ABSTRACT
The present study was focused on comparison of four typical fungicides in ginseng field to evaluate the impact of the different fungicides on the soil bacterial and fungal communities’ composition and diversity by using high-throughput sequencing. Five treatments were designed comprising carbendazim (D), dimethyl disulfide (E), dazomet (M), calcium cyanamide (S), and control (C). The application of fungicide obviously altered the distribution of dominant fungal and bacterial communities and remarkably decreased the diversity (1099-763 and 6457-2245). The most abundant Proteobacteria obviously degenerate in fungicide-treated soil and minimum in E (0.09%) compared to control (25.72%). The relative abundance of Acidobacteria was reduced from 27.76 (C) to 7.14% after applying fungicide and minimum in E. The phylum Actinobacteria are both decomposers of organic matter and enemies of soil-borne pathogens, elevated from 11.62 to 51.54% and are high in E. The fungi community mainly distributed into Ascomycota that enriched from 66.09 to 88.21% and highin M and E (88.21 and 85.10%), and Basidiomycota reduced from 21.13 to 3.23% and low in M and E (5.27 and 3.23%). Overall, environmentally related fungicides decreased the diversity and altered the composition of bacterial and fungal communities, highest sensitivity present in dimethyl disulfide-treated soil.
1. Introduction
Considering the ginseng (Panax ginseng C. A. Meyer) contributes as precious and high-value Chinese herbal medicine, while various microbes proliferate in soil during the long planting period, and once cross the soil tolerance limit, it will explode root rot or other diseases [Citation1,Citation2]. Therefore, it is very essential to the annual periodic maintenance of soil culture and disinfection. Fungicides are widely applied currently and still the most powerful weapon to prevent and control various pathogenic microorganisms during agrochemical crop protection industry [Citation1]. The typical fungicide dazomet is a low-toxic and broad-spectrum soil fumigant, successfully replacing methyl bromide as well as widely used in ginseng fields to control plant pathogenic nematodes. Since dazomet could be decomposed in soil and generate toxic gases such as methyl isothiocyanate and formaldehyde that rapidly spreads to kill bacteria, fungi, nematodes, and weed seeds [Citation3]. The fungicide dimethyl disulfide is derived from natural source and considered as an efficient nematicide to effectively control nematodes and soil-borne pathogens and weeds during high-value crop production [Citation4]. At the same time, the fungicide carbendazim is widely used to control fungal pathogens or leaf diseases, and the fungicide calcium cyanamide is an effective ecological fertilizer for controlling soil-borne diseases and increasing nitrogen use efficiency. As calcium cyanamide can kill most root-knot nematodes and nematode eggs and generate toxic cyanamide liquid in soil by high temperature and solar radiation, thereby inhibiting the growth of root-knot nematodes, effectively protecting underground roots and producing nitrification inhibitor dicyandiamide during fungicide degradation process [Citation5,Citation6].
The microbial community as the vital core of soil ecological environment is responsible in nutrient cycling, plant growth, resistance, and stress response. There are many studies focuseing on fungicides that are responsible for microbial community composition and diversity as well as metabolic activity. Fang et al. [Citation4] evaluated the efficiency of fungicides chloropicrin, dimethyl disulfide, dazomet, allyl isothiocyanate, and 1,3-dichloropropene on soil-borne diseases and pests’ control, and concluded the significant changes of denitrifying bacteria community abundance. Zhang et al. [Citation7] and Han et al. [Citation8] reported fungicide difenoconazole and tebuconazole obviously reduced bacterial community diversity and network complexity. Yan et al. [Citation9] pointed out the presence of chloramphenicol increased the inhibitory effect of carbendazim on the fungi/bacteria ratio. Wang et al. [Citation10] reported that the repeated application of carbendazim reduces soil microbial diversity and temporarily altered bacterial community composition, and suggest that carbendazim should be frequently applied during plant growing season to control fungal and foliage diseases of cultivable crops. The fungicides may cause an increase in Gram-positive bacteria and decrease in Gram-negative bacteria and fungi, and the beneficial microorganisms have the potential to prevent further infection of pathogen infection and control pests/diseases and promote plant growth, such as Chaetomium, Pseudomonas, and Bacillus that are considered as bio-controller and direct-attack soil-borne diseases [Citation2,Citation11,Citation12].
The effect of fungicides on the soil microbial community varies with fungicides character, type, and dosage as well as soil and crop types. Accordingly, the present study focuses on the comparison of four typical fungicides dazomet, dimethyl disulfide, calcium cyanamide, and carbendazim in ginseng field to evaluate the impact of the different fungicides on the soil bacterial and fungal communities’ composition and diversity at the molecular level by using high-throughput sequencing.
