ABSTRACT
Breast cancer is the leading cause of cancer-related death among women despite the significant improvement in diagnosis and treatment. Tumor-associated neutrophils have been shown to suppress antitumor functions of the host. However, how breast cancer tumor microenvironment influences the phenotype and functions of neutrophils to potentiate T cell immunosuppression is unknown. Herein, neutrophils isolated from peripheral blood of healthy donors were treated with supernatants from breast cancer cell lines or recombinant human CCL20. PD-L1 expression on neutrophils was then evaluated by immunofluorescence and flow cytometry. Neutrophils and Jurkat T cells were cocultured to evaluate the effect of tumor-associated neutrophils on T cell functions. Finally, immunohistochemical staining was performed to evaluate the clinical relevance of neutrophils infiltrating breast tumor tissues. Tumor-derived CCL20 activated and upregulated PD-L1 expression on neutrophils. A significant positive correlation was found between CCL20 and CD66b+ neutrophils in tumor tissues. Through in vitro experiment, tumor-associated neutrophils (TANs) effectively suppressed T cell immunity which was reversed upon PD-L1 blockade.
Moreover, a high density of TANs was associated with short disease free survival in breast cancer patients. Furthermore, receiver operating curve showed that the density of TANs could accurately predict disease-free survival in breast cancer patients. Our findings suggest that targeting TANs via CCL20 immunosuppressive pathway may be a novel therapeutic strategy for breast cancer treatment.
1. Introduction
Breast cancer continues to be the commonly diagnosed and the leading cause of cancer-related death among women [Citation1]. Regardless of the substantial advances made in diagnosis, approximately 5–10% of patients show distant metastasis at the time of diagnosis [Citation2]. Even with the improvements in standard chemotherapy and hormonal therapy, recurrence and metastasis occur, contributing to 90% of breast cancer-related deaths [Citation2,Citation3]. In clinical settings, the prognostic assessment of breast cancer patients is based on a set of widely validated factors such as tumor stage, lymph nodes status, histological grade of differentiation and molecular type [Citation4,Citation5]. However, due to the heterogeneous nature of the disease, patients with similar prognostic features have different clinical outcomes. Hence, a deeper understanding of the pathogenesis of breast cancer is needed as we seek to identify new and effective targets for breast cancer treatment.
A substantial body of evidence has linked the tumor microenvironment in tumor development [Citation6,Citation7]. This supportive role results from the infiltration of the tumor stroma by several immune and inflammatory cells, including neutrophils, macrophages and mast cells [Citation8,Citation9]. Neutrophils are critical immune cells in the host defense mechanism during infections [Citation10]. Nevertheless, accumulating evidence suggests that neutrophils contribute to the pathogenesis of several diseases, including cancers. It has been proven that neutrophils infiltrated into the tumor microenvironment exert antitumor and pro-tumor functions [Citation11–14]. Tumor-associated neutrophils (TANs) participate in tumor progression by promoting tumor growth, invasion, angiogenesis and metastasis [Citation15]. Besides these tumor-promoting mechanisms, TANs have been shown to impair the antitumor immune response by expressing immunosuppressive molecules, such as programmed cell death ligand 1(PD-L1) [Citation16,Citation17]. Despite the increasing knowledge in this area, how the tumor microenvironment influences PD-L1 expression on TANs in breast cancer remains unknown.
CCL20, the sole receptor of CCR6, plays a direct role in the progression of several solid tumors, including breast cancer [Citation18–20]. Recently, studies have shown that CCL20 promotes tumor progression by remodeling the functions and phenotype of immune cells infiltrated into the tumor microenvironment [Citation21,Citation22]. Therefore, we hypothesized that CCL20 might have a biological effect on neutrophils infiltrated into the tumor microenvironment. Herein, we sought to elucidate the interplay between tumor-derived CCL20 and neutrophils, emphasizing how such interactions suppress antitumor immunity of the host via PD-L1+ neutrophils.
2. Materials and methods
2.1 Patients and specimens
Formalin-fixed-paraffin-embedded tissue specimens were obtained from 102 breast cancer patients who underwent surgical operation from 2014 to 2019 at The First Affiliated Hospital of Anhui Medical University. Patients included in this study had not received any preoperative adjuvant therapy.
