ABSTRACT
Long non-coding RNA (lncRNA)–microRNA–mRNA signaling axes have recently been shown to have a key role in the development of breast cancer (BC). In this study, we investigated how the cancer susceptibility candidate 9 (CASC9) gene affects the cell growth, invasion, migration, and apoptosis of BC cells. The levels of microRNA-590-3p (miR-590-3p), CASC9, and the sine oculis homeobox 1 (SIX1) gene were determined through qRT-PCR. We conducted cell counting kit-8 (CCK-8) assays to assess cell proliferation, transwell assays to detect cell migration/invasion, and flow cytometry to evaluate cell apoptosis. StarBase v2.0 was used to predict interactions between miR-590-3p and SIX1 or CASC9, and dual-luciferase reporter assays were used to verify these predictions. CASC9 protein was overexpressed in BC cells and tissues, while CASC9 knockdown inhibited BC cell growth, invasion, and migration and promoted apoptosis. Additionally, we verified that CASC9 competes for binding with miR-590-3p. Moreover, SIX1 was determined to be a target of miR-590–3p, and SIX1 expression was inhibited by miR-590-3p overexpression. CASC9 enhanced BC development by downregulating miR-590-3p and upregulating SIX1 during the activation of the NF-κB pathway. These data suggest that the CASC9/miR-590-3p/SIX1/NF-κB axis is involved in breast cancer progression, providing insight into the function of CASC9 in breast cancer development.
1. Introduction
Breast cancer (BC) is a common malignancy with high incidence in women [Citation1]. Recurrence and metastasis after treatment pose severe threats to survival [Citation2]. BC therapeutics mainly include surgery, chemotherapy, and radiation, but the mechanisms underlying BC development remain unclear [Citation3]. Gene therapy has recently become a hot topic in the fields of cancer diagnosis and treatment. The characterization of tumor suppressor genes and oncogenic genes and their involvement in BC has helped improve the diagnosis and treatment of BC.
Long non-coding RNAs (lncRNAs) are RNAs with a length of over 200 nucleotides that do not encode functional proteins [Citation4]. Several lncRNAs have been reported to participate in tumorigenesis and cancer progression. H19, the first lncRNA discovered, is abnormally expressed in various cancers including BC [Citation5–9]. H19, the Let-7 microRNA (miRNA), and LIN28 form a double-negative feedback loop, which plays a key role in enhancing BC cell growth and differentiation [Citation10]. In addition, NEAT1 is positively correlated with breast cancer stage, such that low NEAT1 levels predict good prognosis whereas high NEAT1 levels predict poor prognosis [Citation11]. The ANCR lncRNA is expressed at low levels in BC cells, which effectively inhibits tumorigenesis and distant metastasis [Citation12]. CDKN2B-AS1 promotes the progression of breast cancer through the miR-122-5p/STK39 axis [Citation13]. CASC9 was reported to promote malignancy in ovarian cancer, colorectal cancer (CRC), lung cancer, esophageal squamous cell carcinoma (ESCC), and BC [Citation14–18]. Although the role of CASC9 in BC development has been studied, the underlying molecular mechanisms have not been fully elucidated.
lncRNAs have been suggested to act as competing endogenous RNAs (ceRNAs) that sponge miRNAs [Citation19]. CASC9 has been reported to act as a ceRNA to target the tumor suppressor miR-125a-3p and regulate neuregulin-1 (NRG1) to promote hemangioma endothelial cell invasion and migration [Citation20]. Moreover, CASC9 positively regulates checkpoint kinase 1 (CHK1) by sponging the miR-195/497 cluster to promote BC cell growth [Citation21]. miR-590 has been identified as a tumor suppressor in different cancers including BC [Citation22–26]. We previously identified miR-590-3p as a downstream target of CASC9 in relation to BC [Citation27].
SIX1 has been identified as an oncogene in various cancers [Citation28–30]. In BC, SIX1 induces the epithelial-mesenchymal transition (EMT) [Citation31]. In addition, SIX1 was found to upregulate vascular endothelial growth factor-C (VEGF-C) expression to induce lymphangiogenesis and metastasis in BC mouse models [Citation32]. Recent data suggest that several miRNAs including miR-362, miR-186-5p, and miR-188 target the 3ʹ untranslated regions (3ʹ UTRs) of the SIX1 mRNA to induce its degradation and inhibit its translation in several cancers [Citation33–35]. Through bioinformatic analysis, we discovered putative miR-590-3p binding sites in the SIX1 3ʹ UTR. Considering that miR-590-3p is a downstream target of CASC9, we reasoned that miR-590–3p might represent the ‘bridge’ for CASC9 and SIX1 in BC. Furthermore, SIX1 overexpression has been previously suggested to activate the NF-κB pathway [Citation36]. Thus, CASC9 promotes BC progression by regulating miR-590-3p and modulating the SIX1/NF-κB axis. The present study explored CASC9 expression in BC cells and tissues, while exploring its roles in BC cell growth, invasion, migration, and apoptosis, and the underlying mechanisms. We hypothesized that the CASC9 lncRNA affects BC cell proliferation, migration, and invasion via the miR-590–3p/SIX1 axis.