2. Materials and methods
2.1. Experiment design and sample collection
The present study was carried out in ginseng field of Ji’an City (Jilin, China). Five treatments were designed and comprised 50% carbendazim wettable powder (D, provided by Fuda Agrochemical Co., Ltd., Jiangyin City, Jiangsu, China), 99.5% dimethyl disulfide agent (E, purchase from Baisite Reagent Co., Ltd, Chengdu, China), 98% dazomet particle agent (M, provided by Hisun Chemical Co., Ltd, Zhejiang, China), 50% calcium cyanamide granules (S, purchased from Zhaolong Science and Technology Development Co., Ltd, Jinan, China) and control (C, without any fungicide). Each treatment was performed in the divided block area (25 m2) with four times replication, the dosage of fungicide and active ingredient are listed in .
Table 1. The dosage of different fungicide and active ingredient in five treatments
The soil samples (0–20 cm) of each plot were randomly collected according to the five-point method by stainless-steel cylindrical driller with a diameter of 5 cm and kept in a portable refrigerator (−20°C). After the soil samples were transported to the laboratory, passed through 2 mm sieve to remove plant tissues, roots, rocks, and stored at −20°C for microbial (bacterial and fungal) sequencing analysis.
2.2. Microbial diversity information analysis
Microbial sequencing was conducted by second-generation high-throughput sequencing technology to evaluate soil bacterial and fungal diversity information response of different fungicides. Firstly, total DNA was extracted according to Power Soil DNA Isolation Kit (MoBio Laboratories, Carlsbad, CA) instruction, and performed purity testing via Nano Drop 2000 UV-vis spectrophotometer (Thermo Scientific, Wilmington, USA). Then, target variable regions were amplified by PCR, use 338 F and 806 R primers (5-ACTCCTACGGGAGGCAGCAG-3 and 5-GGACTACHVGGGTATCTAAT-3) amplified V3-4 hypervariable region for bacterial, ITS1F and ITS2 primers (5-CTTGGTCATTTAGAGGAAGTAA-3 and 5-TGCGTTCTTCATCGATGC-3) amplified ITS region for fungal. For each soil sample, 8-digit barcode sequence was added to the 5 ends of the forward and reverse primers (provided by Allwegene Company, Beijing). The PCR was carried out by Mastercycler Gradient (Eppendorf, Germany) using 25 μL reaction volumes, containing 12.5 μL 2× Taq PCR Master Mix, 3 μL BSA (2ng/μL), 1 μL Forward Primer (5 μM), 1 μL Reverse Primer (5 μM), 2 μL template DNA, and 5.5 μL ddH2O. The cycling parameters were 95°C for 5 min, followed by 28 cycles of 95°C for 45 s, 55°C for 50 s and 72°C for 45s with a final extension at 72°C for 10 min [Citation13]. The PCR products were purified by use of Agencourt AMPure XP nucleic acid purification kit. Followed step was Miseq library construction and performed high throughput sequencing on Miseq platform at Allwegene Company (Beijing, China).
2.3. Sequence process and data analyses
The raw sequences were first screened and quality controlled by Trimmomatic (v0.36) and Flash (v1.20) to remove low-quality score (≤20) and obtained fasta sequence, then remove chimera and short sequence to get high-quality sequence clean_tags. Then used Uparse algorithm of Vsearch (v2.7.1) clustered qualified clean tags into operational taxonomic units (OTUs) at 97% similarity [Citation14]. The Ribosomal Database Project and BLAST Classifier tool were used to classify and annotate all sequences of different bacterial and fungal taxonomic levels according to SILVA128 and Unite database [Citation15].
In order to compare bacterial and fungal communities in different samples, barplot diagram and heatmaps were generated based on taxonomic annotation by using R (v3.6.0) [Citation16]. To evaluate the similarity between different fungicide-treated soil, alpha diversity index was done by QIIME (v1.8.0), and beta diversity-based principal co-ordinates and principal component analysis as well as nonmetric multidimensional scaling were performed by R (v3.6.0) [Citation17]. Finally, the correlation among selected genes was performed in network by R (v3.6.0) igraph and psych package.
