ABSTRACT
Ulcerative colitis (UC) is an intestinal inflammatory disorder. Long non-coding RNAs (lncRNAs) are collectively involved in UC. This study is designed to explore the roles of lncRNA (small nucleolar RNA host gene 5) SNHG5 in UC. Gene or microRNA (miRNA) expression was detected using RT-qPCR and western blot, respectively. Cellular functions were analyzed by cell counting kit 8 (CCK8), 5-ethynyl-2′-deoxyuridine (EdU) assay, flow cytometry, and the terminal deoxyribonucleotidyl transferase (TDT)‐mediated dUTP‐digoxigenin nick end labeling (TUNEL) assays. Lactate dehydrogenase (LDH) content was determined by a cell cytotoxicity assay. The interactions between miR-375 and SNHG5 or Janus kinase-2 (JAK2) were verified by a luciferase reporter assay. SNHG5 was up-regulated in intestinal mucosa tissues of UC patients as well as tumor necrosis factor alpha-treated (TNF-α-treated) young adult mouse colon (YAMC) cells. Down-regulated SNHG5 promoted cell proliferation and inhibited apoptosis of YAMC cells. miR-375 was verified to be a target of SNHG5 and was suppressed by TNF-α treatment in YAMC cells. Over-expression of miR-375 restored YAMC cellular functions. Additionally, miR-375 targeted JAK2, which was up-regulated by TNF-α treated YAMC cells. Up-regulation of JAK2 induced the dysfunction of YAMC cells. Knockdown of SNHG5 promoted the proliferation and suppressed the apoptosis of YAMC cells via regulating miR-375/JAK2 axis. Therefore, knockdown of SNHG5 may be a promising therapy for UC.
Introduction
Ulcerative colitis (UC) is an intestinal inflammatory disorder, which occurs most often in adults in their 30s [Citation1,Citation2]. Varieties of drugs for individualized treatment plus regular surveillance colonoscopies are the recognized regular therapeutic treatments for UC patients [Citation3]. Various factors complicated the pathogenesis of UC. Inflammatory response in intestinal epithelial cells promotes the pathogenesis of UC [Citation4,Citation5]. Tumor necrosis factor alpha (TNF-α) collectively participates in inflammatory bowel disease [Citation6]. What’s more, anti-TNF-α drugs have been widely used to treat UC in clinic to minimize steroid exposure and dependence in recent years [Citation7,Citation8]. Surgical rates for UC, however, are not decreased under the application of anti-TNF-α drugs [Citation7]. Thus, it is urgent to study the potential molecular mechanism of TNF-α on UC.
Long non-coding RNAs (lncRNAs), without protein-encoding capability, function as the competing endogenous RNAs (ceRNAs) by sponging microRNAs (miRNAs) to regulate the progression of various diseases [Citation9,Citation10]. Recently, numerous lncRNAs are associated with the onset and development of UC, such as lncRNA MALAT1 [Citation11], lncRNA CDKN2B-AS1 [Citation12], and lncRNA TUG1 [Citation13]. lncRNAs may have the potential as biomarkers and therapeutic targets. lncRNA SNHG5, located on chromosome 6q14.3, was reported to regulate progression of colorectal cancer [Citation14–16], nasopharyngeal carcinoma [Citation17], ovarian cancer [Citation18], breast cancer [Citation19], glioma [Citation20], and COPD [Citation21], etc. What’s more, SNHG5 was down-regulated in colitis samples according to bioinformatic analysis [Citation22]. Despite of the above literature, the mechanisms of SNHG5 have not previously been elucidated in UC.
miRNAs are considered to take part in various diseases via targeting the downstream genes [Citation23]. Dysregulated miR-375 contributes to the progression of oral squamous cell carcinoma [Citation24], prostate cancer [Citation25], CRC [Citation26], and osteosarcoma [Citation27]. Furthermore, miR-375 was down-regulated in UC [Citation28,Citation29]. In this study, the potential roles of SNHG5 and miR-375 in UC were investigated.
Our study aimed to investigate the expression as well as molecular mechanism of SNHG5 in the development of UC. Furthermore, we hypothesized that SNHG5 sponges miR-375 to regulate JAK2 expression to regulate proliferation and apoptosis of UC cell. Our research provided a novel understanding of UC treatment.
