ABSTRACT
Recurrent pregnancy loss (RPL) is closely associated with insufficient functions of trophoblastic cells. Circular RNA forkhead box P1 (circFOXP1) can regulate cell activities in different types of diseases. However, its effects on trophoblastic cells and its role in RPL development remain unknown. In this study, gene expressions were detected by RT-qPCR. Protein levels were detected by Western blotting. Trophoblastic cell viability, apoptosis, invasion, and migration were respectively analyzed via CCK-8, flow cytometry, wound healing, and transwell assays. The association between miR–143–3p and circFOXP1 or S100A11 (S100 calcium binding protein A11) was explored and confirmed by bioinformatics prediction and luciferase reporter assay. Herein, miR–143–3p was upregulated in RPL. Furthermore, miR–143–3p upregulation induced apoptosis and suppressed proliferation, epithelial-to-mesenchymal transition (EMT) process, and metastatic capabilities of trophoblastic cells; whereas, miR–143–3p inhibition exert opposite effects. MiR–143–3p targeted S100A11 and was adversely regulated by circFOXP1 expression. S100A11 inhibition partially offset the effect of miR–143–3p knockdown on trophoblastic cell viability, apoptosis, EMT, invasion, and migration. In addition, circFOXP1 competitively combined with miR–143–3p, thus regulating S100A11 expression. Moreover, circFOXP1 regulated trophoblastic cell functions through the miR–143–3p/S100A11 cascade. To sum up, our study, for the first time, demonstrated that circFOXP1 could improve dysfunction of trophoblastic cells through the miR–143–3p/S100A11 axis, providing novel biomarkers and diagnostic targets for RPL.
Introduction
As a common pregnancy complication, recurrent pregnancy loss (RPL) refers to two or more consecutive miscarriages before 24 gestational weeks and affects almost 5% of women at reproductive age worldwide [Citation1,Citation2]. After several decades of research, anatomical, genetic, hormonal, environmental, psychological, and immune factors are broadly recognized as major identifiable causes for RPL [Citation3]. Given the complex pathogenesis of RPL, almost 50% of RPL cases remain unresolved or even unexplained in pathophysiology [Citation4]. Hence, it is imperative to unearth novel biomarkers for RPL diagnosis and therapy.
Trophoblast cells exert various biological functions during pregnancy [Citation5]. Deficiency in functions, ectopic differentiation, proliferation, migration, invasion, and apoptosis of trophoblastic cells could provide a cytological basis for pregnancy-associated complications, including RPL [Citation5,Citation6]. Besides, epithelial-to-mesenchymal transition (EMT), a process featured with loss of epithelial polarity and adhesiveness and acquisition of mesenchymal features, plays a vital part in regulating the biological behaviors of trophoblastic cells in human placental development [Citation7]. Therefore, inhibited trophoblastic cell activities and EMT process are closely correlative to RPL pathogenesis.
MicroRNAs (miRNAs) are small non-coding RNAs (with about 20–24 nucleotides) that bind to 3ʹ‐untranslated regions (UTR) of target messenger RNAs (mRNAs) and post-transcriptionally regulate their gene expressions by either promoting transcript or inhibiting translation [Citation8–11]. It has been disclosed by previous studies that aberrant miRNA expression is closely relative to reproductive system diseases, including gestational diabetes mellitus (GDM) [Citation12], preeclampsia [Citation13], and endometriosis [Citation14]. Also, a variety of miRNAs were found to be upregulated and inhibit trophoblastic cell activities in RPL. For instance, Wang et al. uncovered that miR–149 inhibited trophoblastic cell activity and promoted trophoblastic cell apoptosis in RPL through the PTEN/Akt pathway via targeting RUNX2 [Citation15]. Ding et al. revealed that miR–27a–3p-induced USP25 suppression inhibited EMT, migration, and invasion of trophoblastic cells in RPL [Citation16]. Dong et al. demonstrated that miRNA–520 promoted DNA-damage-caused apoptosis in trophoblastic cells via regulating PARP1 in RPL [Citation17]. In a former report from Bahia et al., miR–143–3p was identified as an upregulated miRNA in RPL patients, in comparison with healthy controls [Citation18]. Therefore, it was assumed that miR–143–3p might participate in RPL pathogenesis.
Circular RNAs (CircRNAs) are characterized by their covalently closed-loop structure and lack of free 3′ and 5′ ends [Citation19]. CircRNAs could modulate gene expression via absorbing miRNAs and play essential roles in various physiological and pathophysiological processes [Citation20,Citation21]. Also, circRNAs have been demonstrated to exert important roles in regulating biological processes in multiple diseases, including RPL. For example, Li et al. revealed that circZUFSP regulated trophoblastic cell migration and invasion via the miR-203/STOX1 pathway in RPL [Citation22]. Wu et al. disclosed that high circ_0054633 expression was observed in GDM and correlative to glycosylation index [Citation23]. Besides, Ou et al. found that circ_0111277 aggravated attenuates human trophoblast cell dysfunction via the miR–494/HTRA1/Notch-1 pathway in preeclampsia [Citation24]. Moreover, in a former study by Wang et al., circRNA forkhead box P1 (circFOXP1) was determined down-regulated in GDM by Wang et al. [Citation25], suggesting its potential regulatory function in RPL.
In this work, we aimed to inquire about the functions and the underlying mechanism of circFOXP1 and miR–143–3p in RPL. Through in vitro assays, it was demonstrated that circFOXP1 upregulated S100A11 expression via targeting miR–143–3p, thus regulating viability, apoptosis, migration, invasion, and EMT process of trophoblastic cells. The current study would provide novel biomarkers for RPL diagnosis and prognosis.
