ABSTRACT
Asthma occurs accompanied by the ferroptosis in bronchial epithelial cells, during which Interleukin-6 (IL-6) plays a key role. However, the associations between IL-6, ferroptosis and asthma have not been reported. Bronchial epithelial cells BEAS-2B cells were induced by different concentrations of IL-6 and cell viability was detected by MTT assay. The TBARS production rate was detected by corresponding kit. The expression of oxidative stress-related indexes was detected by ELISA. The Iron Assay Kits detected total iron levels and ferrous ion (Fe2+) levels. Labile iron pool assay was used to detect the cell unstable iron pool. The expression of ferroptosis-related proteins was detected by Western blot. To further examine the mechanism of action, ferroptosis inhibitor Ferrostatin 1 (Fer-1), antioxidant NAC, and the iron supplement Fe were added. We found that IL-6 decreased the activity, promoted lipid peroxidation, disrupted iron homeostasis of BEAS-2B cells, and induced iron death in bronchial epithelial BEAS-2B cells. However, pretreatment with Ferrostatin-1 (Fer-1) and antioxidant NAC partially reversed the effect of IL-6 on lipid peroxidation and ferroptosis in BEAS-2B cells, while Fe augmented the effect. Overall, IL-6 promotes ferroptosis in bronchial epithelial cells by inducing reactive oxygen species (ROS)-dependent lipid peroxidation and disrupting iron homeostasis.
Introduction
Asthma is a common chronic inflammatory airway disease that affects more than 300 million people worldwide [Citation1]. Airway epithelial cells are the structural cells of the airway and the body’s first defense against inhaled stimuli, allergens, and pathogens [Citation2]. Epithelial cells can synthesize and release a variety of inflammatory response mediators and cytokines, activate innate and adaptive immune cells, promote tissue regeneration and repair the damage triggered by environmental factors [Citation3]. Studies have shown that defective repair of airway epithelial cells is one of the etiology of asthma [Citation4,Citation5]. The healing ability of epithelial cells in asthmatic patients was significantly lower than that in normal people [Citation6]. Airway epithelial cells play an important role in the occurrence and development of chronic inflammatory diseases of the airway. It has become a hot spot of research on the pathogenesis and therapeutic target of chronic airway inflammatory diseases.
The important pro-inflammatory factor IL-6 induces the polarization of initial T cells to T helper cell 17 (Th17) cells and is in turn associated with neutrophil recruitment that can aggravate airway inflammation in asthma [Citation7]. Recent evidence has suggested that the level of IL-6 in peripheral blood of patients with allergic asthma was increased significantly during the onset of the disease [Citation8]. Michael C et al. [Citation9] found that the expression of IL-6 in plasma of patients with asthma was significantly increased through cross-sectional study, suggesting that plasma IL-6 could be as a predictive biomarker for asthma. In addition, a significant association was found between serum IL-6 and asthma attack risk [Citation10]. The IL-6 signaling pathway in dendritic cells plays a critical role in the uptake of allergens and the initiation of Th2/Th17 mediated airway inflammation and airway hyperresponsiveness in asthma, thus providing a new potential target for the treatment of allergic asthma [Citation11]. Blocking the IL-6 pathway alleviates airway inflammation in asthmatic mice [Citation12]. Thus, epithelial IL-6 signal transduction defines a novel asthma phenotype with increased airway inflammation [Citation13].