2. Materials and methods
2.1 Patients and sample collection
From January 2018 to December 2019, 42 tissue specimens were collected from patients with BC who underwent BC surgery at Shangqiu Central Hospital (Henan, China). All samples were pathologically confirmed to be without preoperative radiotherapy, chemotherapy, or any other treatment. The tissue samples were immersed in liquid nitrogen after removal. Our experimental procedures were approved by the Research Ethics Committee of Shangqiu Central Hospital. This study was performed in accordance with the Declaration of Helsinki. The participants provided informed consent for participation.
2.2 Cell culture
Normal breast epithelial cells (MCF-10A) and BC cells (MDA-MB-468, MCF7, and MDA-MB-231) were provided by the BeNa Culture Collection (Beijing, China) and cultured in high-glucose (HG) DMEM containing 10% fetal bovine serum (FBS). MDA-MB231 and MCF7 cells were cultivated in RPMI 1640 medium containing 10% FBS. Each sample was incubated at 37°C under 5% CO2 conditions [Citation37].
2.3 Cell line transfection
si-CASC9, miR-590–3p mimics, and corresponding negative controls (NCs) were obtained from GenePharma (Shanghai, China). Cells (6 × 105/well) were seeded into 6-well plates and the above plasmids, a pcDNA3.1 empty vector, and a pcDNA3.1-SIX1 vector were separately transfected into MDA-MB231 and MCF7 cells using Lipofectamine 2000 Reagent (Invitrogen, Carlsbad, CA, USA). following previously established protocols [Citation27].
2.4 Quantitative real-time PCR (qRT-PCR)
Total RNA was isolated using TRIzol™ Reagent (Invitrogen, Carlsbad, CA, USA) and quantified. The PrimeScript™ RT Kit (Takara, Dalian,China) was used to reverse transcribe 1 µg of RNA into cDNA. SYBR Green PCR Master Mix Kit (Invitrogen, Thermo Fisher Scientific, Inc.; cat. no. 4,309,155) was used in qRT-PCR reactions run on the ABI 7500HT Real-Time PCR machine (Takara Bio, Shiga, Japan) according to established protocols [Citation27]. The thermocycling conditions were as follows: 10 min at 94°C followed by 40 cycles of 30 s at 94°C, 34 s at 54°C, and 30 s at 72°C. The 2−ΔΔCt approach was utilized to calculate relative transcript levels, with U6 and GAPDH serving as reference genes for miR-590-3p and CASC9/SIX1, respectively. The sequences of all primers used in this study are presented in .
Table 1. The primer sequences
2.5 Cell Counting Kit-8 (CCK-8) assay
A CCK-8 assay was conducted to measure cell proliferation. In brief, after digestion, transfected MCF7 and MDA-MB231 cells (3 × 105/well) were inoculated into 96-well plates and incubated under 5% CO2 at 37°C for 1, 2, 3, 4, and 5 days. After adding 10 µL CCK-8 solution (Dojindo Chemical, Japan), the cells were cultured for 2 h. Finally, the optical density (OD) was determined at 490 nm and the absorbance was measured in triplicate [Citation38].
2.6 Cell migration and invasion assay
Transfected cells (1.0 × 106) were prepared in a cell suspension and added to the top of a Transwell chamber filled with serum-free medium (1 mL), while the bottom chamber was filled with 10% FBS in 600 µL DMEM (Sangon Biotech Co., Ltd.). After 24 h, cells in the top chamber were collected and subjected to 95% ethanol fixation, 15 min of 0.05% crystal violet staining, and observation and enumeration using an optical microscope (Nikon, Tokyo, Japan) at 100× magnification. For the invasion assays, transwell chambers were coated with Matrigel (Millipore, USA) [Citation38].
2.7 Western blotting (WB)
ter 24 h of transfection, MCF7 and MDA-MB231 cells were collected and lysed in RIPA buffer to isolate total protein, which was quantified using a BCA assay (Beyotime Biotechnology, Shanghai, China). Next, total protein samples (30 µg) were separated on a 10% SDS-PAGE gel, followed by the transfer of the proteins to PVDF membranes (Beyotime Biotechnology, Shanghai, China). Membranes were incubated with primary antibodies overnight at 4°C, followed by incubation with secondary antibodies for 2 h at room temperature. Membranes were washed with TBST, developed using ECL and a gel imaging system (Bio-Rad) and assessed by One software (Bio-Rad, USA) [Citation38].
2.8 Luciferase reporter assay
The pmirGLO Dual-Luciferase Reporter Gene Detection System (Promega, Madison, WI, USA) was used to synthesize SIX1- and CASC9-binding sites for miR-590-3p in the corresponding 3ʹ UTR. These constructs were inserted into the pmirGLO luciferase expression vector to generate a pmirGLO/CASC9 vector and a pmirGLO/SIX1-UTR vector. The mutant sequence was synthesized to generate the pmirGLO/CASC9-M and pmirGLO/SIX1-M-UTR vectors using the same method. We used Lipofectamine 2000 (Invitrogen, Thermo Fisher Scientific, Inc.) to transfect the pmirGLO/CASC9 and pmirGLO/SIX1-UTR wild-type vectors, pmirGLO/CASC9-M, pmirGLO/SIX1-M-UTR vectors, and miR-590–3p mimics, together with the corresponding NC, into MCF7 and MDA-MB231. The cells were harvested 48 h after transfection. The fluorescence value of the sea kidney served as the endogenous control, as suggested by the manufacturer. The fluorescence ratio (firefly to sea kidney) was determined to evaluate the relative activity of the reporter gene [Citation27].