Material and methods
Patients and clinical samples
28 RPL patients and 28 age-matched healthy pregnant women (without histories of pregnancy loss, spontaneous abortion, preterm labor, or PE and with at least one live birth) were enrolled in this work. The detailed clinicopathological data of the participants were as shown in . Every participant provided informed consent. The Ethics Committee of The First People’s Hospital of Lianyungang permitted this study. Normal placental tissues were collected from healthy pregnant women accepting induced abortion to terminate unplanned pregnancy within 6 to 12 gestational weeks. RPL placental tissues were collected from RPL patients requiring termination. All placental tissue samples collected were frozen and kept in liquid nitrogen immediately for the following experiments.
Table 1. Clinicopathological features of subjects
Cell culture and transfection
HTR8/SVneo, a human extravillous trophoblastic cell line, was obtained from BeNa Culture Collection (Beijing, China) and cultivated in DMEM/F12 containing 10% FBS (5% CO2; 37°C).
MiR-143-3p mimics (5′-UGAGAUGAAGCACUGUAGCUC-3′), miR-143-3p inhibitor (5′-GAGCUACAGUGCUUCAUCUCA-3′), and corresponding negative controls (NC mimics: 5′-UCACAACCUCCUAGAAAGAGUAGA-3′; and NC inhibitor: 5′-UACUCUUUCUAGGAGGUUGUGAUU-3′), pcDNA3.1/circFOXP1 (oe-circFOXP1), pcDNA3.1 empty vector (Vector), small interfering RNA (siRNA) targeting S100A11 (si-S100A11: 5′-GACAGAGUUCCUAAGCUUCUU-3′), circFOXP1 (si-circFOXP1: 5′-UGAAGUCUGCCGGCUUGAG-3′), and corresponding negative control (si-NC: 5′-UUCUCCGAACGUGUCACGUUU-3′) were bought from GenePharma (Shanghai, China). HTR8/SVneo cells were transfected with the above plasmids via Lipofectamine 2000 reagent (Invitrogen), cultivated for 24 h, and then collected for subsequent experiments.
RT-qPCR
Total RNA was obtained with RNA Extracting Kit (Takara) from tissues or cells. Then, TaqMan microRNA Reverse Transcription Kit (Applied Biosystems) was utilized to synthesize cDNA for miRNA; PrimeScript RT Master Mix (Takara) was applied for reverse transcription of circRNA and mRNA. Thereafter, qPCR was performed with SYBR Green PCR Master Mix (Takara) on Light Cycler 480 II Real-Time PCR System (Roche, Switzerland). The relative gene levels were calculated by 2−ΔΔCt method, with miRNA expression normalized to U6, and circRNA and mRNA expressions normalized to GAPDH. The primers used were also listed: circFOXP1 Forward (F): 5′-CTCCTCTGCACCTTCCAAGA-3′, Reverse (R): 5′-ATCATAGCCACTGACACGGG-3′; miR-143-3p (F): 5′-CTGGCGTTGAGATGAAGCAC-3′, (R): 5′-CAGAGCAGGGTCCGAGGTA-3′; U6 F: 5′-CCTGCGCAAGGATGAC-3′, R: 5′-GTGCAGGGTCCGAGGT-3′; S100A11 F: 5ʹ-ACCGCATGATGAAGAAACTGG-3ʹ, R: 5ʹ-TGATGACAGAAAGGCTGGAAG-3ʹ; E-cadherin F: 5ʹ-ATTTTTCCCTCGACACCCGAT-3ʹ, R: 5ʹ-TCCCAGGCGTAGACCAAGA-3ʹ; Vimentin F: 5ʹ-AGTCCACTGAGTACCGGAGAC-3ʹ, R: 5ʹ-TCCAGCAGCTTCCTGTAGGT-3ʹ; N-cadherin F: 5ʹ-TGCGGTACAGTGTAACTGGG-3ʹ, R: 5ʹ-GAAACCGGGCTATCTGCTCG-3ʹ; GAPDH F: 5′-CCAGCCGAGCCACATCGCTC-3′, R: 5′-ATGAGCCCCAGCCTTCTCCAT-3ʹ.
Western blotting
Total protein was obtained from cells via RIPA buffer. Afterward, protein samples of equivalent amount (20 μg) were subject to 10% SDS-PAGE and transferred onto a PVDF membrane. Then, PVDF membrane was blocked with 5% skimmed milk for 2 h, cultivated with the primary antibodies against S100A11 (ab169530, Abcam), E-cadherin (ab40772, Abcam), N-cadherin (ab76057, Abcam), vimentin (ab20346, Abcam), or GAPDH (ab181602, Abcam), and then cultivated with secondary antibody (ab6721, Abcam). The protein expression was analyzed by Image J.
CCK-8
The transfected HTR8/SVneo cells were cultivated in 96-well plates (3 × 103 cells/well). 10 μL CCK-8 reagent was supplemented into each well at the indicated time points. The absorbance was observed with a microplate reader (Bio-Rad, USA) at 450 nm [Citation26].
Flow cytometry
Flow cytometry assay performed with Annexin V-FITC Apoptosis Assay Kit was applied for apoptosis analysis. Firstly, the transfected cells were digested by trypsin, centrifuged at 1000 rpm, and then resuspended and dispersed to the density of 1 × 106 cells/mL. Thereafter, the cells were labeled with Annexin-V-FITC as well as PI reagents in darkness. Finally, the cell apoptosis was analyzed with a flow cytometer (BD Biosciences, USA) [Citation27].
