ABSTRACT
Glaucoma, characterized with progressive degeneration of retinal ganglion cells (RGCs), is the second frequently leading cause of sight loss in the word after cataract. Baicalin plays a protective role in age-related macular degeneration, retinopathy of prematurity, branch retinal vein occlusion, and ischemia-induced neurodegeneration in the retina. The present study aimed to investigate the role of baicalin in glaucoma. RGCs were stimulated with N-methyl-D-aspartate (NMDA) to mimic the in vitro model of glaucoma. A mouse model of glaucoma induced by chronic elevated intraocular pressure was also established. The apoptosis, oxidative stress, and autophagy of RGCs were detected by flow cytometry analysis, 2,7-dichlorodihydrofluorescein diacetate staining, and Western blotting, respectively. Retinal pathological changes were exhibited by hemotoxylin and eosin staining. Baicalin restrained the NMDA-induced cell apoptosis, autophagy, and oxidative stress of RGCs by activating the PI3K/AKT signaling in vitro. The elevated intraocular pressure-induced pathological changes in retinas of glaucoma mice were attenuated by baicalin. Moreover, the number of RGCs was significantly decreased in glaucoma mice, and then increased by baicalin treatment. Baicalin also inhibited autophagy and activated PI3K/AKT signaling in vivo. In conclusion, baicalin suppresses glaucoma pathogenesis by regulating the PI3K/AKT signaling in vitro and in vivo.
Introduction
Glaucoma is a major cause of irreversible blindness around the world and is characterized by optic nerve injury and degeneration of the retinal ganglion cells (RGCs) [Citation1]. Glaucoma is asymptomatic in the early stage, but leads to decline in contrast and color sensitivity and progressive visual field defects in its advanced stage [Citation2]. In 2020, the number of people affected by glaucoma reached 4.5 million among global population with moderate or severe visual impairment [Citation3]. The etiology of glaucoma is attributed to multiple factors, including oxidative stress, retinal ischemia, nutritional status, cardiovascular diseases, diabetes mellitus, glutamate excitotoxicity as well as hemorheological abnormalities [Citation4,Citation5]. Aqueous humor is the clear liquid filled in the anterior and posterior chambers of the eye, and obstruction of aqueous humor circulation can be caused by the elevated intraocular pressure (EIOP) [Citation6]. As a receptor and protector of visual signals, RGCs play a crucial role in the transmission of visual information from the retina to the visual center of the brain [Citation7]. EIOP easily contributes to RGC loss, stress response, and apoptosis of RGCs, subsequently causing pathological changes of glaucoma [Citation8]. In addition, excessive autophagy and oxidative stress also can cause RGC apoptosis, indirectly leading to glaucoma [Citation9,Citation10]. Currently, the available approach for glaucoma treatment is to reduce IOP including surgical intervention, laser therapy, and medicine administration [Citation11]. However, some anti-glaucoma drugs are associated with adverse symptons on eyes, including xerophthalmia, burning, and tingling sensation [Citation12]. Therefore, identifying novel therapeutic strategies that can regulate RGC proliferation and IOP changes is important in glaucoma treatment and visual function recovery.
Baicalin is the dried root of Scutellaria baicalensis Georgi, a Chinese medicinal herb [Citation13]. Baicalin can inhibit inflammation, oxidative stress, suppress tumor growth, and protect nerves [Citation14]. Moreover, baicalin also can reduce serum cholesterol, capillary permeability, and blood pressure [Citation15]. Thus, since ancient times, baicalin has been considered as a very popular drug in the clinical application of various diseases, such as hyperlipemia, leukemia, hepatoma, and hepatitis [Citation16]. Multiple pharmacological properties of baicalin play important roles in the prevention and treatment of ocular diseases, such as glaucoma diabetic retinopathy age-related macular degeneration and cataract [Citation17]. Baicalein exerts antioxidant and anti-apoptotic effects to prevent retinal ischemia by upregulating heme oxygenase-1 and downregulating hypoxia-inducible factor-1α, vascular endothelium growth factor, and matrix metalloproteinase 9 [Citation18]. Baicalein is beneficial in cornea repair via inhibiting the production of platelet-activating factor [Citation19]. Baicalin significantly inhibits the activity of aldose reductase (the culprit for diabetic cataract) in rat lens [Citation20]. Baicalin dose-dependently exhibits protective effects against oxidative stress-induced RGC-5 injury, which indicates its beneficial role in preventing RGC loss in glaucoma [Citation21]. Therefore, to further elucidate pharmacologic actions of baicalin in glaucoma progression, relevant studies should be conducted.