2.9 Construction of the pCDH-Flag-SIX1 recombinant plasmid
SIX1 was amplified for 34 cycles (5 min of pre-denaturation at 95°C, 30 s of denaturation at 95°C, 30 s of annealing at 62°C, 60 s of extension at 72°C, and 7 min of extension at 72°C). PCR products were visualized by gel electrophoresis, and Not I and BamHI were used to digest the amplicons and the pCDH-Flag vector. T4 DNA ligase was used to ligate the amplicons into the pCDH-flag vector at 16°C for 30 minutes. The resulting vectors were transformed into Escherichia coli DH5α cells and cultured on LB plates containing 100 μg/ml ampicillin [Citation39].
2.10 Flow cytometry
Transfected cells were digested using trypsin, centrifuged, and harvested. Cells were rinsed twice with PBS, resuspended in 70% ethanol, and fixed at 4°C for 30 min. Next, the fixed cells were washed twice with PBS and incubated in AnnexinV-FITC and propidium iodide (PI, Jiancheng, Nanjing, China) for 1 h at 4°C, at which point quantifications were performed [Citation40].
2.11 Bioinformatic analysis
We searched the GEPIA database (http://gepia.cancer- pku.cn/) to analyze SIX1 expression in BC samples [Citation41]. The LncBase Predicted v.2 (http://carolina.imis.athena-innovation.gr/diana_tools/web/index.php?r=lncbasev2%2Findex-predicted) [Citation42] and TargetScan 7.2 databases (http://www.targetscan.org/vert_72/) [Citation43] were used to search for miR-590-3p binding sites in CASC9 and the SIX1 3ʹ UTR. Regarding the search for miR-590-3p binding sites, the LncBase Predicted v.2 database was used to input the location of CASC9 (chr8:75,120,409–75,352,327) and then identify binding sites for miRNAs, including miR-590-3p.
2.12 Statistical analysis
SPSS24.0 software was used to perform all statistical analyses. Data are displayed as the mean ± standard deviation (SD). Significant differences between groups were identified using Student’s t-tests or ANOVA followed by Tukey’s post-hoc test. Correlations were determined using Pearson’s correlation analysis. Statistical significance was set at p< 0.05.
3. Results
In this study, we explored the role of CASC9 in BC. A series of assays led to the discovery that CASC9 promotes BC progression by regulating the miR-590-3p/SIX1/NF-κB axis. Our findings highlight the functional roles of CASC9 in BC, providing new insights into BC pathogenesis.
3.1 CASC9 is upregulated in BC tissues and cells
We first interrogated CASC9 expression in 42 pairs of BC and non-carcinoma tissues by qRT-PCR. CASC9 levels were significantly increased in BC samples compared to the levels in control samples (). CASC9 levels were examined in normal breast epithelial MCF-10A cells and BC cells (MDA-MB-231, MDA-MB-468, and MCF7) by qRT-PCR. CASC9 was found to be highly expressed in MDA-MB231, MCF7, and MDA-MB-468 cell lines (), suggesting that CASC9 is closely related to BC progression. CASC9 expression was the highest in the MCF7 and MDA-MB231 cell lines, therefore these cell lines were selected for the subsequent experiments.
3.2 CASC9 knockdown suppresses BC cell proliferation and promotes apoptosis
siRNA-CASC9 was used to downregulate CASC9, and qRT-PCR was used to confirm that CASC9 expression was lower in the siRNA-CASC91# group than in the siRNA-CASC92# group (). Therefore, siRNA-CASC91# was used for further experiments. CCK8 and flow cytometry assays were used to investigate the function of CASC9 in BC development. CASC9 knockdown significantly reduced BC cell proliferation ( and c) and increased apoptosis in MCF7 and MDA-MB231 cells (). These results suggest that CASC9 promotes BC cell proliferation and inhibits cell apoptosis.
Figure 2. Knock-down of CASC9 inhibits BC cell growth but promotes their apoptosis
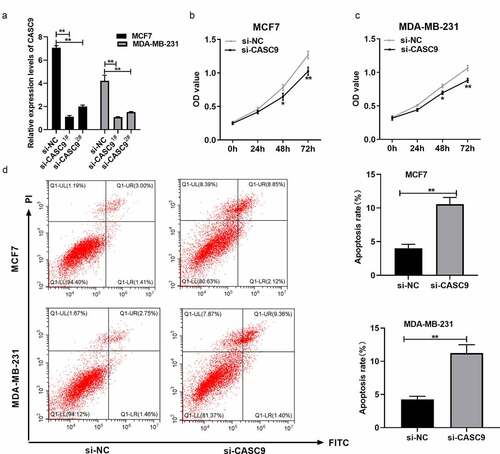
3.3 CASC9 knockdown suppresses BC cell migration and invasion
Transwell migration and invasion experiments revealed that, compared to the NC group, the number of invading and migrating BC cells markedly decreased in response to CASC9 knockdown ( and b), suggesting that CASC9 promotes BC cell migration and invasion.