Wound healing assay
Cell migrative capability was detected by Wound healing. Briefly, HTR8/SVneo cells were plated to 12-well plates and cultivated till the bottom of each well was fully covered by cells. Then, a 200 μL pipette tip was adopted to make a vertical wound in each well. The scratched cells were removed with PBS. Thereafter, the plates were incubated for another 24 h. The wounds were photographed with an optical microscope at 0 and 24 h to analyze the wound closure condition of each group [Citation28].
Transwell assay
Cell invasion was analyzed via transwell chambers equipped with Matrigel-precoated transwell inserts (BD Biosciences). Firstly, cells (2 × 104 cells/well) were seeded into the upper compartment containing 200 μl FBS-free medium; meanwhile, 600 μL medium containing 10% FBS was supplemented to the lower compartment. Thereafter, cells were cultivated at 37°C for 24 h. The invaded cells were fixed and stained in 4% paraformaldehyde and 0.1% crystal violet. The invaded cells were counted with a microscope (Olympus) [Citation29].
Dual-luciferase reporter assay
CircFOXP1 or S100A11 fragments containing latent binding sites or corresponding mutant binding regions for miR–143–3p were cloned into pmirGLO luciferase reporter vectors (Promega) to form wild-type (circFOXP1-WT; S100A11-WT) or mutant (circFOXP1-MUT; S100A11-MUT) plasmids. Then, miR–143–3p mimics or NC mimics, and these reporter plasmids were co-transfected into HTR8/SVneo cells, respectively. Afterward, luciferase activity was tested by Dual-luciferase reporter assay system (Promega); meanwhile, Renilla luciferase activity was applied to normalize firefly luciferase activity.
Statistical analysis
GraphPad Prism 6.0 was adopted for all statistical analyses. Data in the work (exhibited as mean ± SD) were acquired from three independent experiments. Student’s t-test or one-way ANOVA was performed to analyze the significance of differences. Differences were regarded as significant in statistics when P-value <0.05.
Results
Here, we intended to investigate the role of miR–143–3p and circFOXP1 in RPL and their specific mechanism in regulating trophoblastic cell dysfunction. A series of in vitro assays demonstrated that circFOXP1 relieved trophoblastic cell dysfunction via targeting the miR–143–3p/S100A11 pathway, providing novel targets for RPL.
MiR–143–3p is lifted in RPL and impairs the biological functions of trophoblastic cells
According to , the subjects showed no remarkable difference in maternal age or gestational period. To explore the functions of miR–143–3p in RPL progression, RT-qPCR was applied to assess miR–143–3p levels in 28 placental tissue specimens from RPL patients and 28 placental tissue specimens from healthy pregnant women. The miR–143–3p level was prominently upregulated in placental tissues collected from RPL patients, compared with patients in the control group (), indicating miR–143–3p may be a new factor associated with RPL pathogenesis.
Figure 1. MiR–143–3p is lifted in RPL and impairs the biological functions of trophoblastic cells. (a) Relative miR–143–3p expression in placental tissues from RPL patients (n = 28) and normal pregnant women (n = 28) was detected by RT-qPCR. (b) miR–143–3p expression in HTR8/SVneo cells transfected with NC mimics, NC inhibitor, or miR–143–3p inhibitor was detected by RT-qPCR. (c) The cell viability of transfected HTR8/SVneo cells was analyzed by CCK-8 assay at different time points. (d) The apoptosis condition of transfected HTR8/SVneo cells was analyzed by flow cytometry assay. (e) The migration capability of transfected HTR8/SVneo cells was analyzed by wound healing assay. (f) The invasion capability of transfected HTR8/SVneo cells was analyzed by transwell assay. (g and h) The mRNA or protein expression level of EMT markers (E-cadherin, vimentin, and N-cadherin) in transfected HTR8/SVneo cells was determined by RT-qPCR or western blotting. Data are shown as the mean ± SD (n = 3). *P < 0.05; **P < 0.01
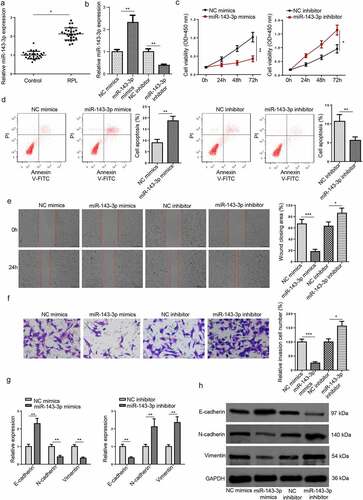
To probe the role of miR–143–3p in trophoblastic cell functions, miR–143–3p was firstly knocked down or overexpressed in HTR8/SVneo cells via transfection with miR-143-3p mimics or miR–143–3p inhibitor. The efficiencies of miR–143–3p overexpression or miR–143–3p interference were evaluated by RT-qPCR (). CCK-8 results revealed that miR-143-3p upregulation inhibited HTR8/SVneo cell viability, while miR–143–3p inhibition promoted HTR8/SVneo cell proliferation (). Besides, flow cytometry assay showed that miR–143–3p addition induced apoptosis in HTR8/SVneo cells; contrarily, miR–143–3p silencing reduced the apoptotic rate of HTR8/SVneo cells (). As indicated by wound healing assay, the number of migrated HTR8/SVneo cells was apparently decreased after miR–143–3p overexpression, while miR–143–3p inhibition significantly enhanced the migrative capability of HTR8/SVneo cells (). It was also revealed by transwell assay that miR–143–3p overexpression remarkably increased the number of invaded HTR8/SVneo cells; however, miR–143–3p interference significantly boosted the invasive ability ().
