. Dr James reports receiving grants from Sanofi Pasteur. Dr Lazarus reports receiving grants and personal fees from AbbVie, Gilead Sciences, and MSD. Dr Meretoja reports receiving grants from the Cancer Foundation Finland. Dr Moradi-Lakeh reports being a consultant for a project funded by Novartis. Dr Postma reports receiving grants from Quintiles and Bayer; personal fees from AbbVie, Astellas, and Pharmerit; grants and personal fees from Bristol-Myers Squibb, AstraZeneca, Sanofi, Novartis, Janssen, GlaxoSmithKline, Pfizer, MSD, and Asc Academics, as well as serving as an advisor for the organization; and earnings from stock in Ingress Health and PAB BV. Dr Savic reports being an employee of the GlaxoSmithKline group of companies and holds restricted shares in the GlaxoSmithKline group of companies. Dr J. Singh reports receiving personal fees from serving as a consultant for Crealta/Horizon, Medisys, Fidia, UBM LLC, Medscape, WebMD, the National Institutes of Health, and the American College of Rheumatology; earnings from stocks in the Amarin Corporation and Viking Therapeutics; and nonfinancial support from serving as a member of OMERACT, the US Department of Veterans Affairs Rheumatology Field Advisory Committee, and committees of the American College of Rheumatology. No other disclosures are reported. eng.
- Kamangar F, Nasrollahzadeh D, Safiri S; The global, regional, and national burden of oesophageal cancer and its attributable risk factors in 195 countries and territories, 1990–2017. a systematic analysis for the global burden of disease study 2017. Lancet Gastroenterol Hepatol. 2020 Jun;5(6):582–597. PubMed PMID: 32246941; PubMed Central PMCID: PMCPMC7232026.eng.
- Bray F, Ferlay J, Soerjomataram I,et al. Global cancer statistics 2018: GLOBOCAN estimates of incidence and mortality worldwide for 36 cancers in 185 countries. CA Cancer J Clin. 2018 Nov;68(6):394–424. PubMed PMID: 30207593.
- Arnold M, Laversanne M, Brown LM,et al. Predicting the future burden of esophageal cancer by histological subtype: international trends in incidence up to 2030. Am J Gastroenterol. 2017 Aug;112(8):1247–1255. PubMed PMID: 28585555; eng.
- Prabhu A, Obi KO, Rubenstein JH. The synergistic effects of alcohol and tobacco consumption on the risk of esophageal squamous cell carcinoma: a meta-analysis. Am J Gastroenterol. 2014 Jun;109(6):822–827. PubMed PMID: 24751582; eng.
- Liu J, Wang J, Leng Y, et al. Intake of fruit and vegetables and risk of esophageal squamous cell carcinoma: a meta-analysis of observational studies. Int J Cancer. 2013 Jul 15;133(2):473–485. PubMed PMID: 23319052; eng.
- Qu X, Ben Q, Jiang Y. Consumption of red and processed meat and risk for esophageal squamous cell carcinoma based on a meta-analysis. Ann Epidemiol. 2013 Dec;23(12):762–770.e1. PubMed PMID: 24176821; eng.
- Lagergren J, Smyth E, Cunningham D, et al. Oesophageal cancer. Lancet. 2017 Nov 25;390(10110):2383–2396. PubMed PMID: 28648400; eng.
- Liu X, Zhang Y, Tang LL, et al. Characteristics of radiotherapy trials compared with other oncological clinical trials in the past 10 years. JAMA Oncol. 2018 Aug 1;4(8):1073–1079. PubMed PMID: 29799987; PubMed Central PMCID: PMCPMC6143047 support from Varian Medical Systems. No other disclosures were reported. eng.
- Delaney G, Jacob S, Featherstone C, et al. The role of radiotherapy in cancer treatment: estimating optimal utilization from a review of evidence-based clinical guidelines. Cancer. 2005 Sep 15;104(6):1129–1137. PubMed PMID: 16080176; eng.
- Citrin DE. Recent developments in radiotherapy. N Engl J Med. 2017 Sep 14;377(11):1065–1075. PubMed PMID: 28902591; eng.
- Gao X-S. Treatment guideline of radiotherapy for Chinese esophageal carcinoma (draft). Chin J Cancer. 2010 Oct;29(10):855–859. PubMed PMID: 20868554; eng.
- Pennathur A, Gibson MK, Jobe BA, et al. Oesophageal carcinoma. Lancet. 2013 Feb 2;381(9864):400–412. PubMed PMID: 23374478; eng.
- Chen Y, Gao P, Wu T, et al. Organelle-localized radiosensitizers. Chem Commun (Camb). 2020 Sep 15;56(73):10621–10630. PubMed PMID: 32930179; eng.
- Gill MR, Vallis KA. Transition metal compounds as cancer radiosensitizers. Chem Soc rev. 2019 Jan 21;48(2):540–557. PubMed PMID: 30499573; eng.
