ABSTRACT
Oral candidiasis is one of the most common types of fungal infection caused by Candida albicans (C. albicans). The present study aims to investigate the antifungal effects of phloretin (a dihydrochalcone flavonoid) against the C. albicans pathogenicity. In this work, we treated C. albicans SC5314 with 37.28, 74.55, or 149.10 μg/mL (equivalent to 0.5×, 1× or 2× MIC) phloretin in vitro. Besides, we established a mice model of oral candidiasis by a sublingual infection of C. albicans suspension (1 × 107 colony-forming unit/mL), and mice were treated with phloretin (3.73 or 7.46 mg/mL, which were equivalent to 50× or 100× MIC) twice a day starting on day one post-infection. The results showed that the MIC of phloretin against C. albicans was 74.55 μg/mL. Phloretin exerted antifungal activity by inhibiting the biofilm formation and suppressing the yeast-to-hyphae transition upon the downregulation of hypha-associated genes including enhanced adherence to polystyrene 1, the extent of cell elongation gene 1, hyphal wall protein 1 gene, and agglutinin-like sequence gene 3. Next, phloretin repressed the secretion of proteases and phospholipases via reducing the expression of protease-encoding genes secreted aspartyl proteases (SAP)1 and SAP2, as well as phospholipase B1. Subsequently, the in vivo antifungal activity of phloretin was testified by the reverse of the enhanced lesion severity, inflammatory infiltration, and the increased colony-forming unit counts caused by C. albicans of tongue tissues in oral candidiasis mice. In conclusion, phloretin suppressed the pathogenicity and virulence factors against C. albicans both in vivo and in vitro.
Introduction
The incidence of fungal infection has increased continuously owing to the widespread use of hormones, immunosuppressants, and broad-spectrum antibiotics [Citation1]. Oral candidiasis is one of the most common types of oral mucosal infection caused by the yeast-like fungus Candida [Citation2]. Infants and the elderly with poor immunity are susceptible to Candida infection [Citation2]. Oral candidiasis may develop into severe stomatitis and even cause life-threatening bloodstream infection, as well as tissue infection [Citation3]. At present, azoles, polyenes, and echinocandins are known as the commonly used drugs for the treatment of oral candidiasis [Citation4]. The widespread use of these drugs may lead to the aggravation of multidrug resistance and markedly reduce the drug efficacy with severe side effects as wells as high cost [Citation5,Citation6]. Thus, there is an urgent need to explore new antifungal agents for the treatment of oral infections caused by Candida.
Candida albicans (C. albicans) is considered an opportunistic pathogen of oral candidiasis and is a polymorphic fungus that can grow as oval budding yeasts, as true hyphae, or as elongated ellipsoid cells with pseudohyphae [Citation7]. All the three forms of C. albicans could be observed in infected tissues [Citation7]. The infecting ability of C. albicans is supported by wide range of virulence factors such as, the expression of adhesins and invasions on the cell surface, the secretion of hydrolytic enzymes (proteases, phospholipases, and lipases), biofilm formation, and the transition from yeast to hyphae form (more invasive form) [Citation8]. Accordingly, the inhibition of these virulence traits is considered an effective policy of oral candidiasis therapy.
Due to the advantages of wide sources, cost effectiveness and lesser toxicity, natural compounds show broad application prospects in antifungal therapy [Citation9]. Phloretin is a dihydrochalcone flavonoid extracted from apples and strawberries and is known for its potent antioxidant, anticancer, and anti-inflammatory characteristics [Citation10–12]. Accumulating evidence has reported that phloretin suppresses the growth, virulence, and the biofilm formation of gram-negative bacteria (Acinetobacter baumannii and Escherichia coli) as well as gram-positive bacteria (Streptococcus pyogenes and Staphylococcus aureus) [Citation13–16]. These studies indicate the excellent antibacterial activity of phloretin. Moreover, Shim et al [Citation17]. have found that phloretin shows in vitro antifungal activity against some plant pathogenic fungi including Phytophthora capsici, Alternaria panax, and Sclerotinia sclerotiorum. Based on the antimicrobial function of phloretin, we speculate that it may play a role in the pathogenic process of C. albicans. However, its specific action on the virulence traits of C. albicans has not been reported.
The present study aimed to investigate the antifungal effects of phloretin against the C. albicans pathogenicity. We considered whether phloretin could abrogate virulence factors such as, biofilm formation, yeast-to-hyphae transition, and the secretion of hydrolases in vitro. Moreover, a mice model of oral candidiasis was established to explore the effectiveness of topical treatments of phloretin on the lesion severity and the inflammatory infiltration of tongue tissues in vivo.
Materials and methods
Strain and culture conditions
C. albicans strain SC5314 was purchased from National Center for Medical Culture Collections (Beijing, China). The strain, from stock stored at −80°C, was streaked on yeast extract-peptone-dextrose (YPD) agar plates (1% yeast extract, 2% peptone, 2% dextrose and 2% agar) and cultured at 37°C overnight. A loopful of colonies was inoculated into liquid YPD medium and incubated at 30°C at 180 rpm overnight. C. albicans cells were adjusted to a final density of 2.5 × 103 CFU/mL, 1 × 105 CFU/mL, 5 × 105 CFU/mL or 1 × 106 CFU/mL with RPMI-1640 medium (Solarbio, Beijing, China) supplemented with 165 mM morpholine propane sulfonic acid (MOPS, Aladdin, Shanghai, China) at pH 7.0 for subsequent experiments.
