ABSTRACT
Gut microbiota are the candidate biomarkers for neurogenic bowel dysfunction (NBD) in patients with spinal cord injury (SCI). We aimed to identify the common features between patients with varying degree of thoracic SCI and healthy individuals and subpopulations of microbiota correlated with the serum biomarkers. Twenty-one patients with complete thoracic SCI (CTSCI), 24 with incomplete thoracic SCI (ITSCI), and 24 healthy individuals (HC) were enrolled in this study. Fresh stool samples and clinical data were collected from all participants, and their bowel functions with SCI were assessed. Microbial diversity and composition were analyzed by sequencing the 16S rRNA gene. The features of gut microbiota correlated with the serum biomarkers and their functions were investigated. The mean NBD score of patients with CTSCI was higher than that of patients with ITSCI. Diversity of the gut microbiota in SCI group was reduced, and with an increase in the degree of damage, alpha diversity had decreased gradually. The composition of gut microbiota in patients with SCI was distinct from that in healthy individuals, and CTSCI group exhibited further deviation than ITSCI group compared to healthy individuals. Four serum biomarkers were found to be correlated with most differential genera. Patients with thoracic SCI present gut dysbiosis, which is more pronounced in patients with CTSCI than in those with ITSCI. Therefore, the gut microbiota profile may serve as the signatures for bowel and motor functions in patients with thoracic SCI.
Introduction
Spinal cord injury (SCI) is a destructive neurological disorder, which is characterized by the disruption of spinal cord [Citation1]. Approximately 2,50,000–5,00,000 new cases are diagnosed with SCI every year mainly due to traffic accidents, sports injuries, and falls [Citation2]. SCI leads to muscle paralysis below the neurological level, thereby leading to a large number of patients bound to wheelchairs. Additionally, a series of complications follow as a result of the damage caused to the nervous system [Citation3]. Neurogenic bowel dysfunction (NBD), a highly prevalent complication, exerts a significantly negative impact on the overall health and well-being of patients with SCI [Citation4]. NBD decreases self-efficacy, reduces quality of life, and often interrupts the rehabilitation therapy sessions due incontinence episodes. The results of a clinical survey demonstrated that most patients with SCI prioritized the recovery of bowel function over the ability to walk, highlighting the importance of bowel management [Citation5–8]. However, routine bowel management for patients with SCI remains unsatisfactory based on diet modification and anorectal stimulation, as these are only the compensation strategies [Citation9]. To develop novel effective methods for bowel management, the identification of biomarkers related to the pathophysiology of impaired gut dysfunction is urgently required.
The gut microbiota have been actively investigated in patients with SCI because of their immunomodulatory role [Citation10,Citation11], potential association with the susceptibility to infections [Citation12], and putative disease-modifying effects [Citation13]. Emerging evidence has shown that SCI affects the normal movement of the gastrointestinal tract, immune surveillance, and intestinal permeability, thereby leading to bacterial translocation and microbial dysbiosis in the gut [Citation14,Citation15]. Ecological dysbiosis followed by traumatic SCI, in turn exacerbates intraspinal inflammation and pathology, which affects the functional recovery [Citation16]. It is hypothesized that gut microbiota could serve as the candidate biomarkers not only for NBD but also for motor recovery in patients with SCI.
Although several researchers have attempted to examine the implications of gut microbiota in the context of SCI [Citation17,Citation18], only a few clinical studies have been reported. Gungor B et al [Citation19] demonstrated in a cross-sectional study that the number of butyrate-producing species in the gut microbiota is reduced in individuals with complete SCI and cauda equina syndrome compared with healthy individuals. Similarly, Zhang C et al [Citation14] reported a decrease in the diversity, as well as altered abundance, of the gut flora in patients with complete SCI, which was subsequently identified to be associated with the serum biomarkers of lipid metabolism in patients with complete cervical SCI; however, gut dysbiosis in patients with incomplete SCI was overlooked in previous studies. As patients with incomplete SCI exhibit greater potential for functional recovery, it is imperative to profile the gut microbiota in them to facilitate the development of rehabilitation strategies for SCI.
In this study, we aimed to test our hypotheses in patients with traumatic thoracic SCI through sequencing the V3-V4 region of 16S rRNA gene and investigate the features of microbiota correlated with the serum biomarkers. Our findings indicate that 1) the composition and function of the gut microbiota are different among patients with complete thoracic SCI (CTSCI), patients with incomplete thoracic SCI (ITSCI), and healthy individuals, and 2) the features of gut microbiota are correlated with the serum biomarkers and implicated in biological functions related to the recovery of thoracic SCI.
Materials and methods
Ethics approval and registration
The present study was examined and approved by the ethics committee of the First Affiliated Hospital of Nanjing Medical University (approval no. 2019-SR-026). The study was registered at the Chinese Clinical Trial Registry (http://www.chictr.org.cn/index.aspx) with the unique identifier ChiCTR1800020450.