Materials and methods
Clinical samples
A total of 30 patients with UC diagnosed at Affiliated Hospital of Integrated Traditional Chinese and Western Medicine, Nanjing University of Chinese Medicine, from January 1, 2019, to May 1, 2020, were obtained. Thirty healthy volunteers who underwent colonoscopy in the same period were selected as controls. Intestinal mucosa tissues were taken from sigmoid colons. Clinical samples were rapidly stored at in liquid nitrogen at −80°C. This study was authorized by the Ethics Committee of Affiliated Hospital of Integrated Traditional Chinese and Western Medicine, Nanjing University of Chinese Medicine. All the patients had provided the informed consent.
Cell culture and transfection
The conditionally immortalized epithelial cell line of young adult mouse colon (YAMC) cell was purchased from Institute of Hematology, Chinese Academy of Medical Sciences (Tianjin, China) and cultured in complete Dulbecco’s modified Eagle medium (DMEM) supplemented with 10% fetal bovine serum (FBS) (Gibco, Calif, USA) and 1% penicillin/streptomycin (Thermo Fisher, Calif, USA). YAMC cells were exposed to 10 ng/ml TNF-α (10 μG; H8916-10UG; Sigma-Aldrich, NJ, USA) for 12 h to establish a model of UC in vitro [Citation13].
si-SNHG5 1#, si-SNHG5 2#, miR-375 inhibitor, NC inhibitor, miR-375 mimic, JAK2, and the negative control or empty vector were transferred into cells using LipofectaminTM 3000 (Life Technologies, Calif, USA).
Real-time quantitative reverse transcription-polymerase chain reaction (qRT-PCR)
qRT-PCR was used to evaluate mRNA and miRNA levels [Citation30]. Total RNA of YAMC cells extracted by TRIzol® reagent (Thermo Fisher Scientific, Calif, USA). Reverse transcription and qPCR were carried out using BlazeTaq One-Step SYBR Green RT-qPCR Kit (with ROX) (QP071; GeneCopoeia, MD, USA) on SEDI Thermo Cycler controlled by the Control Bus Net software package (Wealtec Bioscience, Taiwan, China). GAPDH and U6 served as internal reference for mRNA and miRNA, respectively. The results were calculated using 2−ΔΔCt method. The primers used in PCR were obtained from Nanjing Genscript Biotech Co., Ltd (Jiangsu, China). The primer sequences were listed in .
Table 1. The sequences for primers used in this study
Cell counting kit 8 (CCK8)
Cell viability was determined by a CCK8 assay [Citation31]. YAMC cells were plated in a 96-well plate (1 × 104 cells/well). 10 μl of CCK8 reagents (AMJ-KT0001; AmyJet Technology, Beijing, China) were added to each well of the plate. Then, cells were cultured in the incubator. The absorbance values were evaluated with a microplate reader (HBS-1096 C; Nanjing DeTie Experimental Equipment, Jiangsu, China) at the wavelength of 450 nm.
5-ethynyl-2′-deoxyuridine (EdU) assay
The EdU assay was performed to determine cell proliferation [Citation32]. 2 × 105 YAMC cells were seeded into a 24-well plate with 200 μL of diluted EdU (Beyotime, Jiangsu, China). Then, cells were washed with PBS twice, 200 μL of Hoechst was applied, and were cultured in the dark for 30 min. Finally, EdU-labeled and Hoechst stained cells were captured.
Cell cytotoxicity assay
The levels of lactate dehydrogenase (LDH) in YAMC cell were detected with a LDH Assay Kit (Abcam, Calif, USA) to evaluate cell cytotoxicity [Citation33].
Flow cytometry assay
The apoptosis of logarithmic phase YAMC cells was detected by a Annexin V-FITC/PI kit (Yeason Biotech, Shanghai, China) [Citation34]. The supernatant was discarded after YAMC cells were centrifuged. Cells were stained with Annexin V-FITC and PI. Finally, the apoptosis rates were detected by a flow cytometry.
Terminal deoxyribonucleotidyl transferase (TDT)‐mediated dUTP‐digoxigenin nick end-labeling (TUNEL) assay
Cell death of YAMC was determined using a TUNEL kit (KGA702-1, KeyGEN BioTECH, Jiangsu, China) [Citation35]. Briefly, cells were deparaffinized and then cultured with TUNEL. Then, cells were counterstained with DAPI. Images were acquired on a Nikon microscope (90i, Tokyo, Japan).