- Moding EJ, Kastan MB, Kirsch DG. Strategies for optimizing the response of cancer and normal tissues to radiation. Nat Rev Drug Discov. 2013 Jul;12(7):526–542. PubMed PMID: 23812271; PubMed Central PMCID: PMCPMC3906736. eng.
- Qin S, Caskey CF, Ferrara KW. Ultrasound contrast microbubbles in imaging and therapy: physical principles and engineering. Phys Med Biol. 2009 Mar 21;54(6):R27–57. PubMed PMID: 19229096; PubMed Central PMCID: PMCPMC2818980. eng.
- Helfield BA. Review of phospholipid encapsulated ultrasound contrast agent microbubble physics. Ultrasound Med Biol. 2019 Feb;45(2):282–300. PubMed PMID: 30413335; eng.
- Upadhyay A, Dalvi SV. Microbubble formulations: synthesis, stability, modeling and biomedical applications. Ultrasound Med Biol. 2019 Feb;45(2):301–343. PubMed PMID: 30527395; eng.
- Huang P, You X, Pan M, et al. A novel therapeutic strategy using ultrasound mediated microbubbles destruction to treat colon cancer in a mouse model. Cancer Lett. 2013 Jul 10;335(1):183–190. PubMed PMID: 23415737; eng.
- Lin CY, Huang YL, Li JR,et al. Effects of focused ultrasound and microbubbles on the vascular permeability of nanoparticles delivered into mouse tumors. Ultrasound Med Biol. 2010 Sep;36(9):1460–1469. PubMed PMID: 20800173; eng.
- Sharma D, Giles A, Hashim A, et al. Ultrasound microbubble potentiated enhancement of hyperthermia-effect in tumours. PLoS One. 2019;14(12):e0226475. PubMed PMID: 31851698; PubMed Central PMCID: PMCPMC6919613. eng.
- Klein J, Tran W, Lai P, et al. Effect of treatment sequencing on the tumor response to combined treatment with ultrasound-stimulated microbubbles and radiotherapy. J Ultrasound Med. 2020 Jun 11;12:2415–2425. PubMed PMID: 32525248; eng. DOI:10.1002/jum.15363.
- Deng H, Cai Y, Feng Q, et al. Ultrasound-stimulated microbubbles enhance radiosensitization of nasopharyngeal carcinoma. Cell Physiol Biochem. 2018;48(4):1530–1542. PubMed PMID: 30071515; eng.
- El Kaffas A, Gangeh MJ, Farhat G, et al. Tumour vascular shutdown and cell death following ultrasound-microbubble enhanced radiation therapy. Theranostics. 2018;8(2):314–327. PubMed PMID: 29290810; PubMed Central PMCID: PMCPMC5743550. eng.
- Bulner S, Prodeus A, Gariepy J, et al. Enhancing checkpoint inhibitor therapy with ultrasound stimulated microbubbles. Ultrasound Med Biol. 2019 Feb;45(2):500–512. PubMed PMID: 30447880; eng.
- Czarnota GJ, Karshafian R, Burns PN, et al. Tumor radiation response enhancement by acoustical stimulation of the vasculature. Proceedings of the National Academy of Sciences of the United States of America. 2012 Jul 24;109( 30):E2033–41. PubMed PMID: 22778441; PubMed Central PMCID: PMCPMC3409730. eng.
- Li J, Xi A, Qiao H, et al. Ultrasound-mediated diagnostic imaging and advanced treatment with multifunctional micro/nanobubbles. Cancer Lett. 2020 Apr 10;475:92–98. PubMed PMID: 32032678; eng.
- El Kaffas A, Czarnota GJ. Biomechanical effects of microbubbles: from radiosensitization to cell death. Future oncol (London, England). 2015;11(7):1093–1108. PubMed PMID: 25804124; eng.
- Fuks Z, Kolesnick R. Engaging the vascular component of the tumor response. Cancer Cell. 2005 Aug;8(2):89–91. PubMed PMID: 16098459; eng.
- Kolesnick R. The therapeutic potential of modulating the ceramide/sphingomyelin pathway. J Clin Invest. 2002 Jul;110(1):3–8. PubMed PMID: 12093880; PubMed Central PMCID: PMCPMC151041. eng.
- McDannold NJ, King RL, Jolesz FA,et al. Usefulness of MR imaging-derived thermometry and dosimetry in determining the threshold for tissue damage induced by thermal surgery in rabbits. Radiology. 2000 Aug;216(2):517–523. PubMed PMID: 10924580; eng.
- Helfield B, Chen X, Watkins SC, et al. Biophysical insight into mechanisms of sonoporation. Proceedings of the National Academy of Sciences of the United States of America. 2016 Sep 6;113( 36):9983–9988. PubMed PMID: 27551081; PubMed Central PMCID: PMCPMC5018802. eng.