Ferroptosis is a new type of programmed cell death characterized by intracellular iron accumulation and lipid peroxidation, which ultimately leads to cellular oxidative stress and cell death [Citation14]. During ferroptosis, SLC7A11, a member of the solute carrier family, is down-regulated in ferroptosis cells and the deletion of SLC7A11 gene leads to lipid peroxidation and ferroptosis [Citation15]. Intracellular iron homeostasis is mainly post transcriptional regulated by iron metabolism-related genes via iron response elements (iron regulatory protein system), such as ferritin (heavy-chain FTH1 and light chain FTL) [Citation16]. Iron output is mediated by ferroportin 1 (FPN1, also known as SLC11A3), which releases Fe2+ into the cell and oxidize ferric to ferric [Citation17]. At present, there are relatively few studies on the manifestations and mechanisms of ferroptosis related to asthma, but the existing studies suggest that ferroptosis plays an important role in the occurrence and development of asthma, especially in moderate to severe asthma. Inhibition of ferroptosis and oxidative stress contribute to the inhibitory effect of acupuncture on airway inflammation in an ovalbumin-induced mouse asthma model [Citation18]. Induction of ferroptosis-like cell death exerts synergistic effects with glucocorticoids in allergic airway inflammation [Citation19]. In addition, IL-6 causes ferroptosis of chondrocytes by inducing cellular oxidative stress and interfering with iron homeostasis [Citation20]. IL-6 has an isozyme-specific effect on the expression of glutathione peroxidase (GPX). IL-6 can increase the GPX2 transcription concentration and decrease the GPX4 transcription concentration [Citation21], while GPX plays a key role in the occurrence and development of ferroptosis [Citation22]. However, the regulation of the relationship between IL-6 and ferroptosis in asthma has not been reported so far.
Our paper shows that IL-6 in asthma exacerbates asthma symptoms by inducing ferroptosis. Our paper can provide a solid theoretical basis for the clinical targeting of IL-6 in the treatment of asthma. In addition, in a clinical study, Brittany Esty et al. [Citation23] treated two patients with severe persistent non-atopic asthma with tocilizumab, a human anti-IL-6 receptor (IL-6 R) monoclonal antibody. And the lung function of asthma patient improved and Th2 and Th17 effector cells decreased. However, at present, IL-6 McAb has not been widely used in the clinical treatment of asthma, and more clinical studies need to be reported. We have added the above to the discussion of the article.
Therefore, we hypothesized that IL-6 may play a role in asthma disease through the regulation of ferroptosis. In this paper, we discuss the role of IL-6 in asthma and its mechanism of action on ferroptosis of bronchial epithelial cells. Our paper lays a foundation for future research on the mechanism of ferroptosis in asthma.
Materials and methods
Cell culture model induction
Normal bronchial epithelial cells (BEAS-2B cells, BNCC254518) were obtained from the BeiNa Biological Technology Co., Ltd. Cells were cultured in DMEM (Gibco; Thermo Fisher Scientific, Inc.) with 10% FBS (Gibco; Thermo Fisher Scientific, Inc.) and 1% double resistant at 37℃ with CO2. Different concentrations of IL-6 (0, 5 ng/ml, 10 ng/ml, 25 ng/ml, 50 ng/ml) induced BEAS-2B cells for 24 h [Citation20]. To further verify the effect of IL-6 on lipid peroxidation and ferroptosis in BEAS-2B cells, we added ferroptosis inhibitor Fer-1 (0.1 μM, CAS 347,174–05-4, Sigma–Aldrich, USA), iron supplement Fe (3.3 M, CAS 2238–05-8, Sigma–Aldrich, USA) [Citation24] and antioxidant NAC (1 mM, CAS 616–91-1, Sigma–Aldrich, USA) [Citation25]. The cells were divided into Control, IL-6, Fe+IL-6, Fer-1 + IL-6 and NAC+IL-6 groups.
MTT(3-(4,5-Dimethylthiazol-2-yl)-2,5-diphenyltetrazolium bromide)
Cells were planted in 96-well plates at a density of 8 × 103 cell/well. After corresponding treatment, 10 μL MTT solution was added into each well for 6 h, and the supernatant was discarded. Then 120 μL DMSO was used to detect the absorbance of each well at 490 nm with a microplate analyzer.
Thiobarbituric acid reactive substances (TBARS) assay
Lipid peroxidation in the cells was estimated using the thiobarbituric acid-reactive substance (TBARS) assay [Citation26]. 0.25 mL 15% (W/V) trichloroacetic acid and 7 μL of 500 mM butylated hydroxyanisole (BHA) were added to the cell lysate. The mixture were then centrifuged at 1000 g for 5 min. The supernatant was taken and 0.5 ml 0.375% (w/v) thiobarbituric acid was added. The mixture was then boiled for 10 min. After the mixture was cooled, TBARS was measured at 532 nm using a microplate reader (FilterMax F3/F5 Multi-Mode Microplate Reader, Molecular Devices).