2.2 Cell cultures and enzyme-linked immunosorbent assay (ELISA)
MDA-MB-231, MCF-7 and T47D cell lines were obtained from Shanghai Cell Bank. MDA-MB-231, MCF-7 and T47D cells were cultured in Leibovitz’s-15 (L-15), Rosewell Park Memorial Institute 1640 medium (RPMI 1640) and Dulbecco’s Modified Eagle Medium (DMEM) respectively at 37°C with 5% CO2 where appropriate [Citation23]. These culture media were supplemented with 10% fetal bovine serum (FBS) and 1% penicillin-streptomycin. Jurkat T cell lines were maintained in RPMI1640 culture medium supplemented with 10% FBS and 1% penicillin-streptomycin at 37°C with 5% CO2.
The concentration of CCL20 in supernatants from MDA-MB-231, MCF-7 and T47D cell lines was determined using ELISA (ABclonal, 86 Cummings Park Dr, Woburn, MA 01801, USA).
2.3 Neutrophil isolation and stimulation
For each experiment, neutrophils were isolated from three healthy male volunteers after obtaining informed consent.
Peripheral blood was drawn into ethylenediaminetetraacetic acid (EDTA) tubes and centrifuged at 250 g for 10 minutes. Once centrifugation was complete, supernatants were discarded, and sodium chloride (NaCl) was added to the blood. Ficoll was drawn into a centrifuge tube and the blood was carefully layered on top of the ficoll. This suspension was centrifuged at 450 g for 25 minutes, after which the supernatants were discarded. Subsequently, red lysing buffer was added to the blood and kept on ice for 10 minutes. The mixture was centrifuged at 1500 rpm for 5 minutes, after which the supernatants were discarded. Once the lysing step was complete, cells were washed with phosphate buffer saline (PBS) and centrifuged at 1500 rpm for 5 minutes.
Neutrophils (2 × 105) were stimulated with recombinant human CCL20 (PEPROTECH Rocky Hill, NJ 08553, USA, Cat No. 300-29A) and supernatants from MDA-MB-231, MCF-7 and T47D. For blockade experiments, CCL20 neutralizing antibody (R&D Systems, Minneapolis, MN, USA, Cat No. AF360-SP) was added to the coculture of neutrophils and supernatants from breast cancer cell lines.
2.4 Flow cytometry assay
Neutrophil survival assay
Neutrophils were stimulated with recombinant human CCL20 and supernatants from breast cancer cell lines for 10 hours. After 10 hours of stimulation, the viability of neutrophils was quantified using Annexin V Apoptosis detection kits (Best Bio).
PD-L1 evaluation
Stimulated neutrophils were washed three times with PBS and stained with 5ul of PD-L1 (eBiocienceTM Cat No. 12-5983-42) on ice for 30 min. PD-L1 was evaluated using the Cytoflex flow cytometry machine and analyzed using CytExpert 2.4.
Neutrophil- T cell coculture system
Jurkat T cells (5 × 106) were labeled in 1 ml of PBS containing 5 µM of carboxyfluorescein succinimidyl ester (CFSE, Biolegend, Cat No. 423801) for 20 minutes and seeded at 200,000 cells in a 24 well plate. Stimulated neutrophils (TANs, 2 × 105) were cocultured with Jurkat cells (2 × 105) in 1 ml of RPMI 1640 containing 2ul phytohemagglutinin-L antibody (eBiocienceTM, Cat No. 00-4977-93). For blockade study, TANs were cocultured with Jurkat T cells in the presence of neutralizing antibody against PD-L1 (D-PPA 1 PD-1/PD-L1 inhibitor, TOCRIS, Minnesota, USA, Cat No. 6515).
Jurkat T cells cocultured with TANs were harvested and stained with anti-human CD3 (BioLegend, Clone HIT3a). For IFN-γ (BioLegend, Clone B27) determination, intracellular cytokine staining was performed by fixation and permeabilization. Following this, the content of CFSE and production of IFN-γ on CD3 cells were evaluated using BD flow cytometry machine.