Figure 3. Knock-down of CASC9 suppresses breast cancer cell invasion and migration
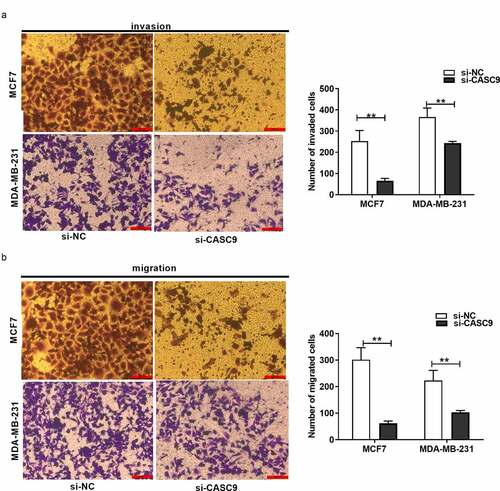
3.4 miR-590-3p is a target of CASC9
To further explore the molecular mechanism underlying the role of CASC9 in the development and progression of BC, putative CASC9-binding sites were predicted using LncBase v.2. Interestingly, bioinformatic analysis revealed that miR-590-3p was a potential target of CASC9 (). A physical interaction between miR-590-3p and CASC9 was observed in MDA-MB231 and MCF7 cells using a luciferase reporter assay ( and c). In addition, we revealed that knockdown of CASC9 markedly upregulated miR-590-3p expression in MCF7 and MDA-MB231 cells relative to the NC (). We also measured miR-590-3p expression in BC tissues and cells, and found that miR-590-3p expression was decreased in BC cells and tissues in a manner that was directly proportional to CASC9 levels in BC tissue samples ().
Figure 4. miR-590-3p as a CASC9 candidate target
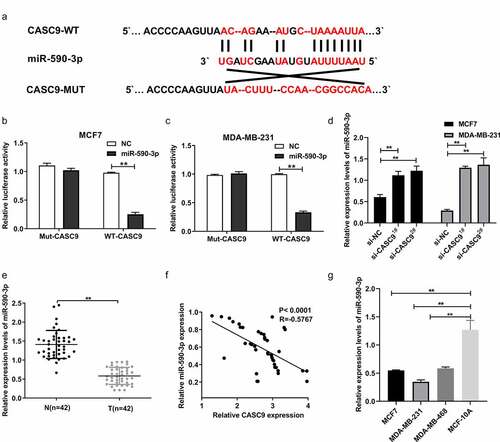
3.5 CASC9 promotes malignancy of BC cells by decreasing miR-590-3p levels
To further explore whether CASC9 promoted malignancy by regulating miR-590-3p in BC, we co-transfected si-CASC9 and miR-590-3p mimics into BC cells. First, miR-590-3p mimics, together with the corresponding NCs, were transfected into MCF7 and MDA-MB231 cells to determine the transfection efficiency. The miR-590-3p mimics group was characterized by remarkably higher miR-590-3p mRNA levels compared to the NC group, demonstrating successful transfection (). In addition, we investigated whether CASC9 promotes the malignancy of breast cancer cells by interacting with miR-590-3p. To verify this hypothesis, we transfected miR-590-3p mimics and si-CASC9 in MCF7 and MDA-MB231 cells. We confirmed that CASC9 knockdown remarkably suppressed cell growth, invasion, and migration, but enhanced apoptosis. However, these effects were enhanced when cells were co-transfected with miR-590-3p mimics (). Based on these findings, CASC9 may promote the malignancy of BC cells by decreasing miR-590-3p.
Figure 5. CASC9 promotes malignant behavior of BC cells through reducing miR-590-3p
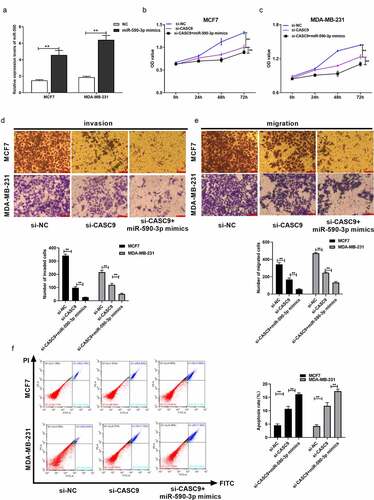
3.6 SIX1 is a direct target of miR-590-3p in BC cells
TargetScan analyses predicted that SIX1 may be a direct target of miR-590-3p (). Luciferase reporter assays verified that miR-590-3p directly interacted with CASC9 in MCF7 and MDA-MB231 cells ( and c). In addition, qRT-PCR assays validated that miR-590-3p overexpression markedly decreased SIX1 expression (). Additionally, SIX1 levels were increased in BC patient tumor samples compared to normal samples, as evidenced by the analysis of The Cancer Genome Atlas database using GEPIA (). SIX1 was highly expressed in BC tissues () in a manner that was indirectly proportional to miR-590-3p expression () and positively correlated with CASC9 levels in BC tissue samples (). These findings suggest that SIX1 is directly targeted by miR-590-3p.