Lipid peroxidation assay
Glutathione peroxidase (GSH-px, S0073), Malondialdehyde (MDA, S0131M) and reactive oxygen species (ROS, S0033M) levels in BEAS-2B cells were measured using corresponding detection kits purchased form Beyotime Institute of Biotechnology (Shanghai, China) in accordance with the manufacturer’s instructions.
Iron measurements
After the cells were treated by corresponding procedures, the total iron content (MAK025, Sigma–Aldrich) and Fe2+ content (AB83366, Abcam, UK) in the cells were detected by the corresponding kits according to the manufacturer’s protocol [Citation27]. In brief, cells were added to an iron assay buffer, homogenized on ice, and then centrifuged at 13,000 × g for 10 min at 4°C, to obtain the supernatant for the assay. Finally, a 50-μL supernatant was incubated with 50 μL of assay buffer in a 96-well microplate for 30 min at room temperature. And then 50 μL of assay buffer was incubated with 200 μL of reagent mix in the dark for 30 min at room temperature. The microplate reader was used to measure the absorbance at 593 nm.
Labile iron pool (LIP) assay
The LIP levels were measured using calcein-acetoxymethyl ester (calcein-AM, MedChemExpress, NJ, USA) and deferiprone (MedChemExpress), according to the methods described in the literature [Citation28]. BEAS-2B cells were seeded in 12-well plates at a density of 6 × 105 cells per well. After treated, cells were incubated with 0.05 μM Calcein AM for 15 min at 37°C. Then, cells were incubated with deferiprone for 1 h at 37°C. The medium containing deferiprone was replaced with fresh medium, and the cells were examined and imaged with a fluorescence microscope (BioTek Synergy 2).
Western blot
BEAS-2B cells were collected and lysed with 0.5 μL RIPA lysis buffer (Beyotime Institute of Biotechnology) on ice for 30 min. Then, proteins were detected using a BCA protein assay kit (Bio-Rad Laboratories, Inc.). 30 µg of protein samples were separated by 10% SDS-PAGE and then transferred onto polyvinylidene fluoride (PVDF) membranes. After being blocked with 5% skimmed milk for 2 h at room temperature, the membranes were incubated with primary antibodies overnight at 4°C at 1:1000 dilution. On the next day, the membranes were incubated with horseradish peroxidase-conjugated secondary antibody (goat anti-rabbit IgG,1:5000, ab172130, Abcam). The signals were detected using enhanced chemiluminescence reagent (GE Healthcare) and Image J software (version 146; National Institutes of Health, Bethesda, MD, USA) was used to analyze the fold-changes of protein levels. The information of primary antibodies were as follows: anti-SLC7A11 (ab175186, Abcam, UK), anti- GPX4 (ab125066, Abcam, UK), anti- FTH1 (ab75972, Abcam, UK), anti- FPN1 (ab78066, Abcam, UK) and anti-GAPDH (ab8245, Abcam, UK).
Statistical analysis
The data are presented as mean ± standard deviation (SD). A multigroup analysis was performed using one-way ANOVA, followed by Tukey’s post hoc analysis to compare the two groups. Statistical analysis was performed with Prism 5.0 (GraphPad Software, La Jolla, CA). p < 0.05 was considered statistically significant.
Results
IL-6 decreased the activity and promoted lipid peroxidation of BEAS-2B cells
To detect the effect of IL-6 on BeAS-2B cells, MTT assay was used to detect the viability of BEAS-2B cells. The results showed that cell viability of these cells was decreased by IL-6 in a dose-dependent manner (). Subsequently, the TBARS production rate was detected by the kit to determine the cell lipid peroxidation level. The results showed that, compared with the control group, the expression of TBARS in cells induced by IL-6 was significantly increased, indicating that IL-6 can promote cell lipid peroxidation (). The levels of GSH-Px, MDA and ROS in cells were detected by the kits. We found that, compared with the control group, the expressions of MDA and ROS were significantly increased and the expression of GSH-Px was significantly decreased after IL-6 induction (). These results suggested that IL-6 decreased the activity and promoted lipid peroxidation of BEAS-2B cells.