2.5 Immunofluorescence staining
Immunofluorescence staining was performed following previous report [Citation24]. Neutrophils were smeared onto a poly lysine-treated sterile glass slide. Cells were fixed using 200 µl of 4% paraformaldehyde for 1 hour and then permeabilized with 0.5% Triton X-100 in PBS for 15 minutes. Following this, cells were blocked for 30 minutes by a blocking buffer containing 1% bovine serum albumin (BSA) in PBS. The cells were then incubated with anti-PD-L1 antibody (dilution 1:500, Ventana, Roche, Clone SP142) in PBS containing 1% BSA for overnight at 4°C. Samples were washed three times and incubated with Alexa Fluor-conjugated secondary antibody (dilution 1:500, Abcam) for 1 hour. Finally, samples were incubated with 4,6-diamino-2-phenylindole (DAPI) (Beyotime, Shanghai, China) in the dark for 5 minutes. PD-L1 expression was observed using laser scanning confocal microscope 880.
2.6 Immunohistochemistry (IHC)
Immunohistochemical staining was performed as previously described [Citation25]. Briefly, formalin-fixed-paraffin-embedded tissues were cut into 4 μm sections and incubated on slides. The sections were deparaffinized in xylene and rehydrated in ethanol solution of decreasing concentrations (100%, 95% and 75%). Antigen retrieval was performed by incubating slides in 0.01 mol/L sodium citrate buffer (pH 6.0) for 10 minutes. Slides were incubated with primary antibodies against CCL20 (Proeintech, Cat No. 26527-1-AP), CD3 (Ventana, Roche, Lot F16525), CD66b (BD PharmingenTM, Clone No G10F5), IFN-γ (Bioss Antibodies, Cat No. bs-0481 R) and PD-L1 (Ventana, Roche, Clone SP142) at 4°C for overnight. The slides were then incubated with goat-anti mouse and rabbit secondary antibody for 30 minutes at room temperature. This was followed by the addition of 3, 3ʹ-diaminobenzidine tetrahydrochloride (DAB) for 1–5 minutes in the dark. Slides were incubated with hematoxylin for 2 minutes, and the images were observed using Olympus CX33 microscope.
2.7 IHC evaluation
The densities of CD3 T cells and CD66b+ neutrophils in tumor tissues were assessed as previously reported [Citation26]. Briefly, CD66+ neutrophils and CD3 T cells were counted from 10 random microscopic sites (×400). The average count of the entire 102 sections was used to divide CD66b into high and low groups. CCL20 staining intensity was graded on a scale of 0–3 as follows: 0, absence of staining; 1, weak staining; 2, moderate staining; 3, strong staining. Positive tumor cells were similarly scored on a scale of 0–4 as follows: 0, absence of tumor cells; 1, 1–25% positive tumor cells; 2, 26–50% positive tumor cells; 3, 50–75% positive tumor cells; 4, >75% tumor cells. The IHC scores (0–12) were obtained by multiplying the staining intensity by the percentage scores. Based on previous report [Citation27], PD-L1 expression on immune cells was evaluated as follows: IC3 (≥10%), IC2 (≥5 < 10%), IC1 (≥1% and <5), and IC0 (<1%). The INF-γ expression on immune cells was scored on a scale of 1–10.
2.8 Data analysis
Correlation analyses were performed using Spearman where appropriate. Student’s- t-test was used to analyze the difference between two groups. All data were analyzed using two-tailed test and P < 0.05 was considered statistically significant. All statistical analyses were performed using SPSS 21.0 software (SPSSInc, Chicago, IL) and GraphPad Prism 5.0 software package (GraphPad Software, Inc., San Diego, USA).
3. Results
Soluble factors secreted by tumor cells have been shown to activate neutrophils infiltrating the tumor microenvironment. Therefore, we hypothesize that CCL20 secreted by breast cancer cells can promote immunosuppressive functions of neutrophils. This study was aimed at assessing how tumor-derived CCL20 activated neutrophils into TANs and how these TANs inhibited T cell immunity through PD-L1 pathway.
3.1 Tumor-derived CCL20 activated and induced PD-L1 expression on neutrophils
To evaluate the functions of tumor-derived CCL20 on neutrophils, we determined the viability and expression of PD-L1 on neutrophils treated with supernatants from breast cancer cell lines and recombinant human CCL20 (rhCCL20). As shown in , the viability of neutrophils treated with rhCCL20 or supernatant from MDA-MB-231 cell line was significantly (P < 0.0001) higher than neutrophils cultured alone. To further confirm the role of tumor-derived CCL20 in the activation of neutrophils, CCL20 neutralizing antibody was added to the coculture of neutrophils and supernatant from MDA-MB-231 cell line. It was observed that the viability of neutrophils was significantly decreased (P < 0.001), suggesting that tumor-derived CCL20 can activate neutrophils infiltrating the tumor microenvironment.