Based on the above studies, we made a hypothesis that baicalin exerts a protective role in glaucoma by alleviating RGC injury. The role and underlying pathway of baicalin in the apoptosis, autophagy, and oxidative stress of NMDA-stimulated RGCs and in pathological changes on retinas of glaucoma mice were investigated, which may provide more insight into the treatment of glaucoma.
Materials and methods
Cell culture
RGC-5 cells were purchased from Fenghui Biotechnology (Changsha, China). The cells were cultured in Dulbecco’s modified Eagle medium (DMEM; Invitrogen, USA) supplemented with 10% fetal bovine serum (Invitrogen), 100 μg/mL of streptomycin (Invitrogen) and 100 U/mL of penicillin (Invitrogen) at 37°C with 5% CO2. Medium was changed every 3 days and RGC-5 cells at passage of 3–5 were used.
Cell treatment
NMDA at different concentrations (0, 50, 100, or 150 μmol/L) was used to treat RGC-5 cells for 30 min [Citation22]. Cells were then cultured with the original culture medium for another 24 h. Since 150 μmol/L of N-methyl-D-aspartate (NMDA) has the best promotive effects on cell injury and autophagy of RGC-5 cells, NMDA at the concentration of 150 μmol/L was chosen to treat RGC-5 cells in the following experiments. Subsequently, RGC-5 cells were assigned into four groups. Cells in group I are basal cells without other treatments. Cells in group II were treated with 150 μmol/L of NMDA for 30 min. Cells in group III were treated with 150 μmol/L of NMDA for 30 min and 10 μmol/L of Baicalin (Sigma Aldrich, St. Louis, MO, USA) for 24 h [Citation23]. 8-Phenyl-2-(morpholin-4-yl)-chromen-4-one (LY294002, formula: C₁₉H₁₇NO₃) is a broad-spectrum PI3K inhibitor. Cells in group IV were treated with 150 μmol/L of NMDA for 30 min followed by 10 μmol/L of baicalin and LY294002 (Calbiochem, La Jolla, CA, USA) for 24 h [Citation24].
Flow cytometry analysis
RGC-5 cells with different treatments were then digested, washed twice with ice-cold phosphate buffered saline (PBS), centrifuged for 5 minutes, and re-suspended in 200 μL of binding buffer. Next, cells were incubated with 10 μL Annexin V and 1 mg/mL propidium iodide (PI) (Beijing Biotech, China) in the dark at 20–25°C for 10 min. Apoptosis was quantified by flow cytometry (FACSCalibur; BD, New Jersey, USA) using Cell Quest Pro software (Beckman Coulter, Brea, CA, USA).
Methyl thiazolyl tetrazolium (MTT) assay
Cell viability was assessed using the MTT assay. Briefly, RGC-5 cells were seeded into 96-well plates at a density of 2 × 105 cells/well. RGC-5 cells with different treatments were incubated with MTT (0.5 mg/mL; Sigma-Aldrich) solution for 3 h. Medium was removed and dimethyl sulfoxide (DMSO; 200 μL) was added to each well. The optical density (OD) of each well was measured at a wavelength of 490 nm using a Multiskan Ascent Revelation Plate Reader (Thermo Fisher Scientific, Waltham, MA, USA) and the data are normalized to cell absorbance without NMDA stimulation.
Measurement of reactive oxygen species (ROS)
Intracellular ROS accumulation was measured using 2,7-dichlorodihydrofluorescein diacetate (H2DCF-DA). Briefly, after different treatments, RGC-5 cells were washed and then stained with 10 μmol/L H2DCF-DA (Sigma-Aldrich) in serum-free medium for 30 minutes at 37°C in the dark. The cells were photographed using a fluorescence microscope (Olympus, Tokyo, Japan).
Estimation of malondialdehyde (MDA)
MDA reacts with thiobarbituric acid to produce a fluorescent product that can be measured using a microplate reader (Thermo Fisher Scientific, Waltham, MA, USA) at a wavelength of 535 nm. After different treatments, RGC-5 cells were harvested, and MDA levels were determined using an MDA detection kit from Nanjing Jiancheng Bioengineering Institute (Nanjing, China) according to the manufacturer’s instructions.