Figure 1. IL-6 decreased the activity and promoted lipid peroxidation of BEAS-2B cells. A. MTT assay detected the cell viability. B. The TBARS production rate was determined by the kit. C. The corresponding kits were used to detect the expression of the indexes related to oxidative stress. *p < 0.05, **p < 0.01, ***p < 0.001 vs Control
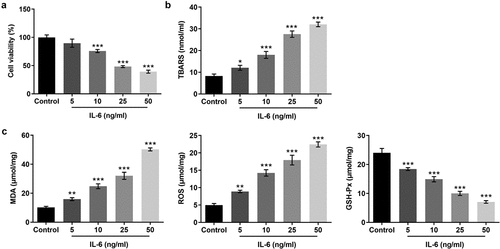
IL-6 disrupted iron homeostasis and induced ferroptosis of BEAS-2B cells
The iron kits were used to measure the levels of total iron and Fe2+ in cells. With the increase of IL-6-induced concentration, the levels of total iron and Fe2+ in cells were increased significantly ( and b). The level of free iron can be determined by LIP Assay. The results showed that the LIP level was significantly decreased after IL-6 induction compared with the control group, indicating that the Fe2+ level in cells was increased (). This result is consistent with the previous expression trend of total iron and Fe2+. The expression of ferroptosis-related proteins was detected by Western blot. Compared with the control group, after IL-6 induction, the expressions of SLC7A11, GPX4, FTH1, and FPN1 were decreased while the expression of NOX1 was increased in a dose-dependent manner (). The induction trend of IL-6 was the most obvious at the concentration of 50 ng/ml, so we chose 50 ng/ml for subsequent experiments. These results showed that IL-6 disrupted iron homeostasis and induced ferroptosis of BEAS-2B cells.
Figure 2. IL-6 disrupted iron homeostasis and induced ferroptosis of BEAS-2B cells. A. Iron Assay Kit detected total iron levels in cells. B. Iron Assay Kit detected Fe2+ levels in cells. C. LIP Assay was used to detect the iron expression. D. Western blot detected the expression of ferroptosis-related proteins. *p < 0.05, **p < 0.01, ***p < 0.001 vs Control
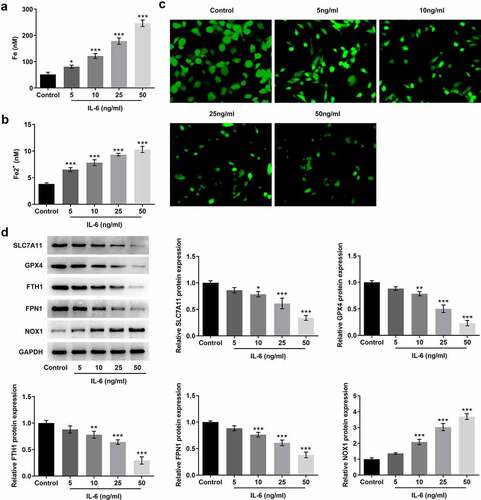
Pretreatment with Fer-1 and NAC partially reversed the effect of IL-6 on lipid peroxidation and ferroptosis in BEAS-2B cells, while Fe increased those effects
To further verify the effect of IL-6 on lipid peroxidation and ferroptosis in BEAS-2B cells, we added ferroptosis inhibitor Fer-1, iron supplement Fe, and antioxidant NAC. MTT results showed that compared with IL-6, the cell viability of Fe+IL-6 group was significantly decreased, while the cell viability of Fer-1 + IL-6 and NAC + IL-6 was significantly increased (). The results of lipid peroxidation showed that compared with IL-6, the lipid peroxidation level of Fe + IL-6 group was further increased, while the lipid peroxidation level of Fer-1 + IL-6 and NAC + IL-6 cells was reversed (). Compared with IL-6, the expression of MDA and ROS in Fe + IL-6 group was further increased, while the expression of GSH-Px was further decreased. The expressions of MDA, ROS and GSH-Px in Fer-1+ IL-6 and NAC+IL-6 cells presented opposite trends (). The iron homeostasis of the cells was then detected. The results showed that compared with the IL-6 group, the total iron and Fe2+ levels in the Fe+IL-6 group were increased, while the LIP level was further decreased. The levels of total iron and Fe2+ in Fer-1+ IL-6 group were decreased significantly, while the level of LIP was further increased. There were no significant change in total iron, Fe2+ and LIP levels in the NAC + IL-6 group (). Western blot results showed that compared with the IL-6 group, the expressions of SLC7A11, GPX4, FTH1, and FPN1 in Fe + IL-6 group were further decreased, while the expression of NOX1 was further increased. The expressions of SLC7A11, GPX4, FTH1, and FPN1 in Fer-1 + IL-6 group were significantly increased, while the expression of NOX1 was significantly decreased. There was no significant change in ferroptosis-related proteins in the NAC + IL-6 group ().