Western blotting assay
Apoptosis-related proteins including apoptosis inhibitor 5 (API5), caspase-3, and BAX were collected from YAMC cells to evaluate cell apoptosis [Citation36]. Protein concentrations were determined with the BCA kit (Sigma-Aldrich, NJ, USA). Additionally, proteins were isolated by 12% SDS-PAGE gel. The isolated protein was moved onto PVDF membranes (Bio-rad, Calif, USA), which were blocked with 5% nonfat milk. Then, the membranes were incubated with primary antibodies: anti-API5 (70 R-32,287, 1:3000, Fitzgerald, Birmingham, UK), anti-caspase-3 (ab32351, 1:5000, Abcam, Calif, USA), anti-BAX (ab32503, 1:1000, Abcam, Calif, USA), and anti-GAPDH (ab8245, 1:500, Abcam, Calif, USA); and then with secondary antibodies. Finally, protein expressions were captured using an ECL kit.
Luciferase reporter assay
The interactions between miR-375 and SNHG5 or JAK2 were verified by a luciferase reporter assay [Citation37]. Full-length 3ʹUTR of SNHG5 and JAK2 inserted into pMIR-GLOTM luciferase vectors. Afterward, cells were co-transfected with luciferase vectors and empty vector and miR-375 mimic or nc mimic using Lipofectamine® 3000 (Life Technologies, Calif, USA). After 48 h, the results were detected using a Dual-Luciferase Reporter Assay kit (K801-200; BioVision Tech, San Francisco, USA).
Statistical analysis
Graphpad 6.0 (GraphPad Software, Calif, USA) was used for statistical analysis. Data were presented as ± SD. Student’s t-test (two groups) and one-way ANOVO (multiple groups) were applied for difference analysis. P < 0.05 was considered statistically significant.
Results
Over-expressed SNHG5 was up-regulated in UC
SNHG5 expression level was significantly increased in intestinal mucosa tissues of UC patients compared with normal group (). As shown in , TNF-α-treated YAMC cells significantly up-regulated expression of SNHG5 compared control group.
Knockdown of SNHG5 modulated cellular functions of YAMC cells
As shown in , the expression of SNHG5 was decreased after si-SNHG5 plasmids were transferred into YAMC cells, which was more remarkable in si-SNHG5 1# group. TNF-α suppressed cell viability () and proliferation () while inhibition of SNHG5 reversed the effects of TNF-α on YAMC cells. Simultaneously, the increase of LDH release induced by TNF-α was significantly suppressed by SHNG5 knockdown (). Furthermore, TNF-α significantly promoted apoptosis while si-SNHG5 neutralized the effects of TNF-α on YAMC cells (). SNHG5 knockdown increased the protein expression of API5 and decreased CASP3 and BAX ().
Figure 2. Effects of SNHG5 on YAMC cells. (a) SNHG5 expression levels were detected. (b) Cell viability was detected by CCK8. (c) EdU staining assay was used to determine cell proliferation. (d) Cell injury was examined with the LDH assay. (e) Flow cytometry assay was used to detect apoptosis. (f) TUNEL staining was used to detect cell death. (g) The protein expression of API5, CASP3, and BAX. ***p < 0.001, vs. (negative control) NC group or control group. ##p < 0.01, vs. TNF-α group
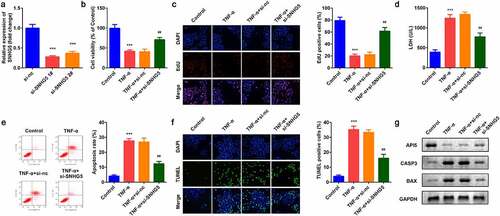
SNHG5 targeted miR-375 in YAMC cells
The online database Starbase 3.0 predicted that miR-375 was a target of SNHG5 (). Luciferase assay further verified the interaction between miR-375 and SNHG5 (). Furthermore, miR-375 expression was remarkably overexpressed by si-SNHG5 in YAMC cells. Moreover, TNF-α exposure notably decreased the expression of miR-375 ().