Antifungal susceptibility assay
The experiment was performed based on the CLSI-M27-A3 methodology [Citation18]. Briefly, 100 μL C. albicans suspension (2.5 × 103 colony-forming unit (CFU)/mL) was added into 96-well plates per well. C. albicans were incubated with an equal volume of two-fold serial dilutions of phloretin (Aladdin; purity ≥ 98%) at a final concentration of 5 μg/mL, 10 μg/mL, 20 μg/ml, 40 μg/mL, or 80 μg/mL at 37°C for 48 h. The optical density (OD) value was measured at 600 nm for identifying the minimal inhibitory concentration (MIC) of phloretin. The minimal concentrations that less than 80% growth of C. albicans occurred were recorded as MIC values [Citation19]. The growth inhibition rate was calculated as follow: [(OD600 (0 μg/mL phloretin) – OD600 (5–80 μg/mL phloretin))/OD600 (0 μg/mL phloretin)] * 100%
Time-kill assay
The time-kill assay was carried out according to the method described previously [Citation20]. C. albicans culture was diluted to 5 × 105 CFU/mL in RPMI-1640 medium supplemented with 165 mM MOPS. Phloretin was added to the C. albicans suspensions with a final concentration of 74.55, 149.10, or 298.20 μg/mL (equivalent to 1×, 2× or 4× MIC). The mixture was incubated at 37°C for 0 h, 0.1 h, 0.25 h, 0.5 h, 1 h, 3 h or 6 h. At each point of time, 10 μL suspension was removed and diluted 1:1000 with phosphate buffered saline (PBS). The suspension was streaked on YPD agar plates. After cultured for 48 h, the CFU was determined.
Calcofluor white staining
The effects of phloretin on C. albicans biofilm formation were evaluated by calcofluor white fluorescent staining [Citation21]. The mixture of C. albicans suspension (1 × 105 CFU/mL) was added into 96-well plates and incubated with 0, 0.5×, 1×, or 2× MIC phloretin for 24 h. Next, treated C. albicans culture was transferred to glass slides and stained with 1 g/L calcofluor white (50 μL; Sigma, St. Louis, MO, USA) for 1 min. The images were captured by microscopy BX53 microscope (Olympus, Tokyo, Japan).
Determination of membrane permeability
The membrane permeability of C. albicans biofilm was detected by employing the LIVE/DEAD ® BacLight™ Bacterial Viability and Counting Kit (Invitrogen, Carlsbad, California, USA) according to the users’ protocols. C. albicans with a compromised biofilm were considered dead, whereas C. albicans with an intact biofilm were regarded as live. 300 μL C. albicans suspension (1 × 105 CFU/mL) was added to 96-well plates and incubated with 0, 0.5×, 1×, or 2× MIC phloretin for 24 h. Then, C. albicans were washed with PBS and centrifuged at 10,000 g for 3 min. After resuspended with PBS, C. albicans were incubated with 1.5 μL SYTO-9 and 1.5 μL propidium iodide for 15 min preventing from the light. The proportion of live/dead cells were accomplished by NovoCyte flow cytometry (ACEA Biosciences, San Diego, California, USA). 20,000 C. albicans cells were counted for the detection. Fluorescence was detected by 503 nm (green; FL1 channel) and 605 nm (red; FL2 channel) bandpass filters. From PI versus SYTO-9 plot, population of dead and live cells were gated for the analysis. Red/green fluorescence double-stained cells were regarded as dead, and only green fluorescence-stained cells were considered live.
2,3-bis-(2-methoxy-4-nitro-5-sulfophenyl)-2 H-tetrazolium-5-carboxanilide (XTT) assay
The metabolic activity of C. albicans biofilm was evaluated by XTT assay as previous described [Citation22]. In brief, C. albicans with a compromised biofilm were considered dead, whereas C. albicans with an intact biofilm were regarded as live. 300 μL C. albicans suspensions (1 × 105 CFU/mL) were added to 96-well plates and incubated with 0, 0.5×, 1×, or 2× MIC phloretin for 24 h. Next, 20 μL XTT solution (Keygen Biotech, Nanjing, China) was added into 96-well plates per well and incubated at 37°C for 3 h. The OD value was measured at 450 nm using an ELX-800 microplate reader (BioTek Instruments, Winooski, VT, USA). The metabolic activity was calculated as follow: OD450 phloretin/OD450 control *100%.
Crystal-violet biofilm assay
300 μL C. albicans (1 × 105 CFU/mL) were added into 96-well plates and incubated with 0, 0.5×, 1×, or 2× MIC phloretin for 24 h. Biofilm cells that adhered to the bottom of the plates were stained with 0.1% crystal violet (Amreso, Shanghai, China) for 20 min and then were dissolved in 95% ethanol. The OD value was read at 570 nm by using NANO 2000 ultraviolet spectrophotometer (Thermo Scientific, Pittsburgh, PA, USA).