Figure 3. Pretreatment with Fer-1 and NAC partially reversed the effect of IL-6 on lipid peroxidation in BEAS-2B cells, while Fe increased those effects. A. MTT assay detected the cell viability after the induction of Fer-1, Fe and NAC. B. The TBARS production rate was determined by the kit after the induction of Fer-1, Fe and NAC. C. The corresponding kits were used to detect the expression of the indexes related to oxidative stress after the induction of Fer-1, Fe and NAC. ***p < 0.001 vs Control; #p < 0.05, ###p < 0.001 vs IL-6
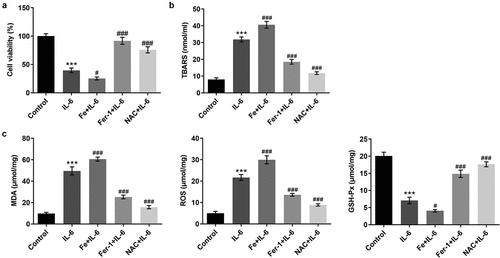
Figure 4. Pretreatment with Fer-1 and NAC partially reversed the effect of IL-6 on ferroptosis in BEAS-2B cells, while Fe increased those effects. A. Iron Assay Kit detected total iron levels in cells after the induction of Fer-1, Fe and NAC. B. Iron Assay Kit detected Fe2+ levels in cells after the induction of Fer-1, Fe and NAC. C. LIP Assay was used to detect the iron expression after the induction of Fer-1, Fe and NAC. D. Western blot detected the expression of ferroptosis-related proteins after the induction of Fer-1, Fe and NAC. ***p < 0.001 vs Control; #p < 0.05, ##p < 0.01, ###p < 0.001 vs IL-6
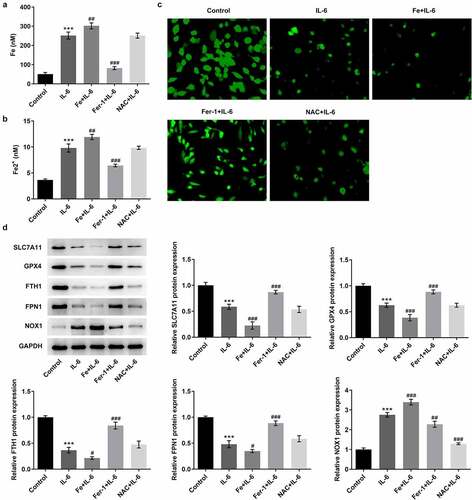
Discussion
The normal bronchial epithelial cells act as a closed self-cleaning barrier in the body against inhaled allergens, pathogens, and other harmful substances [Citation29]. Once damaged, they will release a large number of chemokines and cytokines, which can not only induce acute inflammatory response, but also regulate subsequent innate and adaptive immune responses [Citation30]. Therefore, airway epithelial cells play an important role in asthma, which was why airway epithelial cells BEAS-2B were selected in this study for the experiments.