Figure 6. SIX1, a direct miR-590-3p target within BC cells
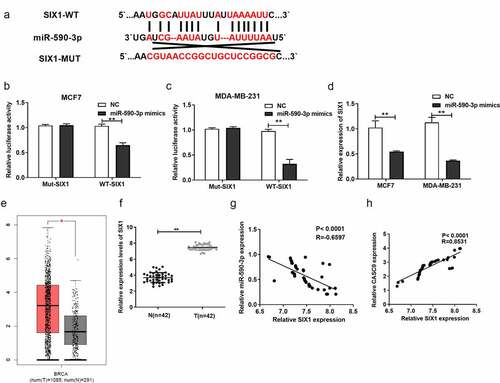
3.7 CASC9 promotes the malignancy of BC cells by decreasing miR-590-3p levels and upregulating SIX1
As previously mentioned, CASC9 binds to miR-590-3p, while miR-590-3p targets SIX1 to exert its function in BC cells. To further explore whether CASC9 promoted the malignancy of BC cells by regulating the miR-590-3p/SIX1 axis in BC, si-SIX1 (si-NC) and pcDNA-SIX1 (pcDNA3.1) were transfected into MCF7 and MDA-MB231 cells. qRT-PCR analysis demonstrated that SIX1 mRNA levels were decreased in response to si-SIX1, while SIX1 mRNA levels increased in response to pcDNA-SIX1, indicating successful transfection ( and b). A series of functional assays demonstrated that transfection of si-CASC9 markedly suppressed BC cell proliferation, migration, and invasion, which markedly increased after miR-590-3p mimic co-transfection, while co-transfection with pcDNA-SIX1 restored these effects (). Moreover, CASC9 knockdown increased BC cell apoptosis, which was markedly increased by co-transfection of a miR-590-3p mimic, an effect that was abolished by SIX1 overexpression (). These observations suggest that CASC9 enhances BC malignancy by decreasing miR-590-3p expression and upregulating SIX1.
Figure 7. CASC9 promotes malignant behavior of BC cells by down-regulating miR-590-3p and up-regulating SIX1
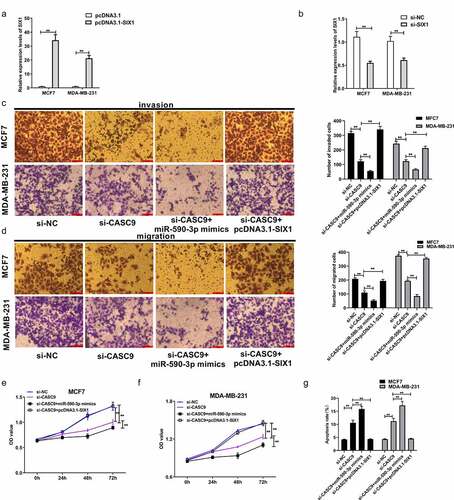
3.8 The CASC9/miR-590-3p/SIX1/NF-κB axis is involved in BC progression
SIX1 silencing was reported to inhibit NF-κB activation [Citation36]. We discovered that CASC9 knockdown decreased the levels of related proteins such as SIX1, p65, matrix metalloproteinase 9 (MMP9), and B-cell lymphoma 2 (BCL2), while miR-590-3p overexpression enhanced these effects and SIX1 overexpression reversed these effects (). These findings confirm that the CASC9/miR-590-3p/SIX1/NF-κB axis is involved in BC progression.
4. Discussion
lncRNAs are endogenous, single-stranded, non-coding RNAs with lengths of over 200 nucleotides [Citation4]. They participate in epigenetic regulation via chromatin remodeling, transcriptional regulation, and post-transcriptional regulation [Citation44]. lncRNAs also function in regulating cell growth, differentiation, neural development, immune homeostasis, tumorigenesis, and other biological processes [Citation45–49]. Several studies have examined the functions of lncRNAs in BC [Citation8,Citation11,Citation13]. For instance, high expression of the VCAN-AS1 lncRNA was detected in BC, which promotes the malignancy of BC by regulating the miR-106a-5p-regulated signal transducer and activator of transcription 3/hypoxia-inducible factor-1alpha pathway [Citation37]. The BCAR4 lncRNA is overexpressed in BC cells and tissues, which promotes BC cell proliferation by suppressing p16 expression [Citation50]. The CASC9 oncogene is highly expressed in esophageal squamous cell carcinoma (ESCC), ovarian cancer, BC, lung cancer, papillary thyroid cancer, and bladder cancer [Citation14–17,Citation51,Citation52]. The CASC9 lncRNA positively regulates CHK1 to promote the growth and survival of BC cells by sponging the miR-195/497 cluster. Zhang et al. showed that CASC9 promotes BC cell metastasis by mediating the miR-215/Twist-related protein 2 signal transduction pathway in BC [Citation53]. Shao et al. reported that CASC9 enhanced the growth and survival of BC cells by sponging the miR-195/497 cluster [Citation21]. However, the effect of CASC9 in BC had not yet been investigated. Understanding its role in BC may shed light on the development and progression of BC.