Hyphal inhibition assay
Hyphal formation assay of C. albicans was performed in RPMI 1640 medium supplemented with 10% fetal bovine serum. A mixture of C. albicans suspension (1 × 106 CFU/mL) was added into 96-well plates and incubated with 0, 0.5×, 1×, or 2× MIC phloretin for 24 h. The quantification of the effects of phloretin on the yeast-to-hyphal transition was determined by the proportion between the number of individual yeast cells and the number of hyphae in the population by a BX53 microscope. The hyphae inhibition rate (%) was calculated as follows: (the number of hyphaecontrol – the number of hyphaephloretin)/the number of hyphaecontrol×100%.
Quantitative real-time polymerase chain reaction (qRT-PCR)
C. albicans were respectively treated with 0.5× MIC phloretin, 1× MIC phloretin, or 2× MIC phloretin for 24 h prior total RNA extraction, reverse transcription, and PCR process. Total RNA was extracted from C. albicans cells by employing Plant RNA Rapid Extraction Kit (Bioteke, Wuxi, China) following the manufacturers’ instructions. cDNA was obtained by using Super M-MLV reverse transcriptase (Bioteke). The amplification was performed by using 2× Taq PCR MasterMix (Solarbio) and SYBR Green (Solarbio). The relative expression of genes’ mRNA was quantified with 2−ΔΔCT method [Citation23]. GTP-binding protein GSP1 was used as an internal control to normalize the qPCR result. The primers were synthesized by GenScript (Nanjing, China), and the sequences of primers were shown in .
Table 1. The sequences of primers used in qRT-PCR
Protease and phospholipase enzyme secretion assay
The protease and phospholipase enzyme secretion assays were conducted as previous performed [Citation24]. For the detection of protease activity, the supernatant of C. albicans biofilm was incubated with 1% azocasein (Shanghai Yuanye Bio-Technology, Shanghai, China) at a 1:9 (vol/vol) dilution at 37°C for 0 (A1) or 1 h (A2). Next, 500 μL of 10% trichloroacetic acid was added to the mixture and incubated for 10 min at room temperature. After centrifuged at 10,000 g for 5 min, 500 μL supernatant was incubated with 500 μL NaOH at 37°C for 15 min. The absorbance was measured at 440 nm [Citation25].
For the detection of phospholipase activity, the supernatant of C. albicans biofilm was incubated with the equal volume of 1.6 mM phosphatidylcholine substrate (MedChemExpress, Shanghai, China) at 37°C for 0 (A1) or 1 h (A2). The absorbance was measured at 630 nm [Citation25]. ΔA (increased absorbance after 1 h of reaction) = A2-A1. The activities of protease or phospholipase were shown as activity unit (U) that enhanced the absorbance by 0.001 per min and normalized by the dry weight of biofilm.
Mice model of oral candidiasis
The animal experiments were approved by the Medical Ethics Committee of Hospital of Stomatology, Hebei Medical University. And the experiments followed The Guideline for the Care and Use of Laboratory Animals. Six-week-old female BALB/c mice were purchased from Liaoning Changsheng biotechnology (Benxi, China) and were used for establishing oral candidiasis model after a week of acclimation. The establishment of mice model of oral candidiasis was conducted as previous performed [Citation26]. Mice oral cavities were sublingually infected with C. albicans by placing cotton ball saturated with 1 × 107 CFU/mL C. albicans suspension for 75 min. Mice were subcutaneously injected with 225 mg/kg cortisone acetate (Aladdin) for immunosuppression on the day before infection, the first and the third day after infection. Next, topical treatments of phloretin (3.73 or 7.46 mg/mL, which were equivalent to 50× or 100× MIC) were applied to the whole oral cavities twice a day starting on day one post-infection. Then, mice were sacrificed on the fifth day and the tongue tissues were dissected for subsequent experiments. After homogenization in 5 mL PBS, the dilutions of tongue tissues were seeded onto YPD ager plates. The colonies were enumerated at 10-fold dilution after 48 h. The results were shown as CFU per gram of tongue tissues.
The evaluation of the severity of C. albicans infection was measured by scoring lesions from zero to four based on the severity of whitish and curd-like patches on the surface of the tongue tissues [Citation27]. Zero: normal; One, white patches in less than 20%; two, white patches in 21%-90%; three, white patches in more than 91%; four, dense and thick white patches like pseudomembranes in more than 91%.
Periodic acid-schiff (PAS) staining
Dried paraffin sections (5 μm) of tongue tissues were soaked to xylene twice (15 min each time) and graded alcohols (100%, 95%, 85%, and 75%) for 5 min per grade. After soaked to distilled water for 2 min and PBS for 5 min, the sections were oxidized with periodic acid solution (Leagene Biotechnology, Beijing, China) for 10 min. Next, sections were stained with Schiff reagent (Leagene) for 15 min and re-stained with hematoxylin for 2 min. Sections were imaged by BX53 microscope after dehydration.