Down-regulation of miR-375 reversed the effects of SNHG5 on cell proliferation and apoptosis
The expression of miR-375 was remarkably downregulated by miR-375 inhibitor group, suggesting cells were successfully transfected (). Decreased miR-375 notably suppressed cell viability () and cell proliferation () and enhanced cell apoptosis (). Moreover, Down-regulation of miR-375 reversed the regulatory roles of si-SNHG5 in the release of LDH (). Besides, miR-375 alleviated the effects of SNHG5 knockdown on the expression of API5, CASP3, and BAX in protein level ().
Figure 4. Effects of miR-375 on YAMC cells. (a) miR-375 expression levels were detected. (b) Cell viability was detected by CCK8. (c) EdU staining assay was used to determine cell proliferation. (d) Cell injury was examined with the LDH assay. (e) Flow cytometry assay was used to detect apoptosis. (f) TUNEL staining was used to detect cell death. (g) The protein expression of API5, CASP3, and BAX. ***p < 0.001, vs. NC group or control group. #p < 0.05, ##p < 0.01, vs. TNF-α group
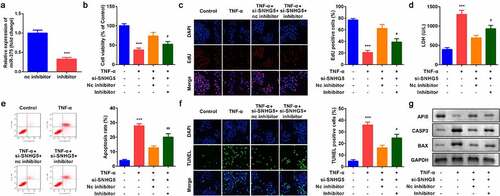
miR-375 directly targeted JAK2
TargetScan 7.2 predicted the binding sites between JAK2 and miR-375 (). Luciferase assay further confirmed the interaction between JAK2 and miR-375 (). The mRNA expression of JAK2 was significantly increased by miR-375 inhibitor in YAMC cells. Additionally, JAK2 was remarkably up-regulated in TNF-α-treated YAMC cells ().
Up-regulation of JAK2 inhibited the effects of miR-375
As shown in , JAK2 expression was significantly increased after JAK2 overexpression plasmids were transfeted into YAMC cells. Compared with miR-375 mimic group, JAK2 apparently inhibited cell viability () and cell proliferation () and accelerated apoptosis (). Moreover, up-regulation of JAK2 reversed the regulatory roles of miR-375 mimic in the release of LDH (). Besides, JAK2 alleviated the effects of miR-375 mimic on the expression of API5, CASP3, and BAX in protein level ().
Figure 6. Effects of JAK2 on YAMC cells. (a) JAK2 expression levels were detected. (b) Cell viability was detected by CCK8. (c) EdU staining assay was used to determine cell proliferation. (d) Cell injury was examined with the LDH assay. (e) Flow cytometry assay was used to detect apoptosis. (f) TUNEL staining was used to detect cell death. (g) The protein expression of API5, CASP3, and BAX. ***p < 0.001, vs. control group. ##p < 0.01, ###p < 0.001, vs. TNF-α group. &p < 0.05, &&p < 0.01, vs. TNF-α + miR-375 mimic group
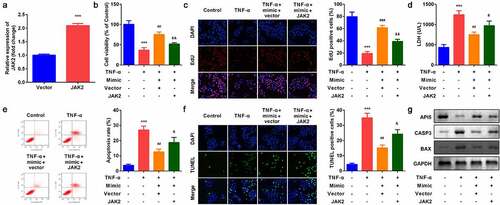
Discussion
This study identified the potential roles of lncRNA SNHG5 in UC. We unveiled the underlying mechanisms that SNHG5 promoted the progression of UC via regulating miR-375/JAK2 axis. SNHG5 was overexpressed in UC. However, knockdown of SNHG5 restored human intestinal epithelial cellular function, the dysfunction of which is one of the key factors for UC. Additionally, SNHG5 sponged miR-375 to promote the upregulation of JAK2, which is the key regulator of cytokine and growth factor signaling. Together, SNHG5 overexpression was associated with the development of UC. Knockdown of SNHG5 inhibited the progression of UC via modulating miR-375/JAK2 axis.