Figure 1. Tumor-derived CCL20 activated and induced PD-L1 expression on neutrophils. (a) Viability of neutrophils treated with recombinant human CCL20 or supernatant from MDA231cell lines. (b) CCL20 expression as determined by ELISA in cell culture supernatant from breast cancer cell lines. (c) The expression of CCL20, CD66b and PD-L1 in breast cancer tissues (Immunohistochemistry, ×400). (d and e) PD-L1 expression on neutrophils cultured with rhCCL20 and MDA-MB-231 supernatant in the presence of CCL20 neutralizing antibody as determined by immunofluorescence and flow cytometry, respectively
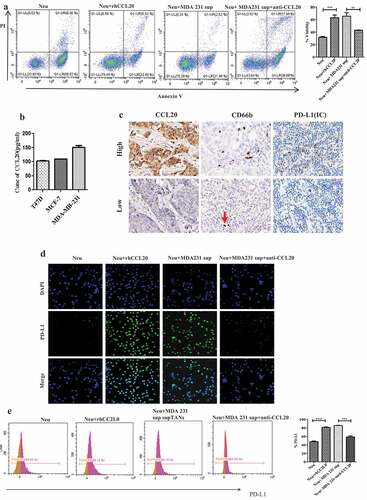
Evidence has shown that tumor-derived factors can induce PD-L1 expression on neutrophils. Hence, we wanted to determine whether tumor-derived CCL20 can induce PD-L1 expression on neutrophils in breast cancer. The levels of CCL20 in the cell culture supernatants from MDA-MB-231, MCF-7 and T47D cell lines () were evaluated. Due to the presence of CCL20 in these supernatants, neutrophils were cocultured with these supernatants for PD-L1 evaluation. Immunofluorescence results showed a higher expression of PD-L1 on neutrophils treated with rhCCL20 or supernatants from MDA-MB-231 (), MCF-7 (Fig S1) and T47D (Fig S1) cell lines than neutrophils cultured alone. However, addition of CCL20 neutralizing antibody to the coculture of neutrophils and supernatants from these cell lines downregulated PD-L1 expression on neutrophils. Flow cytometry results confirmed a significantly (P < 0.001) higher expression of PD-L1 on neutrophils treated with rhCCL20 or supernatants from (MDA-MB-231, ), (MCF-7 Fig S2) and (T47D Fig S2) () than neutrophils cultured alone. However, addition of CCL20 neutralizing antibody to the coculture of neutrophils and supernatants from these cell lines significantly (P < 0.001) downregulated PD-L1 expression on neutrophils.
To establish a connection between CCL20, TANs and PD-L1, immunohistochemistry was performed to evaluate the expression of these markers in 102 breast cancer tumor samples. The analysis showed that the expression of CCL20 was positively correlated to the density of CD66b+ neutrophils (, r = 0.594, P < 0.001) and PD-L1 expression on immune cells (, r = 0.409, P < 0.001). Further, the density of CD66b+ neutrophils showed a positive correlation to PD-L1 expression on immune cells (, r = 0.406, P < 0.001). This suggested a possible relationship between these three markers in breast cancer tumor tissues.
Collectively, these results indicated that tumor-derived CCL20 activated and induced PD-L1 expression on neutrophils.
3.2 TANs promote T cell immunosuppression through PD-L1 in breast cancer
TANs have been demonstrated to promote immunosuppression via PD-L1 in several cancer types. Our next objective was to evaluate the effect of TANs on T cell functions. Jurkat T cells were cocultured with neutrophils treated with supernatant from breast cancer cell lines. Interestingly, TANs significantly suppressed proliferation (, P = 0.0057) and production of IFN-γ (, P = 0.0256) on CD3 T cells. To determine the involvement of PD-L1+ neutrophils in T cell immunosuppression, PD-L1 inhibitor (D-PPA 1) was added to the coculture of TANs and Jurkat T cells. Notably, the blockade of PD-L1 significantly restored both proliferation (P = 0.0037) and production of IFN-γ (P = 0.0229) on CD3 T cells. Collectively, these findings indicated that TANs but not neutrophils might promote T cell immunosuppression through PD-L1 in breast cancer.