ELISA assay
The concentration of protein from the supernatant of tongue tissue homogenate was detected by using BCA Protein Assay Kit (Solarbio). The protein levels of tumor necrosis factor-α (TNF-α), interferon-α (IFN-γ), interleukin (IL)-6, IL-10 in tongue tissue supernatant were measured by employing ELISA kits (Multi Sciences, Hangzhou, China) according to the manufacturer’s protocols.
Statistical analysis
Data was shown as mean ± SD. One-way ANOVA or two-way ANOVA was applied to for multiple comparisons in GraphPad Prism 8.0 software. Three independent repeats were carried out for in vitro experiments, and six independent repeats were performed for in vivo experiments. p < 0.05 was considered statistical significance.
Results
In the current work, we aimed to explore the antifungal effects of phloretin against the C. albicans pathogenicity. We considered whether phloretin could abrogate virulence factors such as, biofilm formation, yeast-to-hyphae transition, and the secretion of hydrolases in vitro. Furthermore, a mice model of oral candidiasis was established to investigate the effectiveness of topical treatments of phloretin on the lesion severity and the inflammatory infiltration of tongue tissues in vivo.
The antifungal activity of phloretin on Candida albicans
To evaluate the antifungal activities of phloretin in vitro, we firstly identified the MIC of strain SC5314 growth. As shown in , the antifungal susceptibility test demonstrated that the MIC of phloretin against C. albicans was 74.55 μg/mL. Then, we conducted the time-killing assay by using a colony counting method. Phloretin killed C. albicans in a dose-dependent manner (). The CFU of C. albicans significantly decreased after an incubation with phloretin for an hour and dropped to an extremely low level when the incubation extended to six hours (). The data indicated that phloretin exerted antifungal effects on C. albicans growth.
Figure 1. The antifungal activity of phloretin on Candida albicans. (a) The minimal inhibitory concentration of phloretin that resulted in 80% suppression (MIC) of C. albicans growth. (b) Time-killing curves of C. albicans were measured by CFU counts in the presence of phloretin at 74.55, 149.10, or 298.20 μg/mL (the concentration equivalent to 1×, 2× or 4× MIC). *p < 0.05, **p < 0.01 versus Control
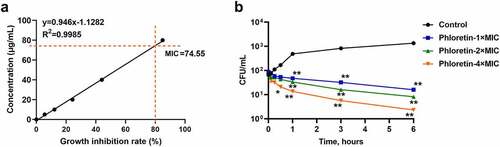
Phloretin inhibited the biofilm formation of Candida albicans
We further investigated the suppressed effects of phloretin on C. albicans biofilm formation. As revealed in , calcofluor white staining demonstrated that phloretin reduced the number of spores and hyphae stained in bright blue. Next, we examined the biofilm integrity of C. albicans by employing a live/dead staining assay. The results showed that the live/dead ratio of C. albicans exposed to phloretin treatment was reduced in a dose-dependent manner, implying that phloretin abrogated the membrane permeability of C. albicans biofilm (). Subsequently, the results of XTT assay revealed that the metabolic activity of C. albicans biofilm was mitigated by the phloretin treatment (). The reduced biofilm biomass of C. albicans caused by phloretin was also confirmed by crystal-violet biofilm assay (). These results suggested that phloretin alleviated the biofilm formation of C. albicans in a dose-dependent manner.
Figure 2. Phloretin inhibited the biofilm formation of Candida albicans. C. albicans (1 × 105 CFU/mL) was treated with 37.28, 74.55, or 149.10 μg/mL (the concentration equivalent to 0.5×, 1× or 2× MIC) phloretin. (a) Calcofluor white staining of C. albicans. Scale bar = 200 μm (b) The membrane permeability of C. albicans was measured by the proportion of live/dead cells. (c) The metabolic activity of C. albicans biofilm exposed to phloretin treatment was evaluated by XTT assay. (d) The biofilm biomass of C. albicans was assessed by crystal-violet biofilm assay. *p < 0.05, **p < 0.01 versus Control
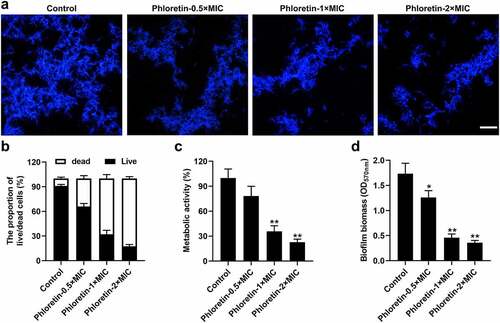
Phloretin suppressed the yeast-to-hyphae transition of Candida albicans
The effects of phloretin on the yeast-to-hyphae transition of C. albicans were explored in vitro. As shown in , phloretin significantly inhibited the hyphal formation, which was reduced by more than 70% when presented at concentration of 1× or 2× MIC. Moreover, the entire process of yeast-to-hyphae transition was strictly regulated at the molecular level. We found that the relative expression of hyphae-associated encoding genes including enhanced adherence to polystyrene 1 (EAP1), extent of cell elongation gene 1 (ECE1), hyphal wall protein 1 gene (HWP1), and agglutinin-like sequence gene 3 (ALS3) were decreased by phloretin in a dose-dependent manner (). The data implying that phloretin suppressed the yeast-to-hyphae transition of C. albicans.