Considering the influence of miR–143–3p on EMT process of trophoblastic cells, the mRNA and protein levels of EMT markers (E-cadherin, vimentin, and N-cadherin) were determined by RT-qPCR and Western blot assays in trophoblastic cells. As indicated in (), miR–143–3p amplification down-regulated N-cadherin and vimentin levels but up-regulated E-cadherin level in HTR8/SVneo cells, whereas miR–143–3p interference showed the opposite effects. To sum up, these results demonstrated that miR–143–3p inhibited trophoblastic cell viability, induced trophoblastic cell apoptosis, and impaired trophoblastic cell invasion and migration by repressing EMT process.
S100A11 is directly targeted by miR–143–3p in trophoblastic cells
To expound the underlying molecular mechanisms involved in the suppressive effects on HTR8/SVneo cell viability, migration, and invasion mediated by miR–143–3p, three databases (miRanda, miRmap, and PITA) were applied to predict target genes of miR–143–3p under the following conditions: CLIP Data: high stringency (≥3), Degradome Data: high stringency (≥3). As indicated in (), 8 candidate genes (NXF1, SRSF11, COL5A1, S100A11, ARL15, PTGES3, PDIA6) were screened out. According to a previous study, the protein level of S100A11 was distinctly downregulated in placental tissues obtained from patients with early pregnancy loss [Citation30], indicating S100A11 may be an important regulator implicated in the pathogenesis of pregnancy loss. Besides, RT-qPCR revealed that S100A11 mRNA expression was significantly decreased in placental tissues from RPL group, relative to the control group (). Hence, S100A11 was selected for subsequent experiments. StarBase website provided the potential binding region between miR–143–3p and S100A11 3′-UTR (). Dual-luciferase reporter assay results exhibited that miR–143–3p amplification attenuated the reporter activity of S100A11-WT; however, S100A11-MUT showed no apparent response to the variation of miR–143–3p expression (). S100A11 mRNA expression was adversely relative to miR-143-3p abundance in placental tissues collected from RPL patients, as indicated by Pearson’s correlation analysis (). Furthermore, both mRNA and protein levels of S100A11 were declined in HTR8/SVneo cells after miR–143–3p overexpression; conversely, miR–143–3p blocking unregulated S100A11 mRNA and protein levels (). Therefore, the results indicated that miR–143–3p could oppositely regulate S100A11 expression in trophoblastic cells.
Figure 2. S100A11 is directly targeted by miR–143–3p in trophoblastic cells. (a) 8 candidate target mRNAs for miR–143–3p were predicted by using three miRNA databases (miRanda, miRmap, and PITA). (b) Relative S100A11 expression in placental tissues from RPL patients (n = 28) and normal pregnant women (n = 28) was detected by RT-qPCR. (c) The putative binding sites between miR–143–3p and S100A11. (d) The HTR8/SVneo cells were co-transfected with either S100A11-WT or S100A11-MUT, and miR–143–3p mimics/inhibitor or corresponding NC. Then, the relative luciferase activity was measured. (e) Pearson’s correlation analysis indicated a negative correlation between the expression of miR–143–3p and S100A11 in placental tissues from RPL patients (n = 28). (f and g) S100A11 mRNA or protein level in HTR8/SVneo cells transfected with NC mimics, miR–143–3p mimics, NC inhibitor or miR–143–3p inhibitor was detected by RT-qPCR or western blotting. Data are shown as the mean ± SD (n = 3). *P < 0.05; **P < 0.01
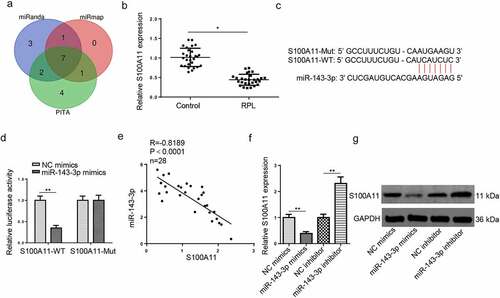
MiR–143–3p weakens trophoblastic cell functions via S100A11
To further probe into the role of the miR–143–3p/S100A11 axis in modulating trophoblastic cell functions, HTR8/SVneo cells were transfected with NC inhibitor, miR–143–3p inhibitor, miR–143–3p inhibitor+si-NC, or miR–143–3p inhibitor+si-S100A11. Firstly, the efficiency of S100A11 knockdown was assessed by RT–qPCR and Western blotting (). The findings manifested that the impacts of miR–143–3p inhibition on the proliferation, apoptosis, invasion, and migration of HTR8/SVneo cells were partly reversed by S100A11 blocking (). Moreover, the alterations on S100A11 mRNA and protein levels, as well as EMT markers induced by miR–143–3p interference were partially abrogated by S100A11 silencing (). To sum up, the above data indicated that miR–143–3p impaired trophoblastic cell functions through regulating S100A11.