The present study discovered that the CASC9 lncRNA was highly expressed in BC tissues and cells, indicating a possible regulatory role for CASC9 in BC progression. To explore the role of CASC9 in BC, we silenced CASC9 in MCF7 and MDA-MB231 BC cells. Furthermore, we demonstrated that low expression of CASC9 remarkably suppressed cell growth, migration, and invasion, but enhanced apoptosis.
lncRNAs can act as molecular sponges by binding to miRNAs to inhibit the miRNA-mediated silencing of target mRNAs. TargetScan analysis indicated that miR-590-3p might be a binding partner of CASC9. Bioinformatic predictions and dual-luciferase reporter assays revealed that SIX1 is a target of miR-590-3p. Thus, the upregulation of SIX1 in BC tissues was confirmed. Thus, in this study, we characterized the CASC9/miR-590-3p/SIX1 regulatory network.
The NF-κB pathway is essential to immunity, and its importance in cancer development has been extensively studied, especially in relation to tumor cell proliferation, survival, and metastasis. Multiple studies have shown that lncRNAs and several protein-coding genes regulate the NF-κB signaling pathway [Citation54–56]. As reported by Yang et al., SIX1 knockdown dramatically suppressed NF-κB activation [Citation36]. Han et al. reported that the DNAJC3-AS1/miR-214-3p axis affected NF-κB activation via the regulation of LIVIN expression, which further impacted the malignant phenotype of colorectal cancer cells [Citation56]. Gao et al. demonstrated that the inhibition of XIST promoted U2OS cell death via the activation of the NF-κB/PUMA pathway [Citation55]. Here, we examined the levels of the NF-κB protein p65, apoptosis-associated protein Bcl2, and MMP9. SIX1, p65, MMP9, and Bcl2 protein levels were reduced by CASC9 knockdown, and miR-590-3p overexpression enhanced these effects. Thus, the CASC9/miR-590-3p/SIX1/NF-κB axis is involved in BC progression. However, there are some limitations to our study. For example, an electrophoretic mobility shift assay was not performed to confirm the involvement of the NF-κB signaling pathway. In addition, RNA immunoprecipitation and RNA pull-down assays were not performed to confirm the relationship between CASC9, miR-590-3p, and SIX1.
5. Conclusion
In this study, we established that CASC9 regulates BC tumorigenesis by modulating the SIX1/NF-κB signaling pathway via miR-590-3p. Thus, CASC9 may represent an attractive therapeutic target for the prevention and treatment of BC.
Highlights
(1) CASC9 was up-regulated in BC.
(2) CASC9 promoted proliferation, migration, invasion and inhibited apoptosis of BC cells.
(3) CASC9 regulated SIX1 by sponging miR-590-3p.
(4) CASC9/miR-590-3p/SIX1 regulated BC through NF-κB signaling.
Disclosure statement
No conflicts of interest was reported by the author(s).
Data availability statement
The data used to support the findings of this study are available from the corresponding author upon request.
Additional information
Funding
References
- Bertucci F, Ng CKY, Patsouris A, et al. Genomic characterization of metastatic breast cancers. Nature. 2019;569(7757):560–564.
- Siegel RL, Miller KD, Jemal A. Cancer statistics, 2019. CA Cancer J Clin. 2019;69(1):7–34.
- Nagini S. Breast cancer: current molecular therapeutic targets and new players. Anticancer Agents Med Chem. 2017;17(2):152–163.
- Ponting CP, Oliver PL, Reik W. Evolution and functions of long noncoding RNAs. Cell. 2009;136(4):629–641.
- Zhao W, Lin X, Han H, et al. Long noncoding RNA H19 contributes to the proliferation and autophagy of glioma cells through mTOR/ULK1 pathway. Neuroreport. 2021;32(5):352–358.
- Zhang W, Zhou K, Zhang X, et al. Roles of the H19/microRNA‑675 axis in the proliferation and epithelial‑mesenchymal transition of human cutaneous squamous cell carcinoma cells. Oncol Rep. 2021;45(4):1–13.
- Wang Y, Zhou P, Li P, et al. Long non-coding RNA H19 regulates proliferation and doxorubicin resistance in MCF-7 cells by targeting PARP1. Bioengineered. 2020;11(1):536–546.
- Li Y, Ma HY, Hu XW, et al. LncRNA H19 promotes triple-negative breast cancer cells invasion and metastasis through the p53/TNFAIP8 pathway. Cancer Cell Int. 2020;20(1):200.
- Yan L, Yang S, Yue C-X, et al. Long noncoding RNA H19 acts as a miR −340-3p sponge to promote epithelial-mesenchymal transition by regulating YWHAZ expression in paclitaxel-resistant breast cancer cells. Environ Toxicol. 2020;35(9):1015–1028.
- Xiong H, Shen J, Chen Z, et al. H19/let‑7/Lin28 ceRNA network mediates autophagy inhibiting epithelial‑mesenchymal transition in breast cancer. Int J Oncol. 2020;56(3):794–806.
- Huang Y, Wang X, Zheng Y, et al. Construction of an mRNA-miRNA-lncRNA network prognostic for triple-negative breast cancer. Aging (Albany NY). 2021;13(1):1153–1175.
- Wang S, Lan F, Xia Y. lncRA ANCR inhibits non-small cell lung cancer cell migration and invasion by inactivating TGF-β pathway. Med Sci Monit. 2018;24:6002–6009.
- Qin S, Ning M, Liu Q, et al. Knockdown of long non-coding RNA CDKN2B-AS1 suppresses the progression of breast cancer by miR-122-5p/STK39 axis. Bioengineered. 2021;12(1):5125–5137.