Figure 3. Phloretin suppressed the yeast-to-hyphae transition of Candida albicans. C. albicans (1 × 106 CFU/mL) was treated with 37.28, 74.55, or 149.10 μg/mL (the concentration equivalent to 0.5×, 1× or 2× MIC) phloretin. (a) Representative images of the hyphal formation of C. albicans. Scale bar = 200 μm. The hyphae inhibition rate (%) = (the number of hyphaecontrol – the number of hyphaephloretin)/the number of hyphaecontrol×100%. (b-e) The relative expression of EAP1, ECE1, HWP1, and ALS3. *p < 0.05, **p < 0.01 versus Control
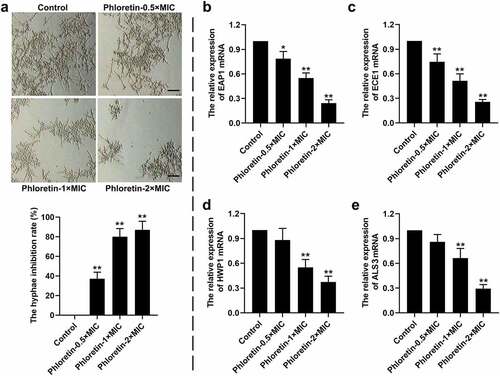
Phloretin attenuated the secretion of Candida albicans protease and phospholipase
We found that phloretin weakened the concentration of cellular protease and phospholipase ( and b). The expression of protease-encoding genes secreted aspartyl proteases (SAP)1 and SAP2, as well as phospholipase-encoding gene phospholipase B1 (PLB1) was reduced by phloretin in a dose-dependent manner (). These results proved that phloretin attenuated the virulence of C. albicans by repressing the protease and phospholipase activities.
Figure 4. Phloretin attenuated the secretion of Candida albicans protease and phospholipase. C. albicans (1 × 106 CFU/mL) was treated with 37.28, 74.55, or 149.10 μg/mL (the concentration equivalent to 0.5×, 1× or 2× MIC) phloretin. (a) The protease activity of C. albicans. (b) The phospholipase activity of C. albicans. (c-e) The mRNA level of SAP1, SAP2, and PLB1. *p < 0.05, **p < 0.01 versus Control
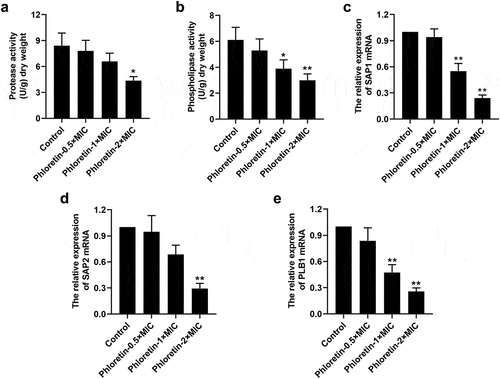
Phloretin ameliorated the fungal activity in the murine model of oral candidiasis
Given the observation of the antifungal activity of phloretin in vitro, we suspected that it might also play a role in the progression of oral candidiasis in vivo. To probe the possibility, we established a murine model of oral candidiasis. As revealed in and b, phloretin rescued the enhanced lesion severity and the increased CFU counts of C. albicans in tongue tissues of mice. Next, PAS and H&E staining were carried out to evaluate the histopathological changes. The results of PAS staining manifested that the increased number of C. albicans in the pathological tissues was decreased in the presence of phloretin in a dose-dependent manner (). H&E staining demonstrated that the inflammatory infiltration of tongue tissues of mice treated with phloretin was much less than that infected with C. albicans only (). Subsequently, an ELISA assay was employed to verify the secretion of inflammatory factors. The increased concentration of IL-6 and TNF-α was reversed by the phloretin treatment ( and f). The concentration of IL-10 and IFN-γ had no significant differences after the phloretin administration ( and h). Overall, we concluded that phloretin ameliorated the fungal activity in murine model of oral candidiasis.