Study cohort
A total of 45 patients with thoracic SCI and 24 healthy individuals were enrolled in this study between April 2019 to August 2019. The International Standards for Neurological Classification of Spinal Cord Injury (ISNCSCI, https://asia-spinalinjury.org/isncsci-2019-revision-released/) was employed to assess the extent of SCI in patients after obtaining their signed informed consent [Citation20]. The NBD score was determined to assess the bowel function in patients with SCI [Citation21]. All patients and healthy individuals were provided standard hospital food during the entire observation process of this study.
Patients with the following features were included: (1) 18–70 years of age, (2) traumatic thoracic SCI, and (3) 2–12 months post injury. The exclusion criteria were as follows: (1) antibiotic use within one month before the enrollment; (2) comorbidity with other trauma, burn, or infection; (3) body mass index (BMI) < 18.5 kg/m2 or > 28 kg/m2; and (4) concurrent diabetes, gastrointestinal diseases, autoimmune disease, malignancy, or mental illness. All individuals in the healthy control group were the people who came to the hospital for a medical examination at the same period of time and did not meet any of the foregoing exclusion criteria.
Collection of fecal samples and 16S rRNA sequencing
All participants were provided the same hospital diet for one week. Fresh fecal samples were collected without enema. Samples were stirred properly using disposable sterile wooden sticks to evenly suspend the bacteria. Thereafter, samples were placed into a new 2-mL Thermo Nalgene sterile cryopreservation tube, quickly placed on ice, and transferred to a freezer maintained at ˗80°C for cryopreservation within 1 h after collection. DNA was extracted according to the DNA extraction kit (Promegene Institute, Shenzhen, China), Microbial cells were crushed through glass bead grinding, chemical lysis, and enzymatic hydrolysis to minimize the reduction of microbial composition. After the extraction, the integrity of the extracted genomic DNA was evaluated through 1% agarose gel electrophoresis. Unique fusion primers were designed based on the universal primers set 338 F (5ʹ-ACTCCTACGGGAGGCAGCA-3ʹ) and 806 R (5ʹ-GGACTACHVGGGTWTCTAAT-3ʹ), as well as the barcode sequence. The bacterial 16S rRNA gene was amplified using polymerase chain reaction (95°C for 2 min, followed by 25 cycles at 95°C for 30 s, 55°C for 30 s, and 72°C for 30 s and a final extension at 72°C for 5 min). Thermal cycling procedure, establishment of the amplicon library, and quantification was conducted using the methods described in a previous report [Citation22]. The raw reads were deposited to the NCBI BioProject database (accession no. PRJNA669472).
Bioinformatics and statistical analysis
Data optimization
Raw sequencing reads with more than 8 homopolymers, 2 mismatches in the primers, or 1 mismatch in the barcode were excluded from the analysis. Using the quantitative information available on the microbial ecology platform and the tools/plugins incorporated in QIIME2 (QIIME2, http://qiime2.org/), high quality paired-end sequencing reads were analyzed [Citation23]. First, the DADA2 algorithm was employed to perform the quality control and feature table construction using the original 16S sequence [Citation24]. Redundant and low-incidence (n < 5 in the pool sample) sequences were subsequently removed to generate the representative sequences. Taxonomic classification of the operational taxonomic units (OTUs) was conducted using the classify-sklearn classification methods based on the Greengenes 13.8 database (https://data.qiime2.org/2018.11/common/gg-13-8-99-515-806-nb-classifier.qza) and the q2-feature-classifier plugin. The taxonomic composition was determined based on the OTU annotation.
Diversity and taxonomic composition analysis
Four indicators of the alpha diversity (Shannon’s diversity index, observed OTUs, Faith’s phylogenetic diversity, and Pielou’s evenness) were investigated using the Kruskal-Wallis test and by conducting the principal coordinate analysis via the Bray-Curtis distance. Taxonomic composition of gut microbiota across different groups was evaluated through the LEfSe analysis [Citation25].
Functional enrichment analysis
The PICRUST2 algorithm was employed to evaluate the functional implications of the collected samples [Citation26]. The composition of the Kyoto Encyclopedia of Genes and Genomes (KEGG) orthologs (KOs) (http://www.kegg.jp/.) was computed based on the reference OTUs [Citation27].
Statistical analysis
Data were analyzed and visualized using the R v3.6.1 platform (http://www.r-project.org/). The effect size was analyzed using the adonis function of the R vegan package, where the adonis P-value was calculated using the data of 1,000 permutations. The OTUs and taxa associated with SCI were identified using the Wilcoxon rank-sum test. P-value < 0.05 was considered to as statistically significant.