Figure 2. TANs promote immunosuppression via PD-L1 in breast cancer. (a) Proliferation of CFSE-labeled T cells co-cultured with neutrophils (Neu) and TANs as examined by flow cytometry. (b) The production of IFN-γ from T cells co-cultured with Neu and TANs, as examined by flow cytometry. (c) The expression of PD-L1, CD3 and IFN-γ in breast cancer tissues (Immunohistochemistry, ×400)
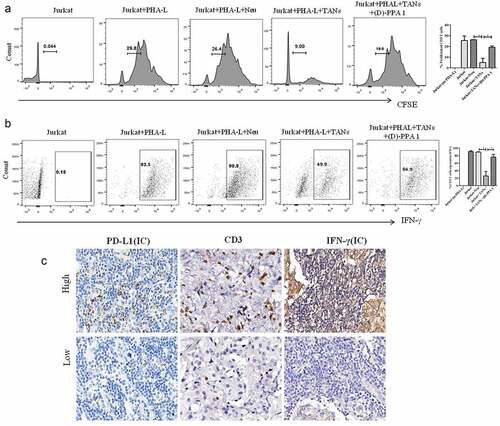
We also performed immunohistochemical staining to evaluate the relationship between CD3, IFN-γ and PD-L1 expression on immune cells (ICs). PD-L1 (ICs) correlated negatively to the density of CD3 T cells (r = −0.315, P = 0.037) and the expression of IFN-γ (ICs) (r = −0.407, P = 0.008) (). Also, the density of CD66b+ neutrophils showed a negative correlation to CD3 T cells (r = −0.427, P < 0.001) and IFN-γ (ICs) (r = −0.451, P < 0.001).
Figure 3. CD66b+ neutrophils predict poor survival in breast cancer patients. (a) High density of CD66b predicts short disease-free survival in breast cancer patients. (b) ROC curve of the predictive value of CD66b . (c) Uni-Cox regression analyses of makers . (d) Multi-Cox regression analyses of markers
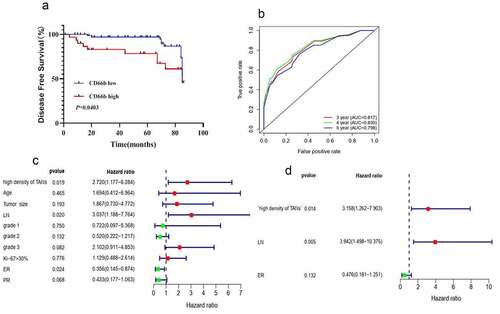
3.3 CD66b+ neutrophils predict poor survival in breast cancer patients
Given the ability of TANs to promote T cell immunosuppression, we evaluated the clinical relevance of TANs in breast cancer. Survival analysis showed that high density of TANs was associated with short disease-free survival in breast cancer patients (). From the receiver operating curve (ROC), the density of TANs accurately predicted the disease-free survival for 3–5 years (). Univariate () and multivariate () Cox regression analyses revealed that the density of TANs was an independent prognostic factor for disease-free survival in breast cancer patients. Unsurprisingly, a high density of TANs was observed in triple-negative breast cancer patients than the other subtypes of breast cancer (S3).
4. Discussion
Neutrophils, the first respondents to tissues damage, have been demonstrated to play crucial roles in several solid tumors. Emerging evidence has documented both protumor [Citation28,Citation29] and antitumor [Citation12,Citation30] functions of neutrophils, highlighting our lack of detailed knowledge about their diversity and functions in the tumor microenvironment. It has been suggested that the plasticity in phenotype and function of neutrophils is mediated by the distinct signals in the tumor microenvironment [Citation10,Citation31]. Therefore, deciphering the exact role of TANs would be particularly useful in the development of TANs targeted therapy. In this research, we showed for the first time how tumor-derived CCL20 induces immunosuppressive neutrophils to inhibit T cell immunity in breast cancer.
The predictive value of neutrophils infiltrating the tumor microenvironment has been reported in several cancer types. The findings from this present demonstrated that high density of CD66b+ neutrophils is not only associated with short disease-free survival but can be used as a marker to predict breast cancer progression. Consistent with our findings, a high density of CD66b+ neutrophils has been reported to be associated with poor prognostic outcomes in germ [Citation26] colorectal [Citation32] and cervical [Citation33] cancer patients.