Figure 5. Phloretin ameliorated the fungal activity in the murine model of oral candidiasis. Mice were sublingually infected with C. albicans suspension (1 × 107 CFU/mL) for 75 min. Topical treatments of phloretin (3.73 or 7.46 mg/mL, which were equivalent to 50× or 100× MIC) were applied twice a day starting on day one post-infection. (a) The score of lesions on the tongue tissues of mice for five days. (b) The CFU counts of C. albicans from the tongue tissues of mice with oral candidiasis. (c) The PAS staining of tongue tissues of mice. Arrows: C. albicans cells. Scale bar = 100 μm. (d) The H&E staining of tongue tissues of mice. Arrows: inflammatory cells. Scale bar = 100 μm. (e-h) The concentration of IL-6, TNF-α, IL-10, and IFN-γ. **p < 0.01 versus Sham; #p < 0.05, ##p < 0.01 versus Oral candidiasis
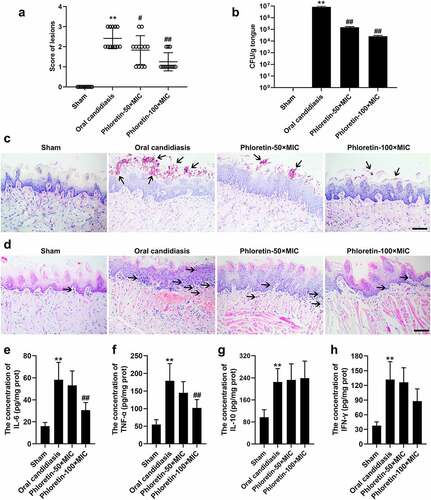
Discussion
In the current study, we found that phloretin inhibited the growth, biofilm formation, yeast-to-hyphae transition, and the hydrolase of C. albicans in vitro. The antifungal activity of phloretin was further confirmed by the in vivo experiments. Phloretin mitigated the lesion severity and the inflammatory infiltration of tongue tissues caused by C. albicans in murine model of oral candidiasis. The data suggested that phloretin might be an excellent candidate for the development of new antifungal drugs that block the oral candidiasis progression.
Natural compounds exert therapeutic functions against fungal infection owing to their easy bioavailability and antimicrobial activities [Citation9]. Phloretin is a dihydrochalcone flavonoid naturally found in apples and strawberries with broad bioactivities, such as anti-tumor, anti-inflammatory, antioxidant, and anti-bacterial effects [Citation28]. Wu et al. [Citation29] have verified that phloretin alleviates the progression of ulcerative colitis via regulating the gut microbiota. Cheon et al. [Citation11] have reported the anti-acne activity of phloretin ameliorates Propionibacterium acnes-induced skin infection. These studies indicate that phloretin plays a vital role in anti-bacterial infection. Moreover, phloretin also exhibits strong broad-range fungicidal activity. Gaucher et al. [Citation30] have proven that phloretin suppresses the growth of Alternaria brassicicola and Neurospora crassa in plants. Similarly, in this work, we found that phloretin exerted antifungal effects on C. albicans growth. The MIC of phloretin against C. albicans was 74.55 μg/mL, which was higher than that of miconazole (a drug clinically used in the treatment of oral candidiasis) against C. albicans [Citation31]. Furthermore, results involving positive drugs such as miconazole could better exhibit the inhibitory effects of phloretin against C. albicans, which is a limitation of this work.
C. albicans is the main etiological agent of oral candidiasis, which undermines the oropharynx and the esophagus of people with the dysregulation of adaptive immunity and even causes the life-threatening systemic fungal infection [Citation32]. A trait that causes a significant medical challenge of the infection is the ability of C. albicans to develop an organized consortia cell called biofilms, followed by the transition from yeast to hyphae [Citation33]. The fully mature biofilm has a mixture of yeast cells, hyphae, and pseudohyphae morphological forms in the extracellular matrix, indicating that the yeast-to-hyphae transition is directly associated with the improvement of biofilm formation [Citation34]. Flavonoids have been reported to inhibit the biofilm formation and hyphal development against C. albicans [Citation35,Citation36]. Similarly, in the present study, we found that phloretin repressed the membrane permeability, biofilm formation, and the yeast-to-hyphae transition in a dose-dependent manner.
Moreover, the entire process of yeast-to-hyphae transition was strictly regulated at the molecular level. ALS3, HWP1, and EAP1 are testified to contribute to biofilm formation and hyphal development via acting as complementary adhesins [Citation37]. Qiao et al. [Citation38] have explicated that the anti-virulence activity of terpenoids against C. albicans is elicited by hyphal formation repression upon reducing the expression of hyphae-associated encoding genes ALS3, HWP1, ECE1, UME6 and upregulating NRG1. In the current study, phloretin suppressed the yeast-to-hyphae transition of C. albicans by downregulating the expression of EAP1, ECE1, ALS3 and HWP1.
Following the adhesion to host cells and the development of hyphae, C. albicans hyphae promote active penetration into host cells by secreting hydrolase, resulting in the tissue degradation [Citation37]. Three types of hydrolase are secreted by C. albicans hyphae: proteases, phospholipases, and lipases [Citation37]. Eva Vaňková et al. [Citation39] have proven that the combination of lasioglossin and azoles abrogates C. albicans virulence by repressing biofilm formation, the secretion of phospholipases and proteases, as well as hemolytic activity. Abirami et al. [Citation40] have verified that the suppression of C. albicans pathogenicity is mediated by the inhibition of biofilm formation and the production of phospholipase and exopolymeric substances upon the downregulation of SAP1, SAP2, ALS3, ALS2, PLB1, and HWP1. In this work, similar results were obtained by the treatment of phloretin on C. albicans. We found that phloretin weakened the activities of cellular protease and phospholipase of C. albicans. The expression of protease-encoding genes SAP1 and SAP2 and the phospholipase-encoding gene PLB1 was reduced by phloretin in a dose-dependent manner.