- Hu X, Li Y, Kong D, et al. Long noncoding RNA CASC9 promotes LIN7A expression via miR-758-3p to facilitate the malignancy of ovarian cancer. J Cell Physiol. 2019;234(7):10800–10808.
- Liang Y, Chen X, Wu Y, et al. LncRNA CASC9 promotes esophageal squamous cell carcinoma metastasis through upregulating LAMC2 expression by interacting with the CREB-binding protein. Cell Death Differ. 2018;25(11):1980–1995.
- Zhao W, Chen T, Zhao Y. Upregulated lncRNA CASC9 contributes to progression of non-small cell lung cancer through inhibition of miR-335-3p and activation S100A14 Expression. Onco Targets Ther. 2020;569:6027–6036.
- Jiang B, Li Y, Qu X, et al. Long noncoding RNA cancer susceptibility candidate 9 promotes doxorubicin‑resistant breast cancer by binding to enhancer of zeste homolog 2. Int J Mol Med. 2018;42(5):2801–2810.
- Liu H-Z, Shan T-D, Han Y, et al. Silencing long non-coding RNA CASC9 inhibits colorectal cancer cell proliferation by acting as a competing endogenous RNA of miR-576-5p to regulate AKT3. Cell Death Discov. 2020;6(1):115.
- Zhang H, Liu S, Tang L, et al. Long non-coding RNA (LncRNA) MRPL23-AS1 promotes tumor progression and carcinogenesis in osteosarcoma by activating Wnt/β-catenin signaling via inhibiting microRNA miR-30b and upregulating myosin heavy chain 9 (MYH9). Bioengineered. 2021;12(1):162–171.
- Li X, Chen B, Chi D, et al. lncRNA CASC9 regulates cell migration and invasion in hemangioma endothelial cells by targeting miR-125a-3p/Nrg1. Onco Targets Ther. 2019;12:423–432.
- Shao G, Wang M, Fan X, et al. lncRNA CASC9 positively regulates CHK1 to promote breast cancer cell proliferation and survival through sponging the miR‑195/497 cluster. Int J Oncol. 2019;54(5):1665–1675.
- Yan M, Ye L, Feng X, et al. MicroRNA-590-3p inhibits invasion and metastasis in triple-negative breast cancer by targeting Slug. Am J Cancer Res. 2020;10(3):965–974.
- Abdolvahabi Z, Nourbakhsh M, Hosseinkhani S, et al. MicroRNA-590-3P suppresses cell survival and triggers breast cancer cell apoptosis via targeting sirtuin-1 and deacetylation of p53. J Cell Biochem. 2019;120(6):9356–9368.
- Wang WT, Qi Q, Zhao P, et al. miR-590-3p is a novel microRNA which suppresses osteosarcoma progression by targeting SOX9. Biomed Pharmacother. 2018;107:1763–1769.
- Gao J, Yu SR, Yuan Y, et al. MicroRNA-590-5p functions as a tumor suppressor in breast cancer conferring inhibitory effects on cell migration, invasion, and epithelial-mesenchymal transition by downregulating the Wnt-β-catenin signaling pathway. J Cell Physiol. 2019;234(2):1827–1841.
- Guan H, Liu J, Lv P, et al. MicroRNA‑590 inhibits migration, invasion and epithelial‑to‑mesenchymal transition of esophageal squamous cell carcinoma by targeting low‑density lipoprotein receptor‑related protein 6. Oncol Rep. 2020;44(4):1385–1392.
- Zhang Y, Chang J, Jiang W, et al. Long non-coding RNA CASC9/microRNA-590-3p axis participates in lutein-mediated suppression of breast cancer cell proliferation. Oncol Lett. 2021;22(1):544.
- Yang C, Xu W, Gong J, et al. Six1 overexpression promotes glucose metabolism and invasion through regulation of GLUT3, MMP2 and snail in thyroid cancer cells. Onco Targets Ther. 2020;13:4855–4863.
- Kong D, Li A, Liu Y, et al. SIX1 activates STAT3 signaling to promote the proliferation of thyroid carcinoma via EYA1. Front Oncol. 2019;9:1450.
- Tang D, Zhao L, Peng C, et al. CRNDE promotes hepatocellular carcinoma progression by upregulating SIX1 through modulating miR-337-3p. J Cell Biochem. 2019;120(9):16128–16142.
- Smith AL, Iwanaga R, Drasin DJ, et al. The miR-106b-25 cluster targets Smad7, activates TGF-β signaling, and induces EMT and tumor initiating cell characteristics downstream of Six1 in human breast cancer. Oncogene. 2012;31(50):5162–5171.
- Micalizzi DS, Wang CA, Farabaugh SM, et al. Homeoprotein Six1 increases TGF-beta type I receptor and converts TGF-beta signaling from suppressive to supportive for tumor growth. Cancer Res. 2010;70(24):10371–10380.
- Wan J, Yang J, Qiao C, et al. MicroRNA-362 inhibits cell proliferation and invasion by directly targeting SIX1 in colorectal cancer. Yonsei Med J. 2019;60(5):414–422.
- Liu X, Zhou X, Chen Y, et al. miR-186-5p targeting SIX1 inhibits cisplatin resistance in non-small-cell lung cancer cells (NSCLCs). Neoplasma. 2020;67(1):147–157.