3. Results and discussion
3.1 Responses of dominant bacterial and fungal communities to different fungicides
Among the samples, the dominant top five phyla were Proteobacteria, Actinobacteria, Acidobacteria, Chloroflexi, and Firmicutes. In particular, fungicide with calcium cyanamide (S) observed an obviously richness in Proteobacteria (32.39%), while decreased in carbendazim (24.88%) and dazomet (22.69%) treated soil, minimum in dimethyl disulfide (0.09%) compare with control (25.72%). The most abundant Proteobacteria was not surprising due to its high adaptability to unfavorable environments and dominant in carbon/nitrogen cycling [Citation8,Citation18]. The favorable habitat to Proteobacteria than Acidobacterium caused prevailing existence of Pseudomonas leading to adverse interference or disease on plant development [Citation10,Citation19]. Thus, the obvious degenerate tendency of Proteobacteria abundance after applying fungicides in this study revealed the positive effect of fungicide on beneficial microbial. Conversely, Acidobacteria could oxidize iron and deposit and caused surface rust in ginseng root [Citation20], and the relative abundance (RA) of Acidobacteria was reduced from 27.76 to 7.14% and minimum in E. However, the phylum Actinobacteria was contributed as decomposers of organic matter and enemies of soil-borne pathogens or act as biocontrol agents to control plant diseases transmitted by soil and seeds [Citation7,Citation21]. The positive effect of fungicide demonstrated by elevated RA of Actinobacteria from 11.62 to 51.54% and significantly highin E (51.54%), followed by D (19.33%) and M (17.41%). Meanwhile Chloroflexi is related to organic halide respiration and increased the richness (11.82–17.33%) after applying fungicide. Firmicutes as the important actor during organic matter decomposition and carbon cycling, the RA increased (3.18–17.03%) in fungicide-treated soil [Citation8]. More specifically, the class Actinobacteria was dominant in E (47.45%) while Alphaproteobacteria reduced from 15.29 to 4.84% and minimum in E. The abundance of Ktedonobacteria and Gammaproteobacteria were increased (6.09–1.09% and 5.11–12.32%) as well as richest in D-treated soil, and the richest genus of Arthrobacter and Bacillus were the highest in E (19.74% and 4.45%) (). Similarly, Huang et al. [Citation22] observed significantly increased in Arthrobacter abundance in dazomet-treated soil, which might be attributed by Arthrobacter ability to degrade dazomet as a nutrient source. And Bacillus is favorable to rhizobacteria that absorbs nutrients and promotes plant yield, and also potentially contributed as antimicrobial agents owing to the production of a variety of antibiotics, including bacitracin and lipopeptides, polyketones, and lipopeptides [Citation23,Citation24], further used in organic biological products to combat plant pathogens and inhibit various soil-borne pathogens.
Figure 1. The dominant bacterial community response of different fungicides-based phylum (a), class (b), order (c), family (d), genus (e) level. the control (c), carbendazim (D), dimethyl disulfide (E), dazomet (m), and calcium cyanamide (s)
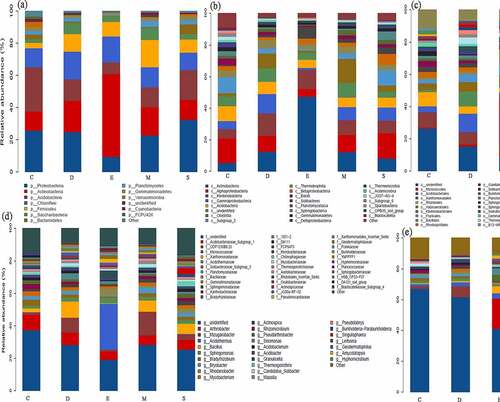
In terms of fungi community, there are fewer types of dominant phyla compared to bacteria and mainly distributed into Ascomycota and Basidiomycota in fungicide-treated soil. The fungicide triggers Ascomycota enriched from 66.09 to 88.21% and high in M and E (88.21 and 85.10%), while Basidiomycota reduced from 21.13 to 3.23% and lowest in M and E (5.27 and 3.23%), meanwhile Mortierellomycota and Chytridiomycota account less than 1%. Particularly, the highest richness class Sordariomycetes was present in M (53.28%), and the RA of Eurotiomycetes increased from 9.51 to 21.11% and Agaricomycetes was reduced from 18.97 to 1.41%. Notably, the existence of dimethyl disulfide contributed competitive advantage for Trichoderma and Botrytis (21.82 and 21.15%) (). The previous study also observed the predominant bacterial in ginseng soil is Proteobacteria and Actinobacteria and predominant fungi including Ascomycota, Basidiomycota and Zygomycota [Citation2,Citation12]. Han et al. pointed out that different fumigation obviously reduced the abundance of Acidobacteria, Proteobacteria and Chloroflexi, while increased Actinobacteria, Firmicutes, Verrucomicrobia, Gemmatimonadetes, and Saccharibacteria [Citation8]. Overall, the application of fungicides regulated the dominant bacterial and fungal community’s abundance by promoting beneficial microbials.