Based on the antifungal activity of phloretin in vitro, we suspected that it might also act as an essential part of the progression of oral candidiasis in vivo. In the previous study, Seleem et al. [Citation24] have reported that Lichochalcone (a compound that belongs to flavonoids) exerts antifungal activity by reducing the C. albicans biofilm formation, the secretion of proteinases and phospholipases in vitro, as well as decreasing the CFU/mL/mg in tongue tissues of oral candidiasis mice in vivo. In another study, Andrade et al. [Citation41] have demonstrated that chalcone derivatives impair the hyphal transition of C. albicans in vitro and reduce the fungal load in vivo in the model of vulvovaginal candidiasis. In this work, our results showed that phloretin alleviated the lesion severity and the inflammatory infiltration of tongue tissues caused by C. albicans in the murine model of oral candidiasis. Furthermore, no tissue necrosis was observed in the phloretin-treated tongue tissues of mice, suggesting low toxicity to in vivo cells. Phloretin might provide an effective approach in C. albicans infection treatment of oral candidiasis.
Conclusion
Phloretin exerted antifungal activity by repressing the biofilm formation, yeast-to-hyphae transition, and the secretion of protease as well as phospholipase in vitro. The results were confirmed by the reversal of the enhanced lesion severity, inflammatory infiltration, and the increased CFU counts of C. albicans in tongue tissues of oral candidiasis mice in vivo.
Disclosure of potential conflicts of interest
No potential conflict of interest was reported by the author(s).
Author contribution
Na Liu and Nan Zhang performed the experiments and wrote the manuscript. Shengrong Zhang and Lifang Zhang assisted in the experiments and analyzed the data. Qing Liu reviewed and edited the manuscript. All authors approved the contents of the final manuscript.
Acknowledgements
The work was supported by Innovative experiment project for College Students of Hebei Medical University.
Data availability statement
The data that support the findings of this study are available from the corresponding author QL upon reasonable request.
References
- Lombardi A, Ouanounou A. Fungal infections in dentistry: clinical presentations, diagnosis, and treatment alternatives. Oral Surg Oral Med Oral Pathol Oral Radiol. 2020;130(5):533–546.
- Poulain D. Candida albicans, plasticity and pathogenesis. Crit Rev Microbiol. 2015;41(2):208–217.
- Lewis MAO, Williams DW. Diagnosis and management of oral candidosis. Br Dent J. 2017;223(9):675–681.
- Quindós G, Gil-Alonso S, Marcos-Arias C, et al. Therapeutic tools for oral candidiasis: current and new antifungal drugs. Med Oral Patol Oral Cir Bucal. 2019;24(2):e172–e80.
- Rodrigues CF, Rodrigues ME, Henriques MCR. Promising alternative therapeutics for oral candidiasis. Curr Med Chem. 2019;26(14):2515–2528.
- Millsop JW, Fazel N. Oral candidiasis. Clin Dermatol. 2016;34(4):487–494.
- Dadar M, Tiwari R, Karthik K, et al. Candida albicans - Biology, molecular characterization, pathogenicity, and advances in diagnosis and control - An update. Microb Pathog. 2018;117:128–138.
- Kadosh D. Regulatory mechanisms controlling morphology and pathogenesis in Candida albicans. Curr Opin Microbiol. 2019;52:27–34.
- Ansari MA, Fatima Z, Hameed S. Sesamol: a natural phenolic compound with promising anticandidal potential. J Pathog. 2014;2014:895193.
- Mendes RA, Silva BLS E, Takeara R, et al. Probing the antioxidant potential of phloretin and phlorizin through a computational investigation. J Mol Model. 2018;24(4):101.
- Cheon D, Kim J, Jeon D, et al. Target proteins of phloretin for its anti-inflammatory and antibacterial activities against -induced skin infection. Molecules. 2019;24(7):1319.
- Choi BY. Biochemical basis of anti-cancer-effects of phloretin-A natural dihydrochalcone. Molecules. 2019;24(2):278.
- Adil M, Baig MH, Rupasinghe HPV. Impact of citral and phloretin, alone and in combination, on major virulence traits of streptococcus pyogenes. Molecules. 2019;24(23):4237.
- Lopes LAA, dos Santos Rodrigues JB, Magnani M, et al. Inhibitory effects of flavonoids on biofilm formation by Staphylococcus aureus that overexpresses efflux protein genes. Microb Pathog. 2017;107:193–197.
- Raorane CJ, Lee J-H, Kim Y-G, et al. Antibiofilm and antivirulence efficacies of flavonoids and curcumin against acinetobacter baumannii. Front Microbiol. 2019;10:990.
- Lee J-H, Regmi SC, Kim J-A, et al. Apple flavonoid phloretin inhibits Escherichia coli O157: h7biofilm formation and ameliorates colon inflammation in rats. Infect Immun. 2011;79(12):4819–4827.