Figure 3. MiR–143–3p weakens trophoblastic cell functions via S100A11. (a and b) S100A11 mRNA or protein level in HTR8/SVneo cells transfected with si-NC or si-S100A11 was detected by RT-qPCR or western blotting. (c) HTR8/SVneo cells transfected with NC inhibitor, miR–143–3p inhibitor, miR–143–3p inhibitor+si-NC, or miR–143–3p inhibitor+si-S100A11, respectively. the cell viability of transfected HTR8/SVneo cells was analyzed by CCK-8 assay at different time points. (d) The apoptosis condition of transfected HTR8/SVneo cells was analyzed by flow cytometry assay. (e) The migration capability of transfected HTR8/SVneo cells was analyzed by wound healing assay. (f) The invasion capability of transfected HTR8/SVneo cells was analyzed by transwell assay. (g and h) The mRNA or protein expression level of S100A11 and EMT markers (E-cadherin, vimentin, and N-cadherin) in transfected HTR8/SVneo cells were determined by RT-qPCR or western blotting. data are shown as the mean ± SD (n = 3). *P < 0.05; **P < 0.01
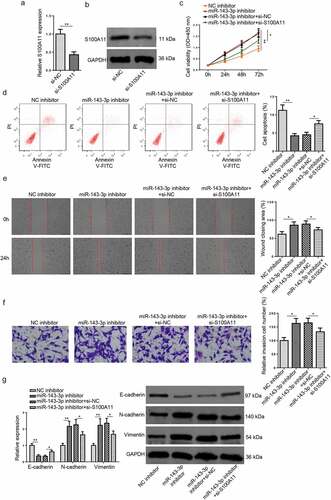
CircFOXP1 acts as a miR–143–3p ceRNA in trophoblastic cells
With the StarBase website, a binding sequence between circFOXP1 and miR–143–3p was predicted (). The results of luciferase reporter assay exhibited that the luciferase activity of circFOXP1-WT was decreased after miR–143–3p overexpression; nevertheless, the luciferase activity of FOXD3–AS1–MT was almost unchanged (). RT-qPCR verified that circFOXP1 abundance was markedly decreased in placental tissues from RPL group, relative to the control group (). Then, RT–qPCR was applied for assessment of circFOXP1 knockdown or overexpression efficiency (). The miR–143–3p level was negatively related to circFOXP1 level in RPL tissues (); moreover, miR–143–3p level was reduced after circFOXP1 overexpression and upregulated after circFOXP1 knockdown (), indicating circFOXP1 could negatively regulate miR–143–3p expression. Besides, S100A11 mRNA expression was positively correlative to circFOXP1 level in placental tissues collected from RPL patients (). Furthermore, RT-qPCR and western blotting manifested that circFOXP1 overexpression elevated S100A11 mRNA and protein expressions, while circFOXP1 silencing reduced S100A11 mRNA and protein levels (). These data revealed that circFOXP1 functioned as a miR–143–3p ceRNA to modulate S100A11 level in trophoblastic cells.
Figure 4. CircFOXP1 binds to miR–143–3p in trophoblastic cells. (a) The putative binding sites between miR–143–3p and circFOXP1. (b) The HTR8/SVneo cells were co-transfected with either circFOXP1-WT or circFOXP1-MUT, and miR–143–3p mimics or NC mimics. then, the relative luciferase activity was measured. (c) Relative S100A11 expression in placental tissues from RPL patients (n = 28) and normal pregnant women (n = 28) was detected by RT-qPCR. (d) CircFOXP1 expression in HTR8/SVneo cells transfected with si-NC or si-circFOXP1 was detected by RT-qPCR. (e) CircFOXP1 expression in HTR8/SVneo cells transfected with vector or oe-circFOXP1was detected by RT-qPCR. (f) Pearson’s correlation analysis indicated a negative correlation between the expression of miR–143–3p and circFOXP1 in placental tissues from RPL patients (n = 28). (g) miR–143–3p expression in HTR8/SVneo cells transfected with si-NC, si-circFOXP1, Vector, or oe-circFOXP1 was detected by RT-qPCR. (h) Pearson’s correlation analysis indicated a positive correlation between S100A11 mRNA expression and circFOXP1 expression in placental tissues from RPL patients (n = 28). (i and j) S100A11 mRNA or protein level in HTR8/SVneo cells transfected with si-NC, si-circFOXP1, Vector, or oe-circFOXP1 was detected by RT-qPCR or western blotting. data are shown as the mean ± SD (n = 3). *P < 0.05; **P < 0.01
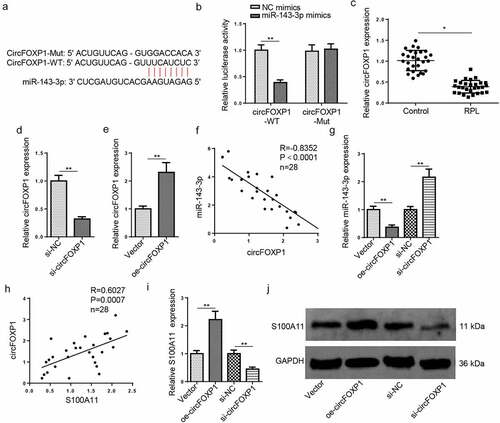
CircFOXP1/miR–143–3p/S100A11 axis regulates trophoblastic cell functions
To further determine the influence of circFOXP1/miR–143–3p/S100A11 axis on trophoblastic cell functions, the proliferation, apoptosis, migration, invasion, and EMT of trophoblastic cells in each group. As shown in (), circFOXP1 overexpression increased viability, reduced apoptosis, and promoted migration and invasion of HTR8/SVneo cells; whereas, miR–143–3p upregulation partly offset such impact. Furthermore, the influence of circFOXP1 upregulation on mRNA and protein expression levels of S100A11, as well as EMT markers was partially neutralized by miR–143–3p overexpression (). Overall, these data indicated that circFOXP1 regulated S100A11 via miR–143–3p expression, thus improving trophoblastic cell dysfunction.