Results
Gut microbiota are considered as the candidate biomarkers for NBD in patients with SCI. So far, gut dysbiosis in patients with incomplete SCI was overlooked in previous studies. However, in this study, we aimed to test our hypotheses that the microbial composition and function of the gut microbiota are different among patients with CTSCI, patients with ITSCI, and healthy individuals, and that the gut microbiome features are correlated with the serum biomarkers and are implicated in the biological functions related to the recovery of thoracic SCI. We analyzed the differences in the microbial composition and function of gut microbiota among patients with CTSCI, patients with ITSCI, and healthy individuals. Furthermore, we explored the correlation between the serum biomarkers and featured gut microbes.
Sample collection and sequencing summary
A total of 69 subjects were enrolled in the study. Of these subjects, 21 were diagnosed with CTSCI, 24 with ITSCI, and 24 were healthy individuals. The cause of injury included motor vehicle collisions (8 CTSCIs and 9 ITSCIs), fall from an elevated height (8 CTSCIs and 4 ITSCIs), bruised by heavy object (3 CTSCIs and 4 ITSCIs), and others (2 CTSCIs and 7 ITSCIs). There was no significant difference in age, sex, BMI, and dietary habit between the groups (, Table S1).
Figure 1. Histogram of the summary characteristics of all subjects. †CTSCI, complete thoracic spinal cord injury; ITSCI, incomplete thoracic spinal cord injury; HC, healthy control. ALT, alanine aminotransferase; GLU, fasting blood glucose; TCHO, serum total cholesterol; LDL, low-density lipoprotein; UA, uric acid; TG, triglyceride; UREA, serum urea; TBIL, total bilirubin; CR, creatinine; AST, aspartate aminotransferase; HDL, high-density lipoprotein; ALB, albumin; TP, total protein; BMI, body mass index
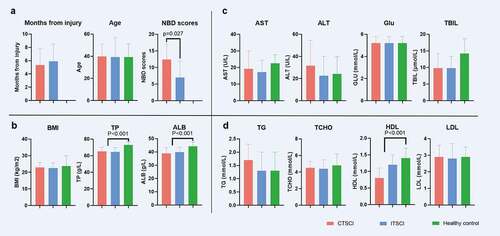
We determined the NBD score (Table S2) to evaluate the bowel function of the subjects with SCI, and the results revealed that the mean NBD score of patients with CTSCI was 12.43 ± 4.66, which was higher than that of patients with ITSCI (7 ± 4.91) (P = 0.027) (). This result indicated that the more severe the SCI, the more obvious the intestinal dysfunction in patients. The causes of injury in descending order were motor vehicle collisions, falls from elevated heights, and bruising by heavy objects. The mean time from injury was 5.64 ± 2.52 months. No significant difference in the post-injury duration was observed between patients with CTSCI and ITSCI (P = 0.406) (). shows the characteristics of all subjects.
Through sequencing the V3-V4 variable region located in the bacterial 16S rRNA gene, the gut microbiota of the 69 collected samples were profiled. With a total of 1426 OTUs identified, the classifications were assigned based on the bioinformatics platform QIIME2 [Citation28]. Of these OTUs, 34% were annotated into specific genera, and 22% into specific species.
Diversity and overall structure of gut microbiota
The alpha diversity was determined based on four indices, including observed OTUs (a measure of species richness), Shannon index (a measure of species richness and species evenness), Faith’s phylogenetic diversity (a measure of species richness), and Pielou’s evenness, which were employed to assess the sample diversity of gut microbiota in all subjects (). The three major indices were higher in the HC group than in patients with SCI when the richness was taken into consideration, and considerable differences were observed between the CTSCI and HC groups. However, no significant differences were observed in the Pielou’s evenness index among these groups. It suggested that as the degree of damage increases, the alpha diversity decreases gradually.
Figure 2. Diversity of gut microbiota among healthy individuals, patients with CTSCI, and ITSCI. (a) Box plot depicting the alpha diversity: observed OTUs, Shannon index, Faith’s phylogenetic diversity, and Pielou’s evenness. (b) PCoA plot depicting the beta diversity based on the Bray-Curtis distance metric. (c) Box plot of the beta diversity, there is a significant difference between the ITSCI and CTSCI groups individually when compared against the healthy cohort *0.01 < P < 0.05 and **p < 0.01. †CTSCI, complete thoracic spinal cord injury; ITSCI, incomplete thoracic spinal cord injury; HC, healthy control
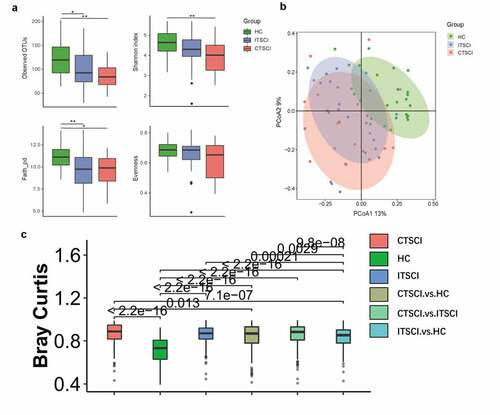
Similarly, for beta diversity, a PCoA plot () based on the Bray-Curtis distance metric () was generated, which demonstrated that two clustering groups (SCI and HC) were generated. Both patients with CTSCI and ITSCI were distinct from healthy individuals. There was a significant difference between the CTSCI and ITSCI groups individually when compared to the HC group (P = 2.2e-16). The CTSCI group deviated more from the healthy individuals than the ITSCI group.