PD-L1 expression on neutrophils is regulated by several factors such as inflammation, infections and cancers [Citation34]. In cancers, studies have reported that tumor-derived factors such as granulocyte-macrophage colony-stimulating factor (GM-CSF) [Citation16], interleukin 6 (IL-6) [Citation35], long non-coding RNA HOXA transcript at the distal tip (HOTTIP) [Citation17] and high-mobility group box-1 (HMGB1) [Citation36] can induce PD-L1 expression on neutrophils. Herein, our in vitro study proved that both recombinant human CCL20 and tumor-derived CCL20 can induce PD-L1 expression on neutrophils, thus revealing tumor-derived CCL20 as a regulator of PD-L1 expression. Furthermore, immunohistochemical analysis revealed a positive correlation between CCL20 and CD66b+ neutrophils in tumor tissues, which further supports a possible interaction between CCL20 and TANs in tumor microenvironment.
Mechanisms leading to suppression of host antitumor immunity are widely known to be a hallmark of cancer. It has been suggested that the interaction between PD-L1 and PD-1 is a key mechanism responsible for immunosuppression in cancers. In principle, the binding of PD-L1 to PD-1 effectively suppresses the proliferation and activation of T cells [Citation37,Citation38]. This study revealed that PD-L1 expression on TANs effectively inhibited proliferation and IFN-γ production on T cells. This finding is consistent with other reports in gastric and hepatocellular cancers where TANs displayed a remarkable ability to suppress T cell immune response via PD-L1 expression [Citation16,Citation35,Citation39]. Taken together, our report suggests that the activation of neutrophils by tumor-derived CCL20 is necessary to suppress and impair the antitumor immunity. This reveals new insight into the role of CCL20 in breast cancer pathogenesis.
Furthermore, PD-L1 expression on immune cells (ICs) correlated negatively with the density of CD3 T cells and IFN-γ (ICs), which further supports the role of PD-L1 in tumor related-immunosuppression. Concordance with these findings, PD-L1 expression on tumor-infiltrating immune cells has been noted to play a key role in suppressing the antitumor immunity independent of PD-L1 expression on tumor cells. More importantly, PD-L1 expression on immune cells was an indicator of immunosuppression in tumors [Citation40].
5. Conclusion
Based on our findings, we propose a model involving T cell immunosuppression in breast cancer (). First, CCL20 secreted by breast cancer cells activates and induces PD-L1 expression on neutrophils. Second, these activated neutrophils exert protumor functions by suppressing T cell functions via PD-L1. Hence, targeting TANs via CCL20 may be a novel therapeutic strategy for breast cancer treatment. Despite the novelty of our findings, further in vivo studies will be required to explain the underlying mechanism by which CCL20-TANs-PD-L1 network influences immunosuppression in breast cancer.
Author contributions
Q Wu and Louis BK designed the experiments. Louis BK performed the experiments. Louis BK and SJ Wang compiled and analyzed the data. SJ Wang, ZY Cai, YJ Sheng, WD Peng, WJ Zhang, H Xiao, X Wang were involved in some experiments. Louis BK wrote the manuscript. Q Wu funded the work, revised the manuscript and provided overall research supervision.
Ethical approval
Written informed consent was obtained from patients before they were enrolled in the study. All specimens and protocols used in this study were approved by the Ethics Committee of Anhui Medical University (20180096, 20200976). The protocols used were under the ethical standards of the Biomedical Ethics Committee of Anhui Medical University and the 1964 Helsinki Declaration.
Research highlights
Tumor-derived CCL20 activated and induced PD-L1 expression on neutrophils.
Tumor-associated neutrophils promoted T cell immunosuppression via PD-L1 in breast cancer.
High density of tumor-associated neutrophils was associated with short disease-free survival in breast cancer patients.
Supplemental Material
Download ()Acknowledgment
We acknowledge the funding support from The Academic and Technological Leaders and Reserves Foundation (2019H230) and China Scholarship Council (2020-1417). We also acknowledge the support offered by the Center for Scientific Research of Anhui Medical University. We thank Mr Jiegou Xu and his research group for providing Jurkat T cell lines. We are grateful to Mrs Meijuan Zheng from the Department of Clinical Laboratory of The First Affiliated Hospital of Anhui Medical University for assisting in flow cytometry. We acknowledge the help offered by Mingfeng Li and Xiaojuan Ding.
Disclosure statement
Authors declare that there is no competing interest.
Supplementary material
Supplemental data for this article can be accessed here
Correction Statement
This article has been republished with minor changes. These changes do not impact the academic content of the article.