Figure 5. CircFOXP1/miR–143–3p/S100A11 axis regulates trophoblastic cell functions. (a) HTR8/SVneo cells transfected with vector, oe-circFOXP1, oe-circFOXP1+NC mimics, or oe-circFOXP1+ miR–143–3p mimics, respectively. The cell viability of transfected HTR8/SVneo cells was analyzed by CCK-8 assay at different time points. (b) The apoptosis condition of transfected HTR8/SVneo cells was analyzed by flow cytometry assay. (c) The migration capability of transfected HTR8/SVneo cells was analyzed by wound healing assay. (d) The invasion capability of transfected HTR8/SVneo cells was analyzed by transwell assay. (e and f) The mRNA or protein expression level of S100A11 and EMT markers (E-cadherin, vimentin, and N-cadherin) in transfected HTR8/SVneo cells were determined by RT-qPCR or western blotting. data are shown as the mean ± SD (n = 3). *P < 0.05; **P < 0.01
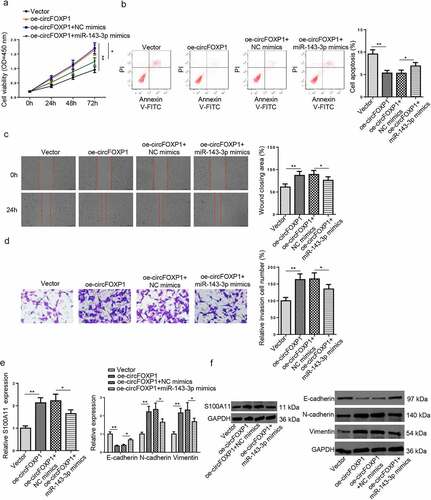
Discussion
Multitudinous miRNAs were anomalously expressed in the placental tissues or plasma of RPL patients, in contrast with normal pregnancies [Citation31]. In this work, we proved that miR–143–3p was manifestly upregulated in the placental tissues from RPL patients, in comparison with healthy controls. The results implied that elevated miR–143–3p level may be correlative to RPL pathogenesis.
It has been demonstrated that miR–143–3p increased cell apoptosis but suppressed cell viability, EMT process, cell migration, and invasion in multiple diseases. For example, Sun et al. elaborated that miR–143–3p increased apoptotic rate and attenuated cell viability in an in vitro model of Alzheimer’s disease via interaction with NRG1 [Citation32]. Peng et al. found that miR–143–3p induced cell apoptosis, and suppressed cell proliferation, EMT, migration, and invasion in hepatocellular carcinoma [Citation33]. MiR–143–3p upregulation is also related to other pregnancy-associated complications, including gestational diabetes mellitus [Citation34] and gestational hypertension [Citation35]. Consistent with the above studies, the functional experiments further manifested that miR–143–3p suppressed proliferative capability but accelerated apoptosis in trophoblastic cells; contrarily, miR–143–3p inhibition reduced apoptosis but enhanced proliferation of trophoblastic cells. EMT is deeply involved in the pathological and physiological processes of various cells [Citation36]. Besides, a previous study demonstrated that inhibition of EMT process would impair the migrative and invasive capability of trophoblastic cells and finally result in RPL [Citation37]. Herein, the results indicated that miR– 143–3p addition restrained trophoblastic cell invasion and migration via inhibiting EMT. Therefore, miR–143–3p promoted trophoblastic cell dysfunction via regulating proliferation, invasion, migration and EMT process in trophoblastic cells.
S100A11, belonging to the S100 family, is closely related to cell viability, apoptosis, migration, invasion, and EMT in many diseases [Citation38,Citation39]. For instance, Xia et al. revealed S100A11 reduced neuronal cell apoptosis caused by cerebral ischemia [Citation40]. Tu et al. elucidated that S100A11 facilitated stem cell growth, EMT, migration, invasion, and generation in glioma [Citation41]. In our study, S100A11 was lowly expressed in RPL. S100A11 was directly targeted by miR–143–3p. Moreover, S100A11 level was lowly expressed and negatively correlative to miR–143–3p abundance in RPL. Also, S100A11 enrichment was adversely modulated by miR–143–3p in trophoblastic cells. More importantly, rescue assays further unearthed that S100A11 knockdown partly reversed the impacts of miR–143–3p suppression on proliferation, apoptosis, invasion, migration, and EMT process of trophoblastic cells. Mechanistically, miR–143–3p impaired trophoblastic cell functions by negatively regulating S100A11 in RPL.
CircRNAs functioned as competitive endogenous RNAs (ceRNAs) to modulate gene levels via miRNAs [Citation42]. CircFOXP1 is involved in the progression of multiple diseases, including osteoporosis [Citation43]. Moreover, Wang et al. disclosed that circFOXP1 promoted gallbladder cancer cell proliferation, migration, and invasion [Citation44]. Li et al. found circFOXP1 facilitated cell proliferation and reduced apoptosis in lung carcinoma [Citation45]. Herein, we confirmed that circFOXP1 expression was downregulated in RPL. In addition, circFOXP1 expression was negatively correlative to miR–143–3p abundance and positively related to S100A11 enrichment in RPL. Also, circFOXP1 promoted S100A11 expression as a ceRNA for miR–143–3p in trophoblastic cells. CircFOXP1 overexpression facilitated trophoblastic cell functions and promoted EMT process, which was partly offset by miR–143–3p upregulation. Therefore, circFOXP1 improved trophoblastic cell functions via interaction with miR–143–3p.