We then compared the effect size of the influencing factors among the CTSCI, ITSCI, and HC groups. The results revealed that SCI and the degree of injury were the main factors, which explained the difference in the structure of the study cohort (Table S3). SCI accounted for 9.8% (adnois P < 0.001) of the gut microbiota variance at the genus level, while the effect size was higher than that observed for other individual characteristic features, including sex, age, BMI, and clinical serum biomarkers.
Alteration in gut taxonomic composition in patients with SCI compared to HCs
LEfSe analysis () of the microbial composition at all taxonomic levels clearly identified 74 SCI-associated microbial taxa (24 CTSCI-enriched and 50 HC-enriched) between patients with CTSCI and healthy individuals [Citation25]. We found that Firmicutes was abundant in both groups, while two phyla, including Actinobacteria and Synergistetes, were significantly enriched in patients with CTSCI.
Figure 3. Cladogram showing differentially abundant gut microbiota among healthy individuals and patients with CTSCI and ITSCI. (a) LEfSe cladogram between healthy individuals and patients with CTSCI. (b) LEfSe cladogram between healthy individuals and patients with ITSCI. (c) LEfSe analysis between patients with CTSCI and ITSCI. †CTSCI, complete thoracic spinal cord injury; ITSCI, incomplete thoracic spinal cord injury; HC, healthy control
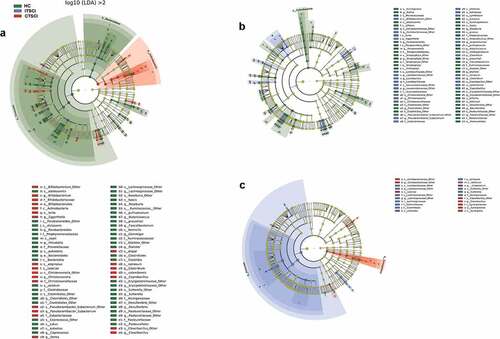
Similarly, 71 SCI-associated microbial taxa (31 ITSCI-enriched and 40 HC-enriched) were identified when patients with ITSCI and healthy individuals were further compared (). At the phylum level, patients with ITSCI exhibited a similar composition of the dominant phyla compared to healthy individuals, except that the levels of several low-abundance phyla (Bacteroidetes, Cyanobacteria, and Proteobacteria) were significantly decreased in patients with ITSCI.
We also compared the composition of gut microbiota between patients with CTSCI and ITSCI to identify the microbial signatures related to the degree of damage (). Synergistetes exhibited the major difference in the more severe CTSCI group followed by Firmicutes and Actinobacteria, whereas the dominant phylum in patients with ITSCI was Firmicutes. At the genus level, we found that four genera, including Coriobacteriaceae, Synergistetes, Eubacterium, and Cloacibacillus, were significantly enriched in patients with CTSCI, while five genera, including Lactobacillaceae, Lachnospiraceae, Eubacterium, Clostridium, and Sutterella, were significantly enriched in patients with ITSCI.
Correlation between SCI-associated microbiota and serum biomarkers
A total of 24 SCI-associated genera were screened (|LDA| > 4) to investigate the correlation between the gut microbiota and serum biomarkers, including 12 HC-enriched genera, 2 ITSCI-enriched genera, and 10 CTSCI-enriched genera (). Among the 13 serum biomarkers, four (aspartate aminotransferase, AST; high-density lipoproteins, HDL; albumin, ALB; and total protein, TP) exhibited significant correlations with most of the genera, thereby demonstrating the impact of their dynamic changes on the structure of SCI-associated microbes. Approximately 91.7% of HC-enriched genera were positively correlated with one or more serum biomarkers. Specifically, Faecalibacterium was found to be associated with the majority of the serum biomarkers.