3.2. The shift of dominant bacterial and fungal communities under different fungicides
The application of fungicide is a remarkable shift of dominant bacterial and fungi communities. As shown the predominant microbial conversion from control to fungicide-treated soil in heatmap . The richness bacterial Proteobacteria and Acidobacteria is converted into Proteobacteria and Actinobacteria, Alphaproteobacteria is converted in to Actinobacteria and Clostridia, Acidobacteriales and Rhizobiales are converted into Micrococcales and Xanthomonadales, and genus enriched in Pseudarthrobacter, Sinomonas, Bacillus and Arthrobacter after treated with fungicide. Additionally, the richness fungal from Basidiomycota is converted into unidentified, Agaricomycetes is converted into Eurotiomycetes, Hypocreales is converted into Sordariales. Nectriaceae is converted into Hypocreaceae and Sclerotiniaceae, and genus enriched in Fusarium and Trichoderma after treated with fungicide.
Figure 3. The shift of dominant bacterial community under different fungicides at phylum (a), class (b), order (c), family (d), genus (e) level. the control (C), carbendazim (D), dimethyl disulfide (E), dazomet (m), and calcium cyanamide (s)
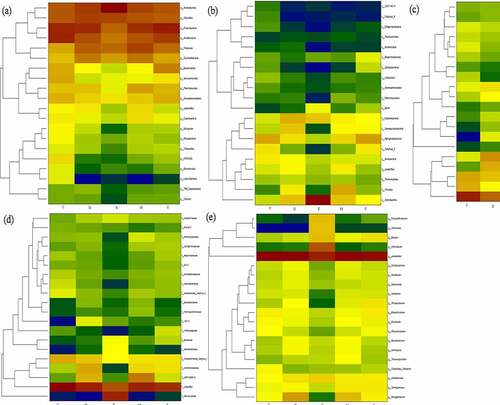
Figure 4. The shift of dominant fungal community under different fungicides at phylum (a), class (b), order (c), family (d), genus (e) level. the control (C), carbendazim (D), dimethyl disulfide (E), dazomet (m), and calcium cyanamide (s)
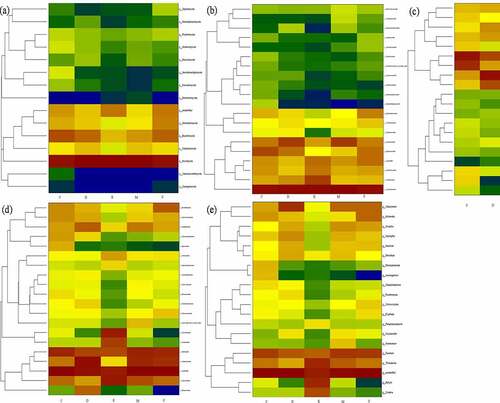
In particularly, the present study detected potential microbial for biocontrol and plant growth promotion, such as inhibition of disease-related bacteria Bacillaceae, Streptomycetaceae, Micromonosporaceae, Chaetomium, and Bacillus. While pathogenic fungal of Cylindrocarpon, Alternaria, Rhizoctonia solani and Gibberella were not identified in this study, it might be attributed by obligate parasitism. The Actinomycete members Streptomyces and Micromonospora can produce broad-spectrum of antibiotics, and enzymes further suppress multiple plant diseases by pathogen inhabitation [Citation12,Citation25]. However, Fusarium includes many members and mainly causes the pathogenicity of diverse plants (e.g., wheat, cucumber, peanut, and cotton) and related to agriculture, environment, and human health [Citation2,Citation26]. Baudy et al. [Citation27] reported the root rot was caused by Cylindrocarpon, and Fusarium was the major reason for yield decreasing and poor growth of ginseng. And pathogenic potentialfungi Mortierella is widely distributed in temperate zone and usually colonized on plant roots and associated with replant disease [Citation28]. Biofertilizer and biocontrol agent of Chaetomium could improve root mass and enzyme activity, and Chaetomium globosum can affect pathogen Coniothyrium Diplodiella and Rhizopus stolonifera [Citation17,Citation29]. Nicola et al. [Citation28] found that Bacillus is effectively defensing against Fusarium Wilt and dominated in carbendazim treated soil.