Conclusion
In summary, this study disclosed that circFOXP1 could enhance proliferation, restrain apoptosis, and facilitate EMT-associated migration and invasion of trophoblastic cells via modulating the miR–143–3p/S100A11 axis, providing potential biomarkers for RPL diagnosis. Nevertheless, our study is still limited as functional assays were only performed in vitro. In the future, further investigation would be conducted in vivo.
Research highlights
MiR–143–3p expression was elevated in RPL and correlative to trophoblastic cell dysfunction.
MiR–143–3p impaired trophoblastic cell functions via targeting S100A11.
CircFOXP1 improved trophoblastic cell functions via the miR–143–3p/S100A11 pathway.
Authors’ contributions
YG and YT designed this study. QS and GG performed all the experiments. YG and ZZ analyzed the data and prepared the figures. YG, XW and FS drafted the initial manuscript. WY reviewed and revised the manuscript. All authors read and approved the final manuscript.
Ethics approval and consent to participate
The Ethics Committee of The First People’s Hospital of Lianyungang permitted this study.
Disclosure statement
No potential conflict of interest was reported by the author(s).
Data availability statement
The datasets used and/or analyzed during the current study are available from the corresponding author on reasonable request.
Additional information
Funding
References
- Krieg SA, Fan X, Hong Y, et al. Global alteration in gene expression profiles of deciduas from women with idiopathic recurrent pregnancy loss. Mol Hum Reprod. 2012;18(9):442–450.
- Baek KH, Lee EJ, Kim YS. Recurrent pregnancy loss: The key potential mechanisms. Trends Mol Med. 2007;13(7):310–317.
- Garrido-Gimenez C, Alijotas-Reig J. Recurrent miscarriage: Causes, evaluation and management. Postgrad Med J. 2015;91(1073):151–162.
- Liu Z, Xu H, Kang X, et al. Allogenic Lymphocyte immunotherapy for unexplained recurrent spontaneous abortion: A meta-analysis. Am J Reprod Immunol Microbiol N Y. 2016;76(6):443–453
- Zhang Y, Zhou J, Li MQ, et al. MicroRNA-184 promotes apoptosis of trophoblast cells via targeting WIG1 and induces early spontaneous abortion. Cell Death Dis. 2019;10(3):223.
- Laird SM, Tuckerman EM, Cork BA, et al. A review of immune cells and molecules in women with recurrent miscarriage. Hum Reprod Update. 2003;9(2):163–174.
- Mayhew TM. Turnover of human villous trophoblast in normal pregnancy: what do we know and what do we need to know? Placenta. 2014;35(4):229–240.
- Thompson RC, Deo M, Turner DL. Analysis of microRNA expression by in situ hybridization with RNA oligonucleotide probes. Methods. 2007;43(2):153–161.
- Bartel DP. MicroRNAs: Genomics, biogenesis, mechanism, and function. Cell. 2004;116(2):281–297.
- Sudhakara Prasad K, Cao X, Gao N, et al. A low-cost nanomaterial-based electrochemical immunosensor on paper for high-sensitivity early detection of pancreatic cancer. Sens Actuators B Chem. 2020;305:127516.
- Shin Low S, Pan Y, Ji D, et al. Smartphone-based portable electrochemical biosensing system for detection of circulating microRNA-21 in saliva as a proof-of-concept. Sens Actuators B Chem. 2020;308:127718.
- Chen SH, Liu XN, Peng Y. MicroRNA-351 eases insulin resistance and liver gluconeogenesis via the PI3K/AKT pathway by inhibiting FLOT2 in mice of gestational diabetes mellitus. J Cell Mol Med. 2019;23(9):5895–5906.
- Zhao G, Zhou X, Chen S, et al. Differential expression of microRNAs in decidua-derived mesenchymal stem cells from patients with pre-eclampsia. J Biomed Sci. 2014;21(1):81.
- Hsu CY, Hsieh TH, Tsai CF, et al. miRNA-199a-5p regulates VEGFA in endometrial mesenchymal stem cells and contributes to the pathogenesis of endometriosis. J Pathol. 2014;232(3):330–343.
- Wang P, Chen X, Chang Y, et al. Inhibition of microRNA-149 microRNA-149 protects against recurrent miscarriage through upregulating RUNX2 and activation of the PTEN/Akt PTEN /Akt signaling pathway. J Obstet Gynaecol Res. 2020;46(12):2534–2546.
- Ding J, Cheng Y, Zhang Y, et al. The miR-27a-3p/USP25 axis participates in the pathogenesis of recurrent miscarriage by inhibiting trophoblast migration and invasion. J Cell Physiol. 2019;234(11):19951–19963.
- Dong X, Yang L, Wang H. miR-520 promotes DNA-damage-induced trophoblast cell apoptosis by targeting PARP1 in recurrent spontaneous abortion (RSA). Gynecol Endocrinol. 2017;33(4):274–278.
- Bahia W, Soltani I, Abidi A, et al. Identification of genes and miRNA associated with idiopathic recurrent pregnancy loss: An exploratory data mining study. BMC Med Genomics. 2020;13(1):75.
- Chen LL, Yang L. Regulation of circRNA biogenesis. RNA Biol. 2015;12(4):381–388.
- Thomson DW, Dinger ME. Endogenous microRNA sponges: Evidence and controversy. Nat Rev Genet. 2016;17(5):272–283.
- Bi J, Liu H, Dong W, et al. Circular RNA circ-ZKSCAN1 inhibits bladder cancer progression through miR–1178–3p/p21 axis and acts as a prognostic factor of recurrence. Mol Cancer. 2019;18(1):133.