Augmenting evidence reveal that non-coding RNAs are involved in the progression of UC. Long non-coding RNAs are families of RNAs with over 200 nucleotides. Abnormal expression of lncRNAs may function as a promoter or suppressor of UC [Citation11–13]. Dysregulation of SNHG5 plays a crucial role in intestinal diseases. SNHG5 is reported to function as an oncogene in CRC [Citation14]. Moreover, Wang K et al. revealed that SNHG5 was down-regulated in colitis in vivo [Citation22]. In this study, SNHG5 was dramatically up-regulated in intestinal mucosa tissues of UC patients and in TNF-α-treated YAMC cells, indicating that SNHG5 may be closely associated with the development of UC. Further study showed that knockdown of SNHG5 restored the function of human intestinal epithelial cells, manifested by enhanced cell proliferation and decreased apoptosis rates. Furthermore, lncRNAs function as ceRNA and modulate cellular functions via sponging miRNAs. For instance, lncRNA CDKN2B-AS1 suppressed inflammatory response in UC through binding to miR-16 and miR-195 [Citation12] Overexpression of TUG1 promoted the proliferation and suppressed the apoptosis of mice intestinal epithelial cell [Citation13].
Therefore, we further identified the target miRNAs of SNHG5. miR-375 was predicted and proved to be a target miRNA of SNHG5. miR-375 was evidenced to be down-regulated in UC [Citation28,Citation29]. It is down-regulation activated inflammatory signaling in UC [Citation28]. Therefore, miR-375 may play a protective role in UC. In this study, miR-375 was decreased in UC. Decreased miR-375 antagonized the effects of SNHG5 and induced dysfunction of human intestinal epithelial cells. These results suggested that SNHG5 may participate in the progression of UC via sponging miR-375.
JAK2 is an ubiquitously expressed protein involved in the inflammatory response, and is critical in the pathogenesis and progression of diseases [Citation38,Citation39]. TNF-α induced apoptosis was suppressed by inhibiting JAK2 in osteoblasts [Citation40]. JAK2-STAT3 inhibitor effectively inhibited TNF-α raised by homocysteine treatment in microglia [Citation41]. Furthermore, intestinal mucosal injury usually leads to the increase of cytokines including TNF-α and is related to the abnormal expression of JAK2 protein [Citation42]. In this study, JAK2 was a target of miR-375 and was up-regulated in UC, which is consistent with previous studies [Citation28,Citation29]. Its upregulation alleviated the effects of miR-375, and suppressed the proliferation and inhibited the apoptosis of YAMC cells.
However, this study has some limitations. For instance, only 30 samples of UC were obtained in this study. Therefore, we need to collect more samples to make our conclusions more accurate and reliable. Further studies are needed to focus on the regulation of SNGH5 in UC at different stages. Moreover, down-regulation of SHNG5 did not totally abolish the TNF-α-induced cell dysfunction compared with untreated cells, which may indicate that there are multiple mechanisms in UC that co-regulate to alleviate TNF-α induced dysfunction. Moreover, the effective in vivo experiments are needed to support the results.
Conclusion
Our research suggested SNHG5 acted as a ceRNA to regulate the proliferation and apoptosis of YAMC cells via miR-375/JAK2 axis. Knockdown of SNHG5 protected against TNF-α reduced dysfunction of human intestinal epithelial cells in UC. Hence, SNHG5/miR-375/JAK2 axis can be an alternative for the treatment of UC.
Research Highlights
lncRNA SNHG5 was upregulated in the intestinal mucosa tissues of UC patients.
Knockdown of lncRNA SNHG5 promoted proliferation and inhibited apoptosis of HT-29 cells.
lncRNA SNHG5 regulated the development of UC via miR-375/JAK2 axis.
Ethics approval and consent to participate
The experimental protocol was established, according to the ethical guidelines of the Helsinki Declaration and was approved by the Ethics Committee of Affiliated Hospital of Integrated Traditional Chinese and Western Medicine, Nanjing University of Chinese Medicine. Written informed consent was obtained from individual or guardian participants.
Acknowledgements
Not applicable
Disclosure statement
No potential conflict of interest was reported by the author(s).
Additional information
Funding
References
- Ungaro R, Mehandru S, Allen PB, et al. Ulcerative colitis. Lancet. 2017;389(10080):1756–1770.
- Olen O, Erichsen R, Sachs MC, et al. Colorectal cancer in ulcerative colitis: a Scandinavian population-based cohort study. Lancet. 2020;395(10218):123–131.
- Kucharzik T, Koletzko S, Kannengiesser K, et al. Ulcerative colitis-diagnostic and therapeutic algorithms. Dtsch Arztebl Int. 2020;117:564–574.
- Keshteli AH, Madsen KL, Dieleman LA. Diet in the pathogenesis and management of ulcerative colitis; a review of randomized controlled dietary interventions. Nutrients. 2019;11(7):1498.