Additional information
Funding
References
- Azamjah N, Soltan-Zadeh Y, Zayeri F. Global trend of breast cancer mortality rate: a 25-year study. Asian Pac J Cancer Prev. 2019;20(7):2015–2020.
- Itani N, Grogan N, Mott S, et al. Metastatic presentations of previously treated early-stage breast cancer patients and association with survival. Clin Breast Cancer. 2020;20(3):209–214.
- Skuli SJ, Sheng JY, Bantug ET, et al. Survivorship care visits in a high-risk population of breast cancer survivors. Breast Cancer Res Treat. 2019;173(3):701–708.
- Susini T, Saccardin G, Renda I, et al. Immunohistochemical evaluation of FGD3 expression: a new strong prognostic factor in invasive breast cancer. Cancers (Basel). 2021;13(15):3824.
- Ding L, Zhang Z, Xu Y, et al. Comparative study of Her-2, p53, Ki-67 expression and clinicopathological characteristics of breast cancer in a cohort of northern China female patients. Bioengineered. 2017;8(4):383–392.
- Tan S, Xia L, Yi P, et al. Exosomal miRNAs in tumor microenvironment. J Exp Clin Cancer Res. 2020;39(1):67.
- Roma-Rodrigues C, Mendes R, Baptista PV, et al. Targeting tumor microenvironment for cancer therapy. Int J Mol Sci. 2019;20(4):840.
- Quail DF, Joyce JA. Microenvironmental regulation of tumor progression and metastasis. Nat Med. 2013;19(11):1423–1437.
- Arneth B. Tumor microenvironment. Medicina (Kaunas). 2019;56(1):15.
- Wu L, Saxena S, Awaji M, et al. Tumor-associated neutrophils in cancer: going pro. Cancers (Basel). 2019;11(4):564.
- Germann M, Zangger N, Sauvain MO, et al. Neutrophils suppress tumor-infiltrating T cells in colon cancer via matrix metalloproteinase-mediated activation of TGFβ. EMBO Mol Med. 2020;12(1):e10681.
- Sun B, Qin W, Song M, et al. Neutrophil suppresses tumor cell proliferation via fas /fas ligand pathway mediated cell cycle arrested. Int J Biol Sci. 2018;14(14):2103–2113.
- Eruslanov EB, Bhojnagarwala PS, Quatromoni JG, et al. Tumor-associated neutrophils stimulate T cell responses in early-stage human lung cancer. J Clin Invest. 2014;124(12):5466–5480.
- Cai Z, Zhang M, Boafo Kwantwi L, Bi X, Zhang C, Cheng Z, Ding X, Su T, Wang H, Wu Q. Breast cancer cells promote self-migration by secreting interleukin 8 to induce NET formation. Gene. 2020;754:144902.
- Hajizadeh F, Aghebati Maleki L, Alexander M, et al. Tumor-associated neutrophils as new players in immunosuppressive process of the tumor microenvironment in breast cancer. Life Sci. 2021;264:118699.
- Wang T-T, Zhao Y-L, Peng L-S, et al. Tumour-activated neutrophils in gastric cancer foster immune suppression and disease progression through GM-CSF-PD-L1 pathway. Gut. 2017;66(11):1900.
- Shang A, Wang W, Gu C, et al. Long non-coding RNA HOTTIP enhances IL-6 expression to potentiate immune escape of ovarian cancer cells by upregulating the expression of PD-L1 in neutrophils. J Exp Clin Cancer Res. 2019;38(1):411.
- Hippe A, Braun SA, Oláh P, et al. EGFR/Ras-induced CCL20 production modulates the tumour microenvironment. Br J Cancer. 2020;123(6):942–954.
- Kadomoto S, Izumi K, Hiratsuka K, et al. Tumor-associated macrophages induce migration of renal cell carcinoma cells via Activation of the CCL20-CCR6 axis. Cancers (Basel). 2020;12(1):89.
- Li Y, Liu J, Xiao Q, et al. EN2 as an oncogene promotes tumor progression via regulating CCL20 in colorectal cancer. Cell Death Dis. 2020;11(7):604.
- Geismann C, Grohmann F, Dreher A, et al. Role of CCL20 mediated immune cell recruitment in NF-κB mediated TRAIL resistance of pancreatic cancer. Biochim Biophys Acta, Mol Cell Res. 2017;1864(5):782–796.