- Carson AR, McTiernan CF, Lavery L, et al. Ultrasound-targeted microbubble destruction to deliver siRNA cancer therapy. Cancer Res. 2012 Dec 1;72(23):6191–6199. PubMed PMID: 23010078; PubMed Central PMCID: PMCPMC3654825. eng.
- Miller DL. Overview of experimental studies of biological effects of medical ultrasound caused by gas body activation and inertial cavitation. Prog Biophys Mol Biol. 2007 Jan-Apr;93(1–3):314–330. PubMed PMID: 16989895; eng.
- Kremkau FW. Cancer therapy with ultrasound: a historical review. J of clin ultrasound: JCU. 1979Aug; 7(4): 287–300. PubMed PMID: 112118; eng.
- Acconcia C, Leung BY, Manjunath A,et al. Interactions between individual ultrasound-stimulated microbubbles and fibrin clots. Ultrasound Med Biol. 2014 Sep;40(9):2134–2150. PubMed PMID: 24882525; eng.
- Tarapacki C, Kumaradas C, Karshafian R. Enhancing laser thermal-therapy using ultrasound-microbubbles and gold nanorods of in vitro cells. Ultrasonics. 2013 Mar;53(3):793–798. PubMed PMID: 23290827; eng.
- Feril LB Jr., Kondo T, Zhao QL,et al. Enhancement of ultrasound-induced apoptosis and cell lysis by echo-contrast agents. Ultrasound Med Biol. 2003 Feb;29(2):331–337. PubMed PMID: 12659921; eng.
- Folkman J. What is the evidence that tumors are angiogenesis dependent? J Natl Cancer Inst. 1990 Jan 3;82(1):4–6. PubMed PMID: 1688381; eng.
- McNabb E, Al-Mahrouki A, Law N, et al. Ultrasound-stimulated microbubble radiation enhancement of tumors: single-dose and fractionated treatment evaluation. PloS One. 2020;15(9):e0239456. PubMed PMID: 32976517; PubMed Central PMCID: PMCPMC7518623. eng.
- Al-Mahrouki AA, Karshafian R, Giles A,et al. Bioeffects of ultrasound-stimulated microbubbles on endothelial cells: gene expression changes associated with radiation enhancement in vitro. Ultrasound Med Biol. 2012 Nov;38(11):1958–1969. PubMed PMID: 22980406; eng.
- Goertz DE, Todorova M, Mortazavi O, et al. Antitumor effects of combining docetaxel (taxotere) with the antivascular action of ultrasound stimulated microbubbles. PloS One. 2012;7(12):e52307. PubMed PMID: 23284980; PubMed Central PMCID: PMCPMC3527530. eng.
- FERIL, Jr. LB Jr., Kondo T. Biological effects of low intensity ultrasound: the mechanism involved, and its implications on therapy and on biosafety of ultrasound. J Radiat Res. 2004 Dec;45(4):479–489. PubMed PMID: 15635256; eng.
- Li S, Xu HX, Wu CT,et al. Angiogenesis in pancreatic cancer: current research status and clinical implications. Angiogenesis. 2019 Feb;22(1):15–36. PubMed PMID: 30168025; eng.
- Xiong J, Yang Q, Li J,et al. Effects of MDM2 inhibitors on vascular endothelial growth factor-mediated tumor angiogenesis in human breast cancer. Angiogenesis. 2014 Jan;17(1):37–50. PubMed PMID: 23907365; eng.
- Unterleuthner D, Neuhold P, Schwarz K,et al. Cancer-associated fibroblast-derived WNT2 increases tumor angiogenesis in colon cancer. Angiogenesis. 2020 May;23(2):159–177. PubMed PMID: 31667643; PubMed Central PMCID: PMCPMC7160098. eng.
- Garcia-Barros M, Paris F, Cordon-Cardo C, et al. Tumor response to radiotherapy regulated by endothelial cell apoptosis. Science (New York, NY). 2003 May 16;300(5622):1155–1159. PubMed PMID: 12750523; eng.
- Kolesnick R, Fuks Z. Radiation and ceramide-induced apoptosis. Oncogene. 2003 Sep 1;22(37):5897–5906. PubMed PMID: 12947396; eng. .
- Albini A, Tosetti F, Li VW,et al. Cancer prevention by targeting angiogenesis. Nat Rev Clin Oncol. 2012 Sep;9(9):498–509. PubMed PMID: 22850752; eng.
- Al-Mahrouki AA, Iradji S, Tran WT,et al. Cellular characterization of ultrasound-stimulated microbubble radiation enhancement in a prostate cancer xenograft model. Dis Model Mech. 2014 Mar;7(3):363–372. PubMed PMID: 24487407; PubMed Central PMCID: PMCPMC3944496. eng.