- Shim SH, Jo SJ, Kim JC, et al. Control efficacy of phloretin isolated from apple fruits against several plant diseases. Plant Pathology J. 2010;26(3):280–285.
- Clinical L Reference Method for Broth Dilution antifungal susceptibility testing of yeasts, 3rd edition, approved standard M27-A3. 2008.
- Chevalier M, Doglio A, Rajendran R, et al. Inhibition of adhesion-specific genes by Solidago virgaurea extract causes loss of Candida albicans biofilm integrity. J Appl Microbiol. 2019;127(1):68–77.
- Yu H, Liu X, Wang C, et al. Assessing the potential of four cathelicidins for the management of mouse candidiasis and Candida albicans biofilms. Biochimie. 2016;121:268–277.
- Wang B, You J, JB K, et al. Polyketide glycosides from Bionectria ochroleuca inhibit Candida albicans biofilm formation. J Nat Prod. 2014;77(10):2273–2279.
- Tan Y, Cheng Q, Yang H, et al. Effects of ALA-PDT on biofilm structure, virulence factor secretion, and QS in Pseudomonas aeruginosa. Photodiagnosis Photodyn Ther. 2018;24:88–94.
- Schmittgen TD, Livak KJ. Analyzing real-time PCR data by the comparative C(T) method. Nat Protoc. 2008;3(6):1101–1108.
- Seleem D, Benso B, Noguti J, et al. In vitro and in vivo antifungal activity of lichochalcone-A against candida albicans biofilms. PLoS ONE. 2016;11(6):e0157188.
- Santana IL, Gonçalves LM, de Vasconcellos AA, et al. Dietary carbohydrates modulate Candida albicans biofilm development on the denture surface. PLoS ONE. 2013;8(5):e64645.
- Pierce CG, Chaturvedi AK, Lazzell AL, et al. A novel small molecule inhibitor of Candida albicans biofilm formation, filamentation and virulence with low potential for the development of resistance. NPJ Biofilms Microbiomes. 2015;1(1):15012.
- Takakura N, Sato Y, Ishibashi H, et al. A novel murine model of oral candidiasis with local symptoms characteristic of oral thrush. Microbiol Immunol. 2003;47(5):321–326.
- Geohagen BC, Korsharskyy B, Vydyanatha A, et al. Phloretin cytoprotection and toxicity. Chem Biol Interact. 2018;296:117–123.
- Wu M, Li P, An Y, et al. Phloretin ameliorates dextran sulfate sodium-induced ulcerative colitis in mice by regulating the gut microbiota. Pharmacol Res. 2019;150:104489.
- Gaucher M, Dugé De Bernonville T, Lohou D, et al. Histolocalization and physico-chemical characterization of dihydrochalcones: insight into the role of apple major flavonoids. Phytochemistry. 2013;90:78–89.
- Vandenbosch D, Braeckmans K, Nelis HJ, et al. Fungicidal activity of miconazole against Candida spp. biofilms. J Antimicrob Chemother. 2010;65(4):694–700.
- Wall G, Montelongo-Jauregui D, Vidal Bonifacio B, et al. Candida albicans biofilm growth and dispersal: contributions to pathogenesis. Curr Opin Microbiol. 2019;52:1–6.
- Tsui C, Kong EF, Jabra-Rizk MA. Pathogenesis of Candida albicans biofilm. Pathog Dis. 2016;74(4):ftw018.
- Manoharan RK, Lee J-H, Kim Y-G, et al. Alizarin and chrysazin inhibit biofilm and hyphal formation by Candida albicans. Front Cell Infect Microbiol. 2017;7:447.
- Smiljković M, Kostić M, Stojković D, et al. Could flavonoids compete with synthetic azoles in diminishing Candida albicans infections? A comparative review based on in vitro studies. Curr Med Chem. 2019;26(14):2536–2554.
- Peralta MA, Ortega MG, Cabrera JL, et al. The antioxidant activity of a prenyl flavonoid alters its antifungal toxicity on Candida albicans biofilms. Food Chem Toxicol. 2018;114:285–291.
- Mayer FL, Wilson D, Hube B. Candida albicans pathogenicity mechanisms. Virulence. 2013;4(2):119–128.
- Qiao Y-N, Jin X-Y, Zhou J-C, et al. Terpenoids from the liverwort plagiochila fruticosa and their antivirulence activity against Candida albicans. J Nat Prod. 2020;83(6):1766–1777.
- Vaňková E, Kašparová P, Dulíčková N, et al. Combined effect of lasioglossin LL-III derivative with azoles against Candida albicans virulence factors: biofilm formation, phospholipases, proteases and hemolytic activity. FEMS Yeast Res. 2020;20(3):foaa020.
- Abirami G, Alexpandi R, Durgadevi R, et al. Inhibitory effect of morin against candida albicans pathogenicity and virulence factor production: an in vitro and in vivo approaches. Front Microbiol. 2020;11:561298.
- Andrade JT, Santos FRS, Lima WG, et al. Design, synthesis, biological activity and structure-activity relationship studies of chalcone derivatives as potential anti-Candida agents. J Antibiot (Tokyo). 2018;71(8):702–712.