Ferroptosis is a type of cell death that plays an important role in iron homeostasis in asthma. In asthmatic airway epithelial cells, the interaction between ferroptosis and autophagy affect the pathological process of asthma, which may be used as a target for the treatment of asthma [Citation31]. As accelerating eosinophilic death can effectively reduce allergic airway inflammation, the use of ferroptosis inducer that can potently complete this task may play a therapeutic role in allergic airway inflammation [Citation19]. In an experimental model of asthma induced by house dust mite (HDM), the association between elevated lung iron levels and enhanced expression of TFR1 in airway tissue suggested that ferroptosis plays a role in the pathogenesis and severity of asthma and may be a potential therapeutic target [Citation32]. A study published in Cell in 2017 showed a positive correlation between lipid peroxidation in airway epithelial cells and exhaled nitric oxide levels in asthmatic patients. Cigarette smoke, infection, and other factors that can lead to the decrease of GPX4 activity can cause the imbalance of lipid redox in epithelial cells, resulting in airway epithelial dysfunction and aggravating airway inflammation in asthma [Citation33]. In the experiment, we detected the level of ferroptosis in BEAS-2B cells by measuring the level of lipid peroxidation and iron homeostasis.
IL-6 is a common marker of inflammation. However, recent studies suggested IL-6 as an inducer for pathogenesis of inflammatory diseases besides its widely known function as a pro-inflammatory marker [Citation34]. IL-6 signaling pathway plays a key role in the control of differentiation and activation of T lymphocytes by inducing JAK/STAT-3 and Ras/ ERK/C/EBP pathways [Citation35]. IL-6 can induce the polarization of primary T cells toward Th17 cells and promote neutrophil recruitment, thereby aggravating airway inflammation in asthma [Citation11]. However, the specific mechanism has not been reported. Our experiment found that after IL-6 acted on bronchial epithelial cells, cell viability was decreased and lipid peroxidation occurred. In addition, IL-6 disrupted iron homeostasis and induced ferroptosis in BEAS-2B cells.
Conclusion
Therefore, our experiment demonstrated that IL-6 promotes ferroptosis of bronchial epithelial cells BEAS-2B by inducing ROS-dependent lipid peroxidation and disrupting iron homeostasis. This study lays a foundation for the study on the role of IL-6 and ferroptosis in asthma disease.
Limitations and future direction
There are some limitations in this article. IL-6 induces ferroptosis in BEAS-2B cells, and the expressions of SLC7A11, GPX4, FTH1, and FPN1 are significantly decreased. However, the specific regulatory mechanism has not been explored. In addition, our paper is a validation at the cellular level, but it has not been carried out on the animal level. Those experiments need to be further explored in our laboratory in the following experiments.
Research highlights
IL-6 induces ROS-dependent lipid peroxidation and disruption of iron homeostasis
IL-6 induced ferroptosis in bronchial epithelial cells BEAS-2B
IL-6 exacerbates asthma by promoting ferroptosis in BEAS-2B cells
Ethics approval and consent to participate
Not applicable
Consent for publication
All authors read and approved the final manuscript and agree to publish the article
Availability of data and materials
The datasets analyzed during the current study are available from the corresponding author on reasonable request.
Authors’ contributions
Zhonghu Bai and Yankun Yang contributed to the conception and design of the present study, analyzed and interpreted the data, and critically revised the manuscript for important intellectual content. Fei Han and Shijie Li contributed to designing the study, analyzed the data, and drafted and revised the manuscript. All authors read and approved the final manuscript.
Acknowledgements
Not applicable
Disclosure statement
The authors declare that they have no competing interests.
Additional information
Funding
References
- Papi A, Brightling C, Pedersen SE, et al. Asthma. Lancet. 2018;391(10122):783–800. PubMed PMID: 29273246.
- Frey A, Lunding LP, Ehlers JC, et al. More than just a barrier: the immune functions of the airway epithelium in asthma pathogenesis. Front Immunol. 2020;11:761. PubMed PMID: 32411147; PubMed Central PMCID: PMCPMC7198799.
- Hiemstra PS, McCray PB Jr., Bals R. The innate immune function of airway epithelial cells in inflammatory lung disease. Eur Respir J. 2015;45(4):1150–1162. PubMed PMID: 25700381; PubMed Central PMCID: PMCPMC4719567.
- Shi W, Bellusci S, Warburton D. Lung development and adult lung diseases. Chest. 2007;132(2):651–656. PubMed PMID: 17699136.