The diverse dominant microbial phenomena were attributed by different types of fungicide affection. The application of dazomet obviously modified bacterial community by directly toxifying methyl isothiocyanate, and Eo et al. [Citation30] identified the most resistant fungal to dazomet was Trichoderma and followed by Zygorhynchus and Rhizopus. Zhu et al. [Citation31] noticed a rapidly increasing trend of Penicillium, Nitrolancea, and Pseudomonas, while decreasing tendency of Fusarium and Mortierella, Nitrospira and Aquicella after applying dazomet. Shen et al. [Citation32] identified the dominant genus, Bacteroides, Flavobacterium, and Cytophaga, and the effect of carbendazim on bacterial community in arable land was mainly contributed by g-Proteobacterium. Fang et al. [Citation33] reported that carbendazim alters bacterial community mostly owing to γ-Proteobacteria, and carbendazim may suppress Pseudomonas, Mycobacterium, Bacillus, Arthrobacter, Burkholderia, and Streptococcus. Additionally, dimethyl disulfide could be damaging the membrane of Sclerotinia minor and is responsible for antifungal activity, then induces host plants systemic resistance [Citation34]. Previous studies have shown that the sensitivity of different microorganisms to cyanamide hydrogen is largely different, some nonpathogenic fungi including Penicillium and Aspergillus can degrade cellulose using cyanoamide as nitrogen source. The calcium cyanamide inhibits spore the formation and growth of pathogenic fungi based on intermediate metabolite hydrogen cyanide [Citation13], and calcium cyanide non-targeting effect on microorganisms can be reflected by local microbial richness and diversity.
3.3. Alpha and beta diversity of bacterial and fungi communities after apply fungicide
The alpha and beta diversity of soil bacteria and fungi had significant changes in the different fungicide treatments (). For bacterial, the richness index value of chao 1 decreased from 6457 in C to 2344, 2245, 2560, and 3846 in D, E, M, and S. The number of OTUs actually observed from 4306 (C) decreased to 1786, 1660, 1894, and 2714 in D, E, M, and S. Considering microbial abundance and the diversity index of evolutionary distance, PD whole tree decreased from 293 to 139, 134, 148, and 192 as well as Shannon index was 9.7, 8.3, 7.1, 8.4, and 8.9 in C, D, E, M, and S. On the other hand, about fungal community, chao 1 value decreased from 1099 (C) to 970, 763, 829, and 816 in D, E, M, and S. Observed species decreased from 806 (C) to 659, 436, 576, and 616 in D, E, M, and S. PD whole tree value was 149, 121, 90, 109, and 134 as well as Shannon was 5.9, 5.6, 3.1, 5.4, and 5.5 in C, D, E, M, and S. Therefore, fungicide application had strong interference on soil microbial community, bacterial, and fungal communities’ alpha-diversity remarkably decreased after treated with fungicide, consistent with [Citation35]. Mumbi et al. [Citation13] pointed out that the diversity and evenness of microorganism were decreased at the beginning of calcium cyanide treated soil, while soil bacteria are less sensitive to calcium cyanide than fungi. Zhang et al. and Nicola et al. [Citation12,Citation28] also reported bacterial diversity decreased significantly under carbendazim treated soil and bacteria have high tolerance to carbendazim. Tortella et al. [Citation36] found out that carbendazim slightly influenced the amount of culturable fungi and bacteria, and carbendazim inhibited the functional diversity of bacterial communities in arable land, mainly due to its effect on g-Proteobacterium. In addition, Venn figure demonstrated that 1524 and 332 shared OTUs for bacterial and fungi, the unique OTUs 4972, 331, 173, 109, and 456 for bacterial while 257, 100, 41, 76, and 278 for fungi during C, D, E, M, and S (Figure S1). Therefore, soil respiration activities are mainly contributed by bacterial metabolism, and the respiration of bacteria is more sensitive and approximately three times than fungi, that is opposite to Bamaga et al. [Citation11] who observed stronger fungal respiration than bacteria and pointed out the inhibition of carbendazim on soil fungi is greater than bacteria.
Figure 5. Alpha diversity of bacterial chao1(a), observed _species (b), PD whole tree (c) and shannon (d), and fungi chao1 (e), observed _species (f), PD whole tree (g) and shannon (h). the control (C), carbendazim (D), dimethyl disulfide (E), dazomet (m), and calcium cyanamide (s)
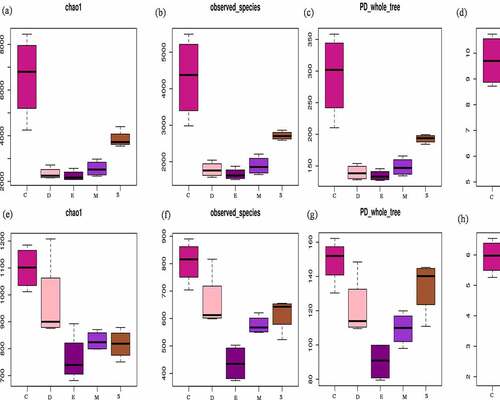
Figure 6. Beta diversity based bacterial principal co-ordinates (a), nonmetric multidimensional scaling (b), principal component analysis (c), fungal principal co-ordinates (d), nonmetric multidimensional scaling (e), principal component analysis (f). The control (C), carbendazim (D), dimethyl disulfide (E), dazomet (m), and calcium cyanamide (s)
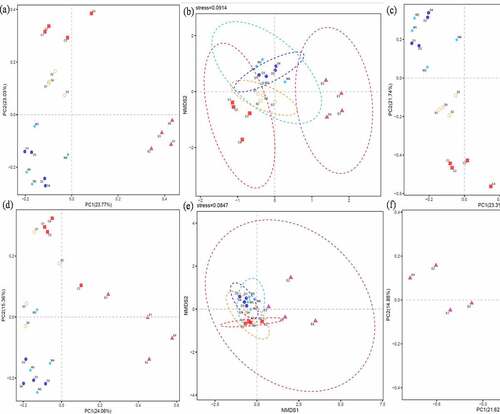
Similarly, Zhu et al. [Citation31] reported that the Venn diagram presenting 1524 and 332, which overlapped OTUs for bacterial and fungi. Wang et al. [Citation37] elaborated that soil bacterial community structure changed after apply carbendazim, and the inhibition of bacterial community by carbendazim is temporary. Furthermore, the beta diversity analysis during bacterial and fungal both identified that C and S were gathered one group, M and D were clustered into one unit, while E was individually distributed based on PCoA and PCA. While from perspective of NMDS analysis, E and C were distributed separately and remarkably dispersed bacterial/fungi community, while other three fungicides (M, D, and S) had weaker dispersion effect on bacterial community. Finally, the results of alpha and beta diversity demonstrated the bacteria and fungi community decreased significantly.