- Wu -H-H, Hwang-Verslues WW, Lee W-H, et al. Targeting IL-17B–IL-17RB signaling with an anti–IL-17RB antibody blocks pancreatic cancer metastasis by silencing multiple chemokines. J Exp Med. 2015;212(3):333–349.
- Yang B, Wang F, Zheng G. Transmembrane protein TMEM119 facilitates the stemness of breast cancer cells by activating Wnt/β-catenin pathway. Bioengineered. 2021;12(1):4856–4867.
- Lei L, Tang L. Schwann cells genetically modified to express S100A4 increases GAP43 expression in spiral ganglion neurons in vitro. Bioengineered. 2017;8(4):404–410.
- Shang D, Liu Y, Zhang J, et al. Peroxisome proliferator-activated receptor γ (PPARγ) suppresses the proliferation and metastasis of patients with urothelial carcinoma after renal transplantation by inhibiting LEF1/β-catenin signaling. Bioengineered. 2020;11(1):1350–1367.
- Yamada Y, Nakagawa T, Sugihara T, et al. Prognostic value of CD66b positive tumor-infiltrating neutrophils in testicular germ cell tumor. BMC Cancer. 2016;16(1):898.
- Emens LA, Cruz C, Eder JP, et al. Long-term clinical outcomes and biomarker analyses of atezolizumab therapy for patients with metastatic triple-negative breast cancer: a phase 1 study. JAMA Oncol. 2019;5(1):74–82.
- Li S, Cong X, Gao H, et al. Tumor-associated neutrophils induce EMT by IL-17a to promote migration and invasion in gastric cancer cells. J Exp Clin Cancer Res. 2019;38(1):6.
- Lianyuan T, Gang L, Ming T, et al. Tumor associated neutrophils promote the metastasis of pancreatic ductal adenocarcinoma. Cancer Biol Ther. 2020;21(10):937–945.
- Comen E, Wojnarowicz P, Seshan VE, et al. TNF is a key cytokine mediating neutrophil cytotoxic activity in breast cancer patients. Npj Breast Cancer. 2016;2(1):16009.
- Li L, Yang C, Zhao Z, et al. Skewed T-helper (Th)1/2- and Th17/T regulatory‑cell balances in patients with renal cell carcinoma. Mol Med Rep. 2015;11(2):947–953.
- Rao HL, Chen JW, Li M, et al. Increased intratumoral neutrophil in colorectal carcinomas correlates closely with malignant phenotype and predicts patients’ adverse prognosis. PloS One. 2012;7(1):e30806.
- Carus A, Ladekarl M, Hager H, et al. Tumour-associated CD66b+ neutrophil count is an independent prognostic factor for recurrence in localised cervical cancer. Br J Cancer. 2013;108(10):2116–2122.
- Bowers NL, Helton ES, Huijbregts RPH, et al. Immune suppression by neutrophils in HIV-1 infection: role of PD-L1/PD-1 pathway. PLoS Pathog. 2014;10(3):e1003993. e1003993-e1003993.
- Cheng Y, Li H, Deng Y, et al. Cancer-associated fibroblasts induce PDL1+ neutrophils through the IL6-STAT3 pathway that foster immune suppression in hepatocellular carcinoma. Cell Death Dis. 2018;9(4):422.
- Shi Y, Zhang J, Mao Z, et al. Extracellular vesicles from gastric cancer cells induce pd-l1 expression on neutrophils to suppress t-cell immunity. Front Oncol. 2020;10:629.
- Sun R, Xiong Y, Liu H, et al. Tumor-associated neutrophils suppress antitumor immunity of NK cells through the PD-L1/PD-1 axis. Transl Oncol. 2020;13(10):100825.
- Zheng Y, Fang YC, Li J. PD‑L1 expression levels on tumor cells affect their immunosuppressive activity. Oncol Lett. 2019;18(5):5399–5407.
- He G, Zhang H, Zhou J, et al. Peritumoural neutrophils negatively regulate adaptive immunity via the PD-L1/PD-1 signalling pathway in hepatocellular carcinoma. J Exp Clin Cancer Res. 2015;34(1):141.
- Kowanetz M, Zou W, Gettinger SN, et al. Differential regulation of PD-L1 expression by immune and tumor cells in NSCLC and the response to treatment with atezolizumab (anti-PD-L1). Proc Natl Acad Sci U S A. 2018;115(43):E10119–E10126. E10119-E10126.