- Martinovich KM, Iosifidis T, Buckley AG, et al. Conditionally reprogrammed primary airway epithelial cells maintain morphology, lineage and disease specific functional characteristics. Sci Rep. 2017;7(1):17971. PubMed PMID: 29269735; PubMed Central PMCID: PMCPMC5740081.
- Inoue H, Hattori T, Zhou X, et al. Dysfunctional ErbB2, an EGF receptor family member, hinders repair of airway epithelial cells from asthmatic patients. J Allergy Clin Immunol. 2019;143(6):2075–2085. e10. PubMed PMID: 30639343; PubMed Central PMCID: PMCPMC6556416.
- Lipworth B, Chan R, Systemic KC. IL-6 and Severe Asthma. Am J Respir Crit Care Med. 2020;202(9):1324–1325. PubMed PMID: 32687393; PubMed Central PMCID: PMCPMC7605205.
- Li X, Hastie AT, Peters MC, et al. Investigation of the relationship between IL-6 and type 2 biomarkers in patients with severe asthma. J Allergy Clin Immunol. 2020;145(1):430–433. PubMed PMID: 31513878; PubMed Central PMCID: PMCPMC7469890.
- Peters MC, Mauger D, Ross KR, et al. Evidence for exacerbation-prone asthma and predictive biomarkers of exacerbation frequency. Am J Respir Crit Care Med. 2020;202(7):973–982. PubMed PMID: 32479111; PubMed Central PMCID: PMCPMC7528796.
- Jackson DJ, Bacharier LB, Calatroni A, et al. Serum IL-6: a biomarker in childhood asthma? J Allergy Clin Immunol. 2020;145(6):1701–4e3. PubMed PMID: 32004524; PubMed Central PMCID: PMCPMC7282967.
- Lin YL, Chen SH, Wang JY. Critical role of IL-6 in dendritic cell-induced allergic inflammation of asthma. J Mol Med (Berl). 2016;94(1):51–59. PubMed PMID: 26232935.
- Schmit T, Ghosh S, Mathur RK, et al. IL-6 deficiency exacerbates allergic asthma and abrogates the protective effect of allergic inflammation against streptococcus pneumoniae pathogenesis. J Immunol. 2020;205(2):469–479. PubMed PMID: 32540994; PubMed Central PMCID: PMCPMC7336542.
- Jevnikar Z, Ostling J, Ax E, et al. Epithelial IL-6 trans-signaling defines a new asthma phenotype with increased airway inflammation. J Allergy Clin Immunol. 2019;143(2):577–590. PubMed PMID: 29902480.
- Liang C, Zhang X, Yang M, et al. Recent progress in ferroptosis inducers for cancer therapy. Adv Mater. 2019;31(51):e1904197. PubMed PMID: 31595562.
- Xie Y, Hou W, Song X, et al. Ferroptosis: process and function. Cell Death Differ. 2016;23(3):369–379. PubMed PMID: 26794443; PubMed Central PMCID: PMCPMC5072448.
- Bogdan AR, Miyazawa M, Hashimoto K, et al. Regulators of iron homeostasis: new players in metabolism, cell death, and disease. Trends Biochem Sci. 2016;41(3):274–286. PubMed PMID: 26725301; PubMed Central PMCID: PMCPMC4783254.
- Chen PH, Wu J, Ding CC, et al. Kinome screen of ferroptosis reveals a novel role of ATM in regulating iron metabolism. Cell Death Differ. 2020;27(3):1008–1022. PubMed PMID: 31320750; PubMed Central PMCID: PMCPMC7206124.
- Tang W, Dong M, Teng F, et al. TMT-based quantitative proteomics reveals suppression of SLC3A2 and ATP1A3 expression contributes to the inhibitory role of acupuncture on airway inflammation in an OVA-induced mouse asthma model. Biomed Pharmacother. 2021;134:111001. PubMed PMID: 33341053.
- Wu Y, Chen H, Xuan N, et al. Induction of ferroptosis-like cell death of eosinophils exerts synergistic effects with glucocorticoids in allergic airway inflammation. Thorax. 2020;75(11):918–927. PubMed PMID: 32759385.