3.4. The correlation of predominant bacterial and fungal communities
The correlation among top 20 bacterial and fungi communities in genus level is demonstrated in network . The genus Acidobacterium was positive with Variibacter (0.61), Granulicella (0.74) and Acidibacter (0.73), while negative with Gemmatimonas (−0.10), Candidatus_Solibacter (−0.17) and Thermosporothrix (−0.01). The genus Rhizomicrobium was positive with Gemmatimonas (0.74) and Candidatus_Solibacter (0.69) while negative with Sinomonas (−0.55). The genus Rhodanobacter was positive with Variibacter (0.77) and Acidibacter (0.68), negative with Sinomonas (−0.46) and Pseudarthrobacter (−0.41). And Pseudarthrobacter was positive with Sinomonas (0.81) and negative with Acidibacter (−0.72) and Acidobacterium (−0.83). With regard to fungal, Mortierella was positive with Exophiala (0.82), Lectera (0.69), and Solicoccozyma (0.69). The genus Chaetomium was positive with Corynascella (0.78) and Aspergillus (0.77). The genus Exophiala was positive with Lectera (0.65) and Powellomyces (0.64), and negative with Paraphaeosphaeria (−0.12) and Leucoagaricus (−0.09). The genus Solicoccozyma was positive with Exophiala (0.80) and Corynascella (0.48). While genus Botrytis was negative with Lectera (−0.49), Powellomyces (−0.52), Exophiala (−0.80) Solicoccozyma (−0.74), Humicola (−0.72) and Mortierella (−0.79).
Figure 7. The correlation of selected bacterial (a) and fungal (b) communities-based network analysis
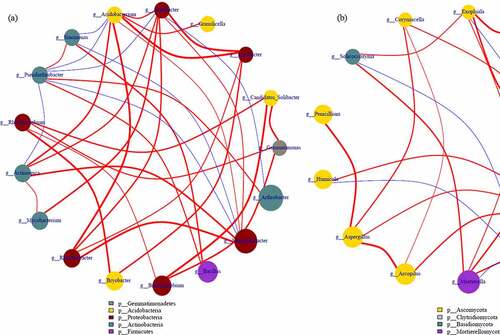
Accordingly, the correlation of bacterial communities was more complex than fungi, and fungicides reduced the complexity of microbial symbiosis network which is due to the reduction of functional microorganisms and consistent with Fournier et al. and Li et al. [Citation38,Citation39]. The main prevailence of Proteobacteria and Ascomycota in fungicide-treated soil indicated high resistance to chemical, similarly results are also found in the previous studies [Citation40–43]. Therefore, environmentally related fungicides decreased the diversity, altered the composition and interaction of bacterial and fungal communities rather than function. Han et al. [Citation8] conclude that the application of fungicides didn’t significantly alter the dominant fungi and bacteria communities, but the abundance changed obviously. Interestingly, the comparison of the microbial community treated with different fungicides showed that the RA of dominant beneficial microorganisms enriched and pathogen decreased, including bacterial of Bacillus, Pseudomonas and Arthrobacter fungi of Fusarium, Mortierella and Botrytis. Therefore, it is inferred that fungicide activates the defense activities of plants by changing the soil microbial community, and the adaptability and enrichment of beneficial microorganisms and reduction of pathogenic bacteria in soil may be the role of fungicides application.