- Lv DQ, Li HY, Wu XM, et al. MiR-188 inhibits proliferation and promotes apoptosis of lung adenocarcinoma cells by targeting SIX1 to negatively regulate ERK signaling pathway. Eur Rev Med Pharmacol Sci. 2020;24(2):721–727.
- Yang ZC, Yi MJ, Shan YC, et al. Targeted inhibition of Six1 attenuates allergic airway inflammation and remodeling in asthmatic mice. Biomed Pharmacother. 2016;84:1820–1825.
- Du P, Luo K, Li G, et al. Long non-coding RNA VCAN-AS1 promotes the malignant behaviors of breast cancer by regulating the miR-106a-5p-mediated STAT3/HIF-1α pathway. Bioengineered. 2021;12(1):5028–5044.
- Yin D, Lu X. Silencing of long non-coding RNA HCP5 inhibits proliferation, invasion, migration, and promotes apoptosis via regulation of miR-299-3p/SMAD5 axis in gastric cancer cells. Bioengineered. 2021;12(1):225–239.
- Dong Y, Zhang X, Wang F, et al. [Construction of lentivirus plasmid pCDH-NLRX1 and stable expression of NLRX1 in A549 cells]. Xi Bao Yu Fen Zi Mian Yi Xue Za Zhi. 2020;36(2):152–156.
- Wu N, Zhang X, Bao Y, et al. Down-regulation of GAS5 ameliorates myocardial ischaemia/reperfusion injury via the miR-335/ROCK1/AKT/GSK-3β axis. J Cell Mol Med. 2019;23(12):8420–8431.
- Tang Z, Li C, Kang B, et al. GEPIA: a web server for cancer and normal gene expression profiling and interactive analyses. Nucleic Acids Res. 2017;45(W1):W98–W102.
- Paraskevopoulou MD, Vlachos IS, Karagkouni D, et al. DIANA-LncBase v2: indexing microRNA targets on non-coding transcripts. Nucleic Acids Res. 2016;44(D1):D231–8.
- Agarwal V, Bell GW, Nam JW, et al. Predicting effective microRNA target sites in mammalian mRNAs. Elife. 2015;4:e05005.
- Li Q, Li Z, Fan Z, et al. Involvement of non‑coding RNAs in the pathogenesis of myocardial ischemia/reperfusion injury (Review). Int J Mol Med. 2021;47(4):1.
- Guan H, Shang G, Cui Y, et al. Long noncoding RNA APTR contributes to osteosarcoma progression through repression of miR-132-3p and upregulation of yes-associated protein 1. J Cell Physiol. 2019;234(6):8998–9007.
- Shang G, Wang Y, Xu Y, et al. Long non-coding RNA TCONS_00041960 enhances osteogenesis and inhibits adipogenesis of rat bone marrow mesenchymal stem cell by targeting miR-204-5p and miR-125a-3p. J Cell Physiol. 2018;233(8):6041–6051.
- Nilsson F, Storm P, Sozzi E, et al. Profiling of coding and noncoding genes in human dopamine neuron differentiation. Cells. 2021;10(1):137.
- Yan S, Xu J, Liu B, et al. Long non-coding RNA BCAR4 aggravated proliferation and migration in esophageal squamous cell carcinoma by negatively regulating p53/p21 signaling pathway. Bioengineered. 2021;12(1):682–696.
- Guan H, Mei Y, Mi Y, et al. Downregulation of lncRNA ANRIL suppresses growth and metastasis in human osteosarcoma cells. Onco Targets Ther. 2018;11:4893–4899.
- Sang Y, Tang J, Li S, et al. LncRNA PANDAR regulates the G1/S transition of breast cancer cells by suppressing p16(INK4A) expression. Sci Rep. 2016;6:22366.
- Zhang Z, Chen F, Zhan H, et al. lncRNA CASC9 sponges miR‑758‑3p to promote proliferation and EMT in bladder cancer by upregulating TGF‑β2. Oncol Rep. 2021;45(1):265–277.
- Chen Y, Li Y, Gao H. Long noncoding RNA CASC9 promotes the proliferation and metastasis of papillary thyroid cancer via sponging miR-488-3p. Cancer Med. 2020;9(5):1830–1841.
- Zhang J, Wang Q, Quan Z. Long non-coding RNA CASC9 enhances breast cancer progression by promoting metastasis through the meditation of miR-215/TWIST2 signaling associated with TGF-β expression. Biochem Biophys Res Commun. 2019;515(4):644–650.
- Gao W, Gao J, Chen L, et al. XIST induced apoptosis of human osteosarcoma cells by activation of NF-kB/PUMA signal. Bioengineered. 2019;10(1):261–270.
- Yang X, Zhang Q, Lu H, et al. Suppression of lncRNA MALAT1 Reduces LPS- or IL-17A-induced inflammatory response in human middle ear epithelial cells via the NF-κB signaling pathway. Biomed Res Int. 2021;2021:8844119.
- Han B, Ge Y, Cui J, et al. Down-regulation of lncRNA DNAJC3-AS1 inhibits colon cancer via regulating miR-214-3p/LIVIN axis. Bioengineered. 2020;11(1):524–535.