Animal grouping
C57BL/6 mice were divided into four groups (n = 10/group): control, model, Model+normal saline (NS), and Model+BAI (BAI refers to baicalin). Mice in the Model+BAI group were intraperitoneally injected with baicalin (50 mg/kg [Citation23]) once a day for 15 days, and mice in the Model+NS group were intraperitoneally injected by identical doses of NS once a day for 15 days. Injections of baicalin or NS were performed after the successful establishment of the mouse model of glaucoma. Finally, all mice in different groups were killed by neck dislocation and the right eyeballs were stored in the refrigerator at −80°C for later experiments. All the animal experiments were approved by the Ethics Committee of Henan Provincial People’s Hospital (DWLL202110011; Henan, China).
Establishment of a mouse model of glaucoma
C57BL/6 healthy mice (male, 8–10 weeks, weighing 25 g ± 2 g) were purchased from Jiangsu ALF Biotechnology (Nanjing, China). All mice were kept under the 12-h day/night cycle and fed with standardized pellet feed at 20°C. For this experiment, 30 mice were randomly divided into the glaucoma group, whereas the remaining 10 mice were in the control group (negative control). As previously described [Citation25], episcleral venous occlusion with cauterization was used to establish a mouse model of glaucoma with chronic EIOP. Mice in the glaucoma group were intraperitoneally injected with 1% pentobarbital sodium (P3761, 50 mg/kg, Sigma Aldrich, USA) for general anesthesia and pentobarbital sodium was used to dropwise anesthetize their ocular surfaces. The bulbar conjunctiva at the limbus of the cornea was cut off in the supratemporal, supratemporal, and suprasal quadrants. After separation of the extraocular muscle and fascia, three proximal external scleral veins were burned with a sterile needle, resulting in congestion and whitening of the proximal and distal veins. The left eye of the mice served as the untreated control. After the surgery, the mixture of saline and gentamicin (G3632; Sigma-Aldrich Chemical Company, USA) was applied to wash the conjunctival sac. Erythromycin ophthalmic ointment (Chen Xin Pharmaceutical Co., Ltd., Beijing, China) was used to treat the eye area 3 times a day for 3 consecutive days to relieve inflammatory response. Next, the IOP of mice in the control group and glaucoma group was measured by Goldmann applanation tonometer (Shanghai Precision Scientific Instruments Co., Ltd., Shanghai, China) at 0, 3, 7, 14, 21, 28 and 42 days. After 42 days, the model is considered successfully built only when the average IOP is observed to remain above 22 mmHg (1 mmHg = 0.133 kPa).
Hemotoxylin and eosin (H&E) staining
Eyes isolated from mice were fixed in 4% paraformaldehyde, dehydrated, paraffin-embedded, and sliced up. Next, the eye specimen was subjected to hematoxylin staining for 5 min and eosin staining for 2 min. After that, the stained eye specimen was washed in distilled water for 1 min. Gradient alcohol and xylene were used for dehydration and cleanout of the specimen. Morphological structure of eye specimen was observed via a light microscope (Olympus). Ganglion cell layer (GCL) thickness was quantified by the ImageJ software.
Counting of RGCs
The right eyeballs of each group were thoroughly rinsed twice with 0.1 M PBS, and the anterior segments 1 mm away from corneoscleral limbus were removed. The vitreous bodies were clipped with toothless forceps, and the retinas were bluntly separated with tip‐curved forceps. The eyeballs were fixed in 4% polyoxymethylene for 30 min at room temperature, washed with PBS three times for 10 min, and put into 30% sucrose solution overnight in a refrigerator at 4°C. Furthermore, the eyeballs were washed with PBS three times for 10 min and blocked with 10% normal donkey serum overnight in a refrigerator at 4°C. After the addition of primary antibody against brain‐specific homeobox/POU domain protein 3B (dilution 1:1,000, sc‐514,474; Santa Cruz, CA), the eyeballs were put in a refrigerator overnight at 4°C for 6 days and washed with PBS three times for 10 min. With the addition of the Alexa Fluor® 488 labeled donkey anti‐mouse secondary antibody immunoglobulin G (dilution 1:50, ab150105; Abcam, Cambridge, MA), the eyeballs were placed overnight in a refrigerator at 4°C and washed with PBS for 10 min. With retinal stretched preparation and vitreous body upward, the retinas were mounted, stored without light, and put into a refrigerator overnight for drying at 4°C. Moreover, the retinas were divided into four quadrants with optic nervous papilla in the center and