4. Conclusion
Fungicide application strongly interfered on soil microbial community, remarkably decreased bacterial and fungal communities’ diversity. The respiration of bacteria is more sensitive and approximately three times than fungi. The abundance of dominant bacterial Proteobacteria decreased and fungal Ascomycota increased in fungicide treated soil, especially enriched in beneficial microorganisms and reduced in pathogen, including bacterial of Bacillus, Pseudomonas and Arthrobacter, fungi of Fusarium, Mortierella and Botrytis. The adaptability and enrichment of beneficial microorganisms and reduction of pathogenic bacteria in soil may be the role of fungicides application.
Supplemental Material
Download ()Acknowledgments
This study was funded by the National Key Research and Development Program of China (No.2017YFD0201606).
Disclosure statement
No potential conflict of interest was reported by the author(s).
Supplementary material
Supplemental data for this article can be accessed here.
Additional information
Funding
References
- Steinberg G, Gurr S. Fungi, fungicide discovery and global food security. Fungal Genet Biol. 2020;144:103476.
- Li Z, Fu J, Zhou R, et al. Effects of phenolic acids from ginseng rhizosphere on soil fungi structure, richness and diversity in consecutive monoculturing of ginseng. Saudi J Biol Sci. 2018;25(8):1788–1794.
- Fang W, Yan D, Wang X, et al. Responses of nitrogen-cycling microorganisms to dazomet fumigation. Front Microbiol. 2018;9:2529.
- Fang W, Wang X, Huang B, et al. Comparative analysis of the effects of five soil fumigants on the abundance of denitrifying microbes and changes in bacterial community composition. Ecotox Environ Safe. 2019;187:109850.
- Wang YS, Huang YJ, Chen WC, et al. Effect of carbendazim and pencycuron on soil bacterial community. J Hazard Mater. 2009;172(1):84–91.
- Shi K, Wang L, Zhou YH, et al. Effects of calcium cyanamide on soil microbial communities and Fusarium oxysporum f. sp. Cucumberinum. Chemosphere 2009;75(7):872–877.
- Li Q, Zhang D, Cheng H, et al. Chloropicrin alternated with dazomet improved the soil’s physicochemical properties, changed microbial communities and increased strawberry yield. Ecotox Environ Safe. 2021;220:112362.
- Han L, Kong X, Xu M, et al. Repeated exposure to fungicide tebuconazole alters the degradation characteristics, soil microbial community and functional profiles. Environ Pollut. 2021;287:117660.
- Yan H, Wang D, Dong B, et al. Dissipation of carbendazim and chloramphenicol alone and in combination and their effects on soil fungal: bacterial ratios and soil enzyme activities. Chemosphere. 2011;84(5):634–641.
- Wang X, Song M, Wang Y, et al. Response of soil bacterial community to repeated applications of carbendazim. Ecotox Environ Safe. 2012;75:33–39.
- Bamaga M, Wyszkowska J, Kucharski J. Response of soil microorganisms and enzymes to the foliar application of Helicur 250EW fungicide on Horderum vulgare L. Chemosphere. 2020;242:125163.
- Zhang H, Song J, Zhang Z, et al. Effects of cultivation ages and modes on microbial diversity in the rhizosphere soil of Panax ginseng diversity and the co-occurrence network complexity. J Hazard Mater. 2020;405:124208.
- Mumbi MP, Eissa N, Noah BC, et al. Antepartum antibiotic treatment increases offspring susceptibility to experimental colitis: a role of the gut microbiota. Plos One. 2015;10(11):e0142536.
- Edgar RC. UPARSE: highly accurate OTU sequences from microbial amplicon reads. Nat Methods. 2013;10(10):996.
- Cole JR, Wang Q, Cardenas E, et al. The Ribosomal Database Project: improved alignments and new tools for rRNA analysis. Nucleic Acids Res. 2009;37(Database):141–145.
- Jami E, Israel A, Kotser A, et al. Exploring the bovine rumen bacterial community from birth to adulthood. Isme J. 2013;7(6):1069–1079.
- Wang Y, Sheng HF, He Y, et al. Comparison of the levels of bacterial diversity in freshwater, intertidal wetland, and marine sediments by using millions of illumina tags. Appl Environ Microbiol. 2012;78(23):8264.
- Egamberdieva D, Wirth S, Li L, et al. Microbial cooperation in the rhizosphere improves liquorice growth under salt stress. Bioengineered. 2017;8(4):433–438.
- Zheng W, Wang L, Wang X, et al. Dominant protozoan species in rhizosphere soil over growth of Beta vulgaris L.in Northeast China. Northeast China. Bioengineered 2020;11(1):229–240.