Figure 4. Correlation heat map analysis of different serum biomarkers between SCI-associated genera. *0.01 < P < 0.05 and **P < 0.01. †SCI, spinal cord injury; HC, healthy control; ALT, alanine aminotransferase; GLU, fasting blood glucose; TCHO, serum total cholesterol; LDL, low-density lipoprotein; UA, uric acid; TG, triglyceride; UREA, serum urea; TBIL, total bilirubin; CR, creatinine; AST, aspartate aminotransferase; HDL, high-density lipoprotein; ALB, albumin; TP, total protein
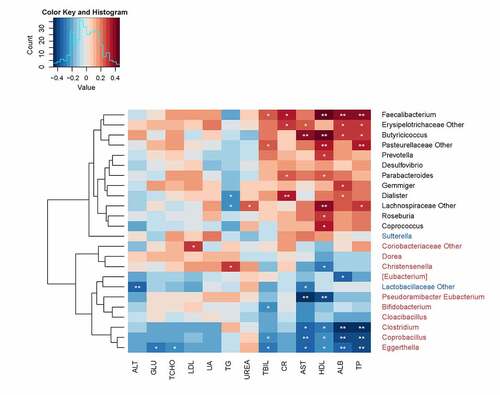
Among ITSCI-enriched genera, there was a significant negative correlation between Lactobacillaceae and two aminotransferases (AST and ALT). Seventy percent of CTSCI-enriched genera were negatively correlated with at least one serum biomarker, and among these genera, Eggerthella, Coprobacillus, and Clostridium were associated with majority of the serum biomarkers. Generally, most of the clinical indicators were negatively correlated with SCI-enriched genera and positively correlated with HC-enriched genera, thereby indicating that the changes in serum biomarkers, especially for serum protein and AST, caused due to SCI were intrinsically related to the structural modification of gut microbiota.
KEGG functional signatures
Based on the 16S OTU profiles, the functional implication of gut microbiota in the subjects with SCI was predicted through the PICRUSt analysis [Citation29], where the relative abundance of 183 KEGG modules was quantified. As a result, 37 modules (20.22%) exhibited significant difference in the abundance between patients with SCI and healthy individuals (P < 0.05), including 17 downregulated and 20 upregulated modules in the SCI groups (). Most of the SCI-enriched modules were found to be associated with purine metabolism (n = 3), carbon fixation (n = 6), and amino acid metabolism (n = 5), while the metabolism of aromatic amino acids (n = 3), cofactors, and vitamins (n = 6) constituted the major functional SCI-reduced modules. All the SCI-enriched modules, along with the SCI-reduced modules, were found to be correlated with one or more SCI-associated genera. In SCI-enriched genera, Christensenella, Bifidobacterium, Pseudoramibacter Eubacterium, and Coriobacteriaceae were associated with the majority of SCI-enriched functional modules, thereby indicating that they play pivotal roles in SCI. In SCI-reduced genera, Faecalibacterium, Dialister, Roseburia, Lachnospiraceae, Butyricicoccus, and Pasteurellaceae played central roles.
Figure 5. Correlation heat map analysis of 37 most abundant KEGG modules exhibiting significant difference between SCI-associated genera. The correlation effect is indicated through a color gradient from blue (negative correlation) to red (positive correlation). *0.01 < P < 0.05 and **P < 0.01. †SCI, spinal cord injury
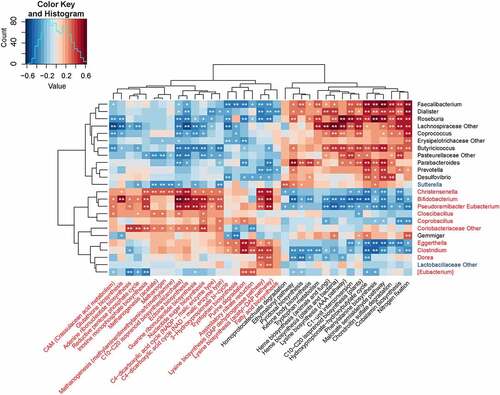
Discussion
In this cross-sectional study, the changes in gut microbiota of patients with thoracic SCI were assessed and compared with those of healthy individuals; the results demonstrated remarkable microbiota dysbiosis and functional changes in patients with thoracic SCI. Especially, as the severity of bowel dysfunction increased, the diversity of the microbial community decreased gradually, and the difference in the structural composition between healthy individuals and patients with thoracic SCI continued to increase with an increase in the degree of injury. These results are concordant with the findings of previous studies [Citation14,Citation19,Citation30], which indicated the occurrence of significant dysbiosis in patients with SCI. Further, our study is the first to report the common gut microbiome signatures between CTSCI and ITSCI, as the results revealed that the diversity of the gut microbiota may be associated with the degree of SCI.
Compared to the healthy individuals, Actinobacteria and Synergistetes were found to be significantly enriched in patients with CTSCI, and both of them are pivotal in the maintenance of gut homeostasis [Citation31,Citation32]. Similarly, altered composition of gut microbiome was observed in patients with ITSCI, wherein the levels of Bacteroidetes, Cyanobacteria, and Proteobacteria were found to be significantly depleted compared to the healthy individuals. Proteobacteria, a phylum comprising endotoxin-harboring, gram-negative bacteria, was observed to be correlated with inflammatory markers in rat models of SCI [Citation33]. However, the depletion of Proteobacteria was notable, as the inflammation in patients with ITSCI was expected to be increased compared to healthy individuals. Therefore, further research on this particular phylum is much required. A comparison between patients with CTSCI and ITSCI highlighted nine genera, with four (Coriobacteriaceae, Synergistetes, Eubacterium, and Cloacibacillus) being enriched in patients with CTSCI, and five (Lactobacillaceae, Lachnospiraceae, Eubacterium, Clostridium, and Sutterella) being significantly enriched in patients with ITSCI. These featured gut microbes could act as relevant biomarkers related to bowel function, as well as disease-modifying processes, given that clinical observations indicated distinct functions between complete and incomplete SCI.