- Bin S, Xin L, Lin Z, et al. Targeting miR-10a-5p/IL-6R axis for reducing IL-6-induced cartilage cell ferroptosis. Exp Mol Pathol. 2021;118:104570. PubMed PMID: 33166496.
- Martitz J, Becker NP, Renko K, et al. Gene-specific regulation of hepatic selenoprotein expression by interleukin-6. Metallomics. 2015;7(11):1515–1521. PubMed PMID: 26399395.
- Luo H, Zhang R. Icariin enhances cell survival in lipopolysaccharide-induced synoviocytes by suppressing ferroptosis via the Xc-/GPX4 axis. Exp Ther Med. 2021;21(1):72. PubMed PMID: 33365072; PubMed Central PMCID: PMCPMC7716635.
- Esty B, Harb H, Bartnikas LM, et al. Treatment of severe persistent asthma with IL-6 receptor blockade. J Allergy Clin Immunol Pract. 2019;7(5):1639–1642. e4. PubMed PMID: 30885880; PubMed Central PMCID: PMCPMC6511285.
- Dong H, Qiang Z, Chai D, et al. Nrf2 inhibits ferroptosis and protects against acute lung injury due to intestinal ischemia reperfusion via regulating SLC7A11 and HO-1. Aging (Albany NY). 2020;12(13):12943–12959. PubMed PMID: 32601262; PubMed Central PMCID: PMCPMC7377827.
- Park E, Chung SW. ROS-mediated autophagy increases intracellular iron levels and ferroptosis by ferritin and transferrin receptor regulation. Cell Death Dis. 2019;10(11):822. PubMed PMID: 31659150; PubMed Central PMCID: PMCPMC6817894.
- Aguilar Diaz De Leon J, Borges CR. Evaluation of oxidative stress in biological samples using the thiobarbituric acid reactive substances assay. J Vis Exp. 2020;159. PubMed PMID: 32478759. DOI:10.3791/61122.
- Zhang HY, Zhang BW, Zhang ZB, et al. Circular RNA TTBK2 regulates cell proliferation, invasion and ferroptosis via miR-761/ITGB8 axis in glioma. Eur Rev Med Pharmacol Sci. 2020;24(5):2585–2600. PubMed PMID: 32196629.
- Prus E, Fibach E. The labile iron pool in human erythroid cells. Br J Haematol. 2008;142(2):301–307. PubMed PMID: 18503586.
- Zhou X, Wei T, Cox CW, et al. Mast cell chymase impairs bronchial epithelium integrity by degrading cell junction molecules of epithelial cells. Allergy. 2019;74(7):1266–1276. PubMed PMID: 30428129.
- Zhuang J, Cui H, Zhuang L, et al. Bronchial epithelial pyroptosis promotes airway inflammation in a murine model of toluene diisocyanate-induced asthma. Biomed Pharmacother. 2020;125:109925. PubMed PMID: 32014690.
- Zhao J, Dar HH, Deng Y, et al. PEBP1 acts as a rheostat between prosurvival autophagy and ferroptotic death in asthmatic epithelial cells. Proc Natl Acad Sci U S A. 2020;117(25):14376–14385. PubMed PMID: 32513718; PubMed Central PMCID: PMCPMC7321965.
- Ali MK, Kim RY, Brown AC, et al. Crucial role for lung iron level and regulation in the pathogenesis and severity of asthma. Eur Respir J. 2020;55(4):1901340. PubMed PMID: 32184317.
- Wenzel SE, Tyurina YY, Zhao J, et al. PEBP1 wardens ferroptosis by enabling lipoxygenase generation of lipid death signals. Cell. 2017;171(3):628–641. e26. PubMed PMID: 29053969; PubMed Central PMCID: PMCPMC5683852.
- Kishimoto T. IL-6: from its discovery to clinical applications. Int Immunol. 2010;22(5):347–352. PubMed PMID: 20410258.
- Neurath MF, Finotto S. IL-6 signaling in autoimmunity, chronic inflammation and inflammation-associated cancer. Cytokine Growth Factor Rev. 2011;22(2):83–89. PubMed PMID: 21377916.