- Li Z, Zhou G, Tao F, et al. circ-ZUFSP regulates trophoblasts migration and invasion through sponging miR-203 to regulate STOX1 expression. Biochem Biophys Res Commun. 2020;531(4):472–479.
- Wu H, Wu S, Zhu Y, et al. Hsa_circRNA_0054633 is highly expressed in gestational diabetes mellitus and closely related to glycosylation index. Clin Epigenetics. 2019;11(1):22.
- Ou Y, Zhu L, Wei X, et al. Circular RNA circ_0111277 attenuates human trophoblast cell invasion and migration by regulating miR-494/HTRA1/Notch-1 signal pathway in pre-eclampsia. Cell Death Dis. 2020;11(6):479.
- Wang H, She G, Zhou W, et al. Expression profile of circular RNAs in placentas of women with gestational diabetes mellitus. Endocr J. 2019;66(5):431–441.
- Yao Y, Li X, Cheng L, et al. Circular RNA FAT atypical cadherin 1 (circFAT1)/microRNA–525–5p/spindle and kinetochore-associated complex subunit 1 (SKA1) axis regulates oxaliplatin resistance in breast cancer by activating the notch and Wnt signaling pathway. Bioengineered. 2021;12(1):4032–4043.
- Ge C, Zeng B, Li R, et al. Knockdown of STIM1 expression inhibits non-small-cell lung cancer cell proliferation in vitro and in nude mouse xenografts. Bioengineered. 2019;10(1):425–436.
- Shi Z, Shen C, Yu C, et al. Long non-coding RNA LINC00997 silencing inhibits the progression and metastasis of colorectal cancer by sponging miR–512–3p. Bioengineered. 2021;12(1):627–639.
- Wang X, Li T. Ropivacaine inhibits the proliferation and migration of colorectal cancer cells through ITGB1. Bioengineered. 2021;12(1):44–53.
- Liu AX, Jin F, Zhang WW, et al. Proteomic analysis on the alteration of protein expression in the placental villous tissue of early pregnancy loss. Biol Reprod. 2006;75(3):414–420.
- Qin W, Tang Y, Yang N, et al. Potential role of circulating microRNAs as a biomarker for unexplained recurrent spontaneous abortion. Fertil Steril. 2016;105(5):1247–1254 e1243.
- Sun C, Jia N, Li Z, et al. miR–143–3p inhibition promotes neuronal survival in an Alzheimer’s disease cell model by targeting neuregulin-1. Folia Neuropathol. 2020;58(1):10–21.
- Peng J, Wu HJ, Zhang HF, et al. miR–143–3p inhibits proliferation and invasion of hepatocellular carcinoma cells by regulating its target gene FGF1. Clin Transl Oncol. 2021;23(3):468–480.
- Hromadnikova I, Kotlabova K, Dvorakova L, et al. Diabetes mellitus and cardiovascular risk assessment in mothers with a history of gestational diabetes mellitus based on postpartal expression profile of MicroRNAs associated with diabetes mellitus and cardiovascular and cerebrovascular diseases. Int J Mol Sci. 2020;21(7):2437.
- Hromadnikova I, Kotlabova K, Dvorakova L, et al. Postpartum profiling of microRNAs involved in pathogenesis of cardiovascular/cerebrovascular diseases in women exposed to pregnancy-related complications. Int J Cardiol. 2019;291:158–167.
- Lamouille S, Xu J, Derynck R. Molecular mechanisms of epithelial-mesenchymal transition. Nat Rev Mol Cell Biol. 2014;15:178–196.
- Lv S, Wang N, Lv H, et al. The attenuation of trophoblast invasion caused by the downregulation of EZH2 is involved in the pathogenesis of human recurrent miscarriage. Mol Ther Nucleic Acids. 2019;14:377–387.
- Liu Y, Han X, Gao B. Knockdown of S100A11 expression suppresses ovarian cancer cell growth and invasion. Exp Ther Med. 2015;9(4):1460–1464.
- Ma G, Dai W, Zhang J, et al. ELK1-mediated upregulation of lncRNA LBX2-AS1 facilitates cell proliferation and invasion via regulating miR–491–5p/S100A11 axis in colorectal cancer. Int J Mol Med. 2021;48(1). DOI:10.3892/ijmm.2021.4971
- Xia Q, Li X, Zhou H, et al. S100A11 protects against neuronal cell apoptosis induced by cerebral ischemia via inhibiting the nuclear translocation of annexin A1. Cell Death Dis. 2018;9(6):657.
- Tu Y, Xie P, Du X, et al. S100A11 functions as novel oncogene in glioblastoma via S100A11/ANXA2/NF-κB positive feedback loop. J Cell Mol Med. 2019;23(10):6907–6918.
- Ashwal-Fluss R, Meyer M, Pamudurti NR, et al. circRNA biogenesis competes with pre-mRNA splicing. Mol Cell. 2014;56(1):55–66.
- Shen W, Sun B, Zhou C, et al. CircFOXP1/FOXP1 promotes osteogenic differentiation in adipose-derived mesenchymal stem cells and bone regeneration in osteoporosis via miR-33a-5p. J Cell Mol Med. 2020;24(21):12513–12524.
- Li T, Zhao P, Li Z, et al. miR-200c-3p suppresses the proliferative, migratory, and invasive capacities of nephroblastoma cells via targeting FRS2. Biopreserv Biobank. 2019;17(5):444–451.
- Li O, Kang J, Zhang JJ, et al. Circle RNA FOXP1 promotes cell proliferation in lung cancer by regulating miR–185–5p/Wnt1 signaling pathway. Eur Rev Med Pharmacol Sci. 2020;24:6767–6778.