Synergistetes, one of the opportunistic bacteria [Citation34], is known to be involved in the pathogenesis of periodontitis and peri-implantitis [Citation35]. A previous study has demonstrated a positive correlation between Synergistetes and cholesterol, nicotinamide acid, and selenium intake [Citation36]. The variation in the level of Synergistetes may also be related to the changes in cholesterol in patients with SCI and elevated low-density lipoprotein cholesterol and reduced HDL cholesterol [Citation37]. Conversely, neuroprotective effects of nicotinamide have been reported in an experimental model of SCI [Citation38]. In the present study, a strong association between the temporal changes in selenium status and outcome after traumatic SCI was observed [Citation39], suggesting that a selenium deficit might lead to poor outcomes [Citation40]. It appears that the enrichment of Synergistetes may contribute to high susceptibility of metabolic disorders in patients with CTSCI.
As shown in the results, the level of Bacteroidetes was reduced in patients with CTSCI, which is concordant with the findings of a previous study [Citation14]. Bacteroidetes [Citation41] ferment carbohydrates to release single chain fatty acid (SCFA) metabolites, such as acetate, propionate, and butyrate [Citation42]. SCFAs are crucial for providing energy for epithelial cell turnover to maintain gut barrier homeostasis [Citation43]. Additionally, butyrate exerts potent anti-inflammatory effects on macrophages [Citation44] in the central nervous system and suppresses ongoing inflammation. The reduction in the level of Bacteroidetes in patients with CTSCI led to an adverse effect on gut barrier homeostasis and contributed to neurotoxicity in these patients. Vitamin D deficiency induced an increase in the level of Firmicutes, chronic pain, and spinal electrophysiological changes [Citation45]. Cyanobacteria, characterized by pharmacologically significant anti-depressive properties, are considered to be beneficial [Citation46]. Therefore, the reduction in the level of cyanobacteria in patients with ITSCI may contribute to a depressive state post-SCI.
Correlation analysis revealed that altered gut microbiota is correlated with serum biomarkers in patients with thoracic SCI, and most of the serum biomarkers are negatively correlated with the SCI-enriched genera, which indicated that the alterations in gut microbiota may be implicated in multiple metabolic processes. Among them, HC-enriched Faecalibacterium was associated with most of the serum biomarkers. Many studies have demonstrated that Faecalibacterium abundance was reduced in different intestinal disorders [Citation47]. Additionally, it has been reported that the decrease in relative abundance of Faecalibacterium was related to the worse pain scores of patients with encephalomyelitis [Citation48]. However, patients with SCI often exhibit NBD and neuropathic pain. As shown in the KEGG analysis, most of the SCI-enriched modules were associated with purine metabolism, carbon fixation, and amino acid metabolism, while the main functional SCI-reduced modules were associated with the metabolism of aromatic amino acids, cofactors, and vitamins.
The strong point of this study is that it is the first to report the differentiation in gut microbiome signatures between patients with complete SCI and incomplete SCI, which may explain the varying degrees of bowel dysfunction between the SCI groups.
A limitation of our study is that we only focused on the gut microbiota in patients with thoracic SCI. Here, to control the heterogeneity of patients with SCI, we only enrolled patients with thoracic SCI, as it is the most prevalent subtype presented in our hospital. It is impractical to compare patients with different types of SCI due to the small number of subjects included. Additionally, no follow-up information was obtained to delineate the temporal relationship between the gut microbiota and functioning of patients with SCI. Finally, the lack of functional experiments in animal models is considered as a major limitation of the present study, and therefore, the investigation on the relationship between the gut microbiota and somatic function of thoracic SCI is warranted.
Conclusion
We analyzed the differences in the microbial composition and function of the gut microbiota among patients with CTSCI, patients with ITSCI, and healthy individuals. Additionally, we elucidated the correlation between the serum biomarkers and characterized gut microbes, which could be the potential targets for disease prognosis and diagnosis.
Highlights:
We focused on gut dysbiosis in individuals with incomplete thoracic spinal cord injury (SCI).
We found that there is a correlation between bowel dysfunction and SCI.
We propose that the gut microbes can be considered as therapeutic targets for varying degrees of SCI. (This is one of our highlights. Thank you)
Supplemental Material
Download ()Disclosure statement
No potential conflict of interest was reported by the author(s).