- Han J, Li Y, Zhang B, et al. lncRNA TUG1 regulates ulcerative colitis through miR-142-5p/SOCS1 axis. Microb Pathog. 2020;143:104139.
- Glick LR, Cifu AS, Feld L. Ulcerative Colitis in Adults. JAMA. 2020;324(12):1205–1206.
- Pugliese D, Felice C, Papa A, et al. Anti TNF-alpha therapy for ulcerative colitis: current status and prospects for the future. Expert Rev Clin Immunol. 2017;13(3):223–233.
- Raine T, Verstockt B, De Cruz P. Immune therapies in ulcerative colitis: are we beyond anti-TNF yet? Lancet Gastroenterol Hepatol. 2020;5(9):794–796.
- Dieter C, Lourenco ED, Lemos NE. Association of long non-coding RNA and leukemia: a systematic review. Gene. 2020;735:144405.
- Pouyanrad S, Rahgozar S, Ghodousi ES. Dysregulation of miR-335-3p, targeted by NEAT1 and MALAT1 long non-coding RNAs, is associated with poor prognosis in childhood acute lymphoblastic leukemia. Gene. 2019;692:35–43.
- Zhu M, Xie J. LncRNA MALAT1 promotes ulcerative colitis by upregulating lncRNA ANRIL. Dig Dis Sci. 2020;65(11):3191–3196.
- Tian Y, Cui L, Lin C, et al. LncRNA CDKN2B-AS1 relieved inflammation of ulcerative colitis via sponging miR-16 and miR-195. Int Immunopharmacol. 2020;88:106970.
- Tian Y, Wang Y, Li F, et al. LncRNA TUG1 regulates the balance of HuR and miR-29b-3p and inhibits intestinal epithelial cell apoptosis in a mouse model of ulcerative colitis. Hum Cell. 2021;34(1):37–48.
- Damas ND, Marcatti M, Côme C, et al. SNHG5 promotes colorectal cancer cell survival by counteracting STAU1-mediated mRNA destabilization. Nat Commun. 2016;7(1):13875.
- Zhang M, Li Y, Wang H, et al. LncRNA SNHG5 affects cell proliferation, metastasis and migration of colorectal cancer through regulating miR-132-3p/ CREB5. Cancer Biol Ther. 2019;20(4):524–536.
- Li Y-H, Hu Y-Q, Wang S-C, et al. LncRNA SNHG5: a new budding star in human cancers. Gene. 2020;749:144724.
- Liu D, Wang Y, Zhao Y, et al. LncRNA SNHG5 promotes nasopharyngeal carcinoma progression by regulating miR-1179/HMGB3 axis. BMC Cancer. 2020;20(1):178.
- Lin H, Shen L, Lin Q, et al. SNHG5 enhances Paclitaxel sensitivity of ovarian cancer cells through sponging miR-23a. Biomed Pharmacother. 2020;123:109711.
- Chi J-R, Yu Z-H, Liu B-W, et al. SNHG5 promotes breast cancer proliferation by sponging the miR-154-5p/PCNA axis. Mol Ther Nucleic Acids. 2019;17:138–149.
- Meng X, Deng Y, Lv Z, et al. LncRNA SNHG5 promotes proliferation of glioma by regulating miR-205-5p/ZEB2 axis. Onco Targets Ther. 2019;12:11487–11496.
- Shen Q, Zheng J, Wang X, et al. LncRNA SNHG5 regulates cell apoptosis and inflammation by miR-132/PTEN axis in COPD. Biomed Pharmacother. 2020;126:110016.
- Wang K, Zhang Z, Liu K, et al. Neat1-miRNA204-5p-PI3K-AKT axis as a potential mechanism for photodynamic therapy treated colitis in mice. Photodiagnosis Photodyn Ther. 2018;24:349–357.
- Zhang B, Chen J, Cui M, et al. LncRNA ZFAS1/miR-1271-5p/HK2 promotes glioma development through regulating proliferation, migration, invasion and apoptosis. Neurochem Res. 2020;45(12):2828–2839.
- Wu Y, Sun X, Song B, et al. MiR-375/SLC7A11 axis regulates oral squamous cell carcinoma proliferation and invasion. Cancer Med. 2017;6(7):1686–1697.