- Wang Q, Sun H, Xu C, et al. Analysis of rhizosphere bacterial and fungal communities associated with rusty root disease of Panax ginseng. Appl Soil Ecol. 2019;138:245–252.
- Sarsaiya S, Shi J, Chen J. A comprehensive review on fungal endophytes and its dynamics on Orchidaceae plants: current research, challenges, and future possibilities. Bioengineered. 2019;10(1):316–334.
- Huang B, Yan D, Wang Q, et al. Effects of Dazomet Fumigation on Soil phosphorus and the Composition of pho D-Harboring Microbial Communities. J Agr Food Chem. 2020;68(18):5049–5058.
- Zhang C, Li Y, Zhang T, et al. Increasing chitosanase production in Bacillus cereus by a novel mutagenesis and screen method. Bioengineered. 2021;12(1):266–277.
- O’Mahony M, Henneberger R, Selvin J, et al. Inhibition of the growth of Bacillus subtilis DSM10 by a newly discovered antibacterial protein from the soil metagenome. Bioengineered. 2015;6(2):89–98.
- Jain A, Sarsaiya S, Wu Q, et al. A review of plant leaf fungal diseases and its environment speciation. Bioengineered. 2019;10(1):409–424.
- Shi TQ, Peng H, Zeng SY, et al. Microbial production of plant hormones: opportunities and challenges. Bioengineered. 2017;8(2):124–128.
- Baudy P, Zubrod JP, Konschak M, et al. Environmentally relevant fungicide levels modify fungal community composition and interactions but not functioning. Environ Pollut. 2021;285:117234.
- Nicola L, Turco E, Albanese D, et al. Fumigation with dazomet modifies soil microbiota in apple orchards affected by replant disease. Appl Soil Ecol. 2017;113:71–79.
- Gao J, Pei H, Xie H. Synergistic effects of organic fertilizer and corn straw on microorganisms of pepper continuous cropping soil in China. Bioengineered. 2020;11(1):1258–1268.
- Eo J, Park KC. Effects of dazomet on soil organisms and recolonisation of fumigated soil. Pedobiologia. 2014;57(3):147–154.
- Zhu F, Xiao J, Zhang Y, et al. Dazomet application suppressed watermelon wilt by the altered soil microbial community. Sci Rep. 2020;10(1):21668.
- Shen F, Wu W, Han X, et al. Study on the occurrence law and green control of grape gray mold from the perspective of ecological balance. Bioengineered. 2021;12(1):779–790.
- Fang H, Han L, Cui Y, et al. Changes in soil microbial community structure and function associated with degradation and resistance of carbendazim and chlortetracycline during repeated treatments. Sci Total Environ. 2016;572:1203–1212.
- Tyagi S, Lee KJ, Shukla P, et al. Dimethyl disulfide exerts antifungal activity against Sclerotinia minor by damaging its membrane and induces systemic resistance in host plants. Sci Rep. 2020;10(1):18183.
- Zhang H, Song J, Zhang Z, et al. Exposure to fungicide difenoconazole reduces the soil bacterial community diversity and the co-occurrence network complexity. J Hazard Mater. 2020;405:124208.
- Tortella GR, Mella-Herrera RA, Sousa DZ, et al. Carbendazim dissipation in the biomixture of on-farm biopurification systems and its effect on microbial communities. Chemosphere. 2013;93(6):1084–1093.
- Wang X, Song M, Gao C, et al. Carbendazim induces a temporary change in soil bacterial community structure. J Environ. 2009;12:1679–1683.
- Fournier B, Santos S, Gustavsen JA, et al. Impact of a synthetic fungicide (fosetyl-Al and propamocarb-hydrochloride) and a biopesticide (Clonostachys rosea) on soil bacterial, fungal, and protist communities. Sci Total Environ. 2020;738:139635.
- Li W, Shen S, Chen H. Bio-herbicidal potential of wheat rhizosphere bacteria on Avena fatua L. grass. Bioengineered. 2021;12(1):516–526.
- Zhang D, Yan D, Fang W, et al. Chloropicrin alternated with biofumigation increases crop yield and modifies soil bacterial and fungal communities in strawberry production. Sci Total Environ. 2019;675:615–622.
- Huang X, Zhao J, Zhou X, et al. Differential responses of soil bacterial community and functional diversity to reductive soil disinfestation and chemical soil disinfestation. Geoderma. 2019;348:124–134.
- Tian L, Shi S, Ma L, et al. The effect of Glomus intraradices on the physiological properties of Panax ginseng and on rhizospheric microbial diversity. J Ginseng Res. 2017;43(1):77–85.
- Rajesh R, Godan T, Sindhu R, et al. Bioengineering advancements, innovations and challenges on green synthesis of 2, 5-furan dicarboxylic acid. Bioengineered. 2020;11(1):19–38.