Supplementary material
Supplemental data for this article can be accessed here
Additional information
Funding
References
- Kisala PA, Victorson D, Pace N, et al. Measuring psychological trauma after spinal cord injury: development and psychometric characteristics of the SCI-QOL Psychological Trauma item bank and short form. J Spinal Cord Med. 2015 May;38(3):326–334.
- Lim SW, Shiue YL, Ho CH, et al. Anxiety and depression in patients with traumatic spinal cord injury: a nationwide population-based cohort study. PLoS One. 2017;12(1):e0169623.
- McKinley WO, Jackson AB, Cardenas DD, et al. Long-term medical complications after traumatic spinal cord injury: a regional model systems analysis. Arch Phys Med Rehabil. 1999 Nov;80(11):1402–1410.
- Benevento BT, Sipski ML. Neurogenic bladder, neurogenic bowel, and sexual dysfunction in people with spinal cord injury. Phys Ther. 2002 Jun;82(6):601–612.
- Lynch AC, Antony A, Dobbs BR, et al. Bowel dysfunction following spinal cord injury. Spinal Cord. 2001 Apr;39(4):193–203.
- Anderson KD. Targeting recovery: priorities of the spinal cord-injured population. J Neurotrauma. 2004 Oct;21(10):1371–1383.
- Simpson LA, Eng JJ, Hsieh JT, et al. Spinal cord injury rehabilitation evidence scire research, t. the health and life priorities of individuals with spinal cord injury: a systematic review. J Neurotrauma. 2012 May 20;29(8):1548–1555.
- Sezer N, Akkus S, Ugurlu FG. Chronic complications of spinal cord injury. World J Orthop. 2015 Jan 18;6(1):24–33.
- Stoffel JT, Van Der Aa F, Wittmann D, et al. Neurogenic bowel management for the adult spinal cord injury patient. World J Urol. 2018 Oct;36(10):1587–1592.
- Benarroch EE. Autonomic nervous system and neuroimmune interactions: new insights and clinical implications. Neurology. 2019 Feb 19;92(8):377–385.
- Kigerl KA, Zane K, Adams K, et al. The spinal cord-gut-immune axis as a master regulator of health and neurological function after spinal cord injury. Exp Neurol. 2020 Jan;323:113085.
- Jogia T, Ruitenberg MJ. Traumatic spinal cord injury and the gut microbiota: current insights and future challenges. Front Immunol. 2020;11:704.
- Kigerl KA, Mostacada K, Popovich PG. Gut microbiota are disease-modifying factors after traumatic spinal cord injury. Neurotherapeutics. 2018 Jan;15(1):60–67.
- Zhang C, Zhang W, Zhang J, et al. Gut microbiota dysbiosis in male patients with chronic traumatic complete spinal cord injury. J Transl Med. 2018 Dec 13;16(1):353.
- Krassioukov A, Eng JJ, Claxton G, et al. Neurogenic bowel management after spinal cord injury: a systematic review of the evidence. Spinal Cord. 2010 Oct;48(10):718–733.
- Kigerl KA, Hall JC, Wang L, et al. Gut dysbiosis impairs recovery after spinal cord injury. J Exp Med. 2016 Nov 14;213(12):2603–2620.
- O’Connor G, Jeffrey E, Madorma D, et al. Investigation of microbiota alterations and intestinal inflammation post-spinal cord injury in rat model. J Neurotrauma. 2018 Sep 15;35(18):2159–2166.
- Wallace DJ, Sayre NL, Patterson TT, et al. Spinal cord injury and the human microbiome: beyond the brain-gut axis. Neurosurg Focus. 2019 Mar 1;46(3):E11.
- Gungor B, Adiguzel E, Gursel I, et al. Intestinal microbiota in patients with spinal cord injury. PLoS One. 2016;11(1):e0145878.
- Kirshblum S, Waring W. 3rd. updates for the international standards for neurological classification of spinal cord injury. Phys Med Rehabil Clin N Am. 2014 Aug;25(3):505–517. . vii.
- Krogh K, Christensen P, Sabroe S, et al. Neurogenic bowel dysfunction score. Spinal Cord. 2006 Oct;44(10):625–631.
- Cao J, Amakye WK, Qi C, et al. Bifidobacterium Lactis Probio-M8 regulates gut microbiota to alleviate Alzheimer’s disease in the APP/PS1 mouse model. Eur J Nutr. 2021Apr;60:1.
- Caporaso JG, Kuczynski J, Stombaugh J, et al. QIIME allows analysis of high-throughput community sequencing data. Nat Methods. 2010 May;7(5):335–336.
- Callahan BJ, McMurdie PJ, Rosen MJ, et al. DADA2: high-resolution sample inference from Illumina amplicon data. Nat Methods. 2016 Jul;13(7):581–583.