- Wang Y, Lieberman R, Pan J, et al. miR-375 induces docetaxel resistance in prostate cancer by targeting SEC23A and YAP1. Mol Cancer. 2016;15(1):70.
- Chen X, Xu M, Xu X, et al. METTL14 suppresses CRC progression via regulating N6-methyladenosine-dependent primary miR-375 processing. Mol Ther. 2020;28(2):599–612.
- Liu G, Huang K, Jie Z, et al. CircFAT1 sponges miR-375 to promote the expression of Yes-associated protein 1 in osteosarcoma cells. Mol Cancer. 2018;17(1):170.
- Wu CP, Bi Y-J, Liu D-M, et al. Hsa-miR-375 promotes the progression of inflammatory bowel disease by upregulating TLR4. Eur Rev Med Pharmacol Sci. 2019;23(17):7543–7549.
- Jabandziev P, Kakisaka T, Bohosova J, et al. MicroRNAs in colon tissue of pediatric ulcerative pancolitis patients allow detection and prognostic stratification. J Clin Med. 2021;10(6):6.
- Pu Z, Ge F, Wang Y, et al. Ginsenoside-Rg3 inhibits the proliferation and invasion of hepatoma carcinoma cells via regulating long non-coding RNA HOX antisense intergenic. Bioengineered. 2021;12(1):2398–2409.
- Tang Y, Li Y, Xin D, et al. Icariin alleviates osteoarthritis by regulating autophagy of chondrocytes by mediating PI3K/AKT/mTOR signaling. Bioengineered. 2021;12(1):2984–2999.
- Tang C, Feng W, Bao Y, et al. Long non-coding RNA TINCR promotes hepatocellular carcinoma proliferation and invasion via STAT3 signaling by direct interacting with T-cell protein tyrosine phosphatase (TCPTP). Bioengineered. 2021;12(1):2119–2131.
- Chen M-Y, Fan K, Zhao L-J, et al. Long non-coding RNA nuclear enriched abundant transcript 1 (NEAT1) sponges microRNA-124-3p to up-regulate phosphodiesterase 4B (PDE4B) to accelerate the progression of Parkinson’s disease. Bioengineered. 2021;12(1):708–719.
- Wang J, Lv P. Chrysophanol inhibits the osteoglycin/mTOR and activates NF2 signaling pathways to reduce viability and proliferation of malignant meningioma cells. Bioengineered. 2021;12(1):755–762.
- Shi J, Fu C, Su X, et al. Ultrasound-stimulated microbubbles inhibit aggressive phenotypes and promotes radiosensitivity of esophageal squamous cell carcinoma. Bioengineered. 2021;12(1):3000–3013.
- Mao HY, Liu LN, Hu YM. Mesenchymal stem cells-derived exosomal miRNA-28-3p promotes apoptosis of pulmonary endothelial cells in pulmonary embolism. Eur Rev Med Pharmacol Sci. 2020;24(20):10619–10631.
- Zhou Q, Zhang L. MicroRNA-183-5p protects human derived cell line SH-SY5Y cells from mepivacaine-induced injury. Bioengineered. 2021;12(1):3177–3187.
- Hu H, Wang S, Shi D, et al. Lycorine exerts antitumor activity against osteosarcoma cells in vitro and in vivo xenograft model through the JAK2/STAT3 pathway. Onco Targets Ther. 2019;12:5377–5388.
- Fu Y, Xu Y, Chen S, et al. MiR-151a-3p promotes postmenopausal osteoporosis by targeting SOCS5 and activating JAK2/STAT3 signaling. Rejuvenation Res. 2020;23(4):313–323.
- Ni Y, Zhang H, Zhang J, et al. Inhibition of JAK2 by AG490 promotes TNF-alpha-induced apoptosis by inhibiting autophagy in MC3T3-E1 cells. Die Pharmazie. 2020;75(6):255–260.
- Chen S, Dong Z, Cheng M, et al. Homocysteine exaggerates microglia activation and neuroinflammation through microglia localized STAT3 overactivation following ischemic stroke. J Neuroinflammation. 2017;14(1):187.
- Lee SY, Lee SH, Yang E-J, et al. Coenzyme Q10 inhibits Th17 and STAT3 signaling pathways to ameliorate colitis in mice. J Med Food. 2017;20(9):821–829.