- Segata N, Izard J, Waldron L, et al. Metagenomic biomarker discovery and explanation. Genome Biol. 2011 Jun 24;12(6):R60.
- Langille MG, Zaneveld J, Caporaso JG, et al. Predictive functional profiling of microbial communities using 16S rRNA marker gene sequences. Nat Biotechnol. 2013 Sep;31(9):814–821.
- Kanehisa M, Furumichi M, Tanabe M, et al. KEGG: new perspectives on genomes, pathways, diseases and drugs. Nucleic Acids Res. 2017 Jan 4;45(D1):D353–D361.
- Bolyen E, Rideout JR, Dillon MR, et al. Author Correction: reproducible, interactive, scalable and extensible microbiome data science using QIIME 2. Nat Biotechnol. 2019 Sep;37(9):1091.
- Douglas GM, Maffei VJ, Zaneveld J, et al. PICRUSt2: an improved and extensible approach for metagenome inference. 2019. bioRxiv.
- Zhang C, Jing Y, Zhang W, et al. Dysbiosis of gut microbiota is associated with serum lipid profiles in male patients with chronic traumatic cervical spinal cord injury. Am J Transl Res. 2019;11(8):4817–4834.
- Binda C, Lopetuso LR, Rizzatti G, et al. Actinobacteria: a relevant minority for the maintenance of gut homeostasis. Dig Liver Dis. 2018 May;50(5):421–428.
- Hugenholtz P, Hooper SD, Kyrpides NC. Focus: synergistetes. Environ Microbiol. 2009 Jun;11(6):1327–1329.
- Myers SA, Gobejishvili L, Saraswat Ohri S, et al. Following spinal cord injury, PDE4B drives an acute, local inflammatory response and a chronic, systemic response exacerbated by gut dysbiosis and endotoxemia. Neurobiol Dis. 2019 Apr;124:353–363.
- Marchandin H, Damay A, Roudière L, et al. Phylogeny, diversity and host specialization in the phylum Synergistetes with emphasis on strains and clones of human origin. Res Microbiol. 2010;161(2):91–100.
- Yu XL, Chan Y, Zhuang LF, et al. Distributions of Synergistetes in clinically-healthy and diseased periodontal and peri-implant niches. Microb Pathog. 2016;94:90–103.
- Nuli R, Cai J, Kadeer A, et al. Integrative analysis toward different glucose tolerance-related gut microbiota and diet. Front Endocrinol (Lausanne). 2019;10:295.
- Olivia Gilbert, James R, Croffoot Allen; J. Taylor, Mark Nash. Serum lipid concentrations among persons with spinal cord injury – a systematic review and meta-analysis of the literature. Atherosclerosis. 2014.
- Brewer KL, Hardin JS. Neuroprotective effects of nicotinamide after experimental spinal cord injury. Acad Emerg Med. 2004 Feb;11(2):125–130.
- Seelig J, Heller RA, Hackler J, et al. Selenium and copper status - potential signposts for neurological remission after traumatic spinal cord injury. J Trace Elem Med Biol org Soc Min Trace Elem (GMS). 2020 Jan 57:126415. .
- Heller RA, Seelig J, Bock T, et al. Relation of selenium status to neuro-regeneration after traumatic spinal cord injury. J Trace Elem Med Biol org Soc Min Trace Elem (GMS). 2019 Jan 51:141–149. .
- Linares DM, Ross P, Stanton C. Beneficial Microbes: the pharmacy in the gut. Bioengineered. 2016;7(1):11–20.
- Roediger WE. Short chain fatty acids as metabolic regulators of ion absorption in the colon. Acta veterinaria Scandinavica Supplementum. 1989;86:116–125.
- Scaldaferri F, Pizzoferrato M, Gerardi V, et al. The gut barrier: new acquisitions and therapeutic approaches. J Clin Gastroenterol. 2012 Oct;46(Suppl):S12–17.
- Zhao X, Zhang Z, Hu B, et al. Response of gut microbiota to metabolite changes induced by endurance exercise. Front Microbiol. 2018;9:765.
- Guida F, Boccella S, Belardo C, et al. Altered gut microbiota and endocannabinoid system tone in vitamin D deficiency-mediated chronic pain. Brain Behav Immun. 2020 Mar;85:128–141.
- Lax NC, Ahmed KT, Ignatz CM, et al. Marine cyanobacteria-derived serotonin receptor 2C active fraction induces psychoactive behavioral effects in mice. Pharm Biol. 2016 Nov;54(11):2723–2731.
- Lopez-Siles M, Duncan SH, Garcia-Gil LJ, et al. Faecalibacterium prausnitzii: from microbiology to diagnostics and prognostics. Isme J. 2017;11(4):841.
- Nagy-Szakal D, Williams BL, Mishra N, et al. Fecal metagenomic profiles in subgroups of patients with myalgic encephalomyelitis/chronic fatigue syndrome. Microbiome, 2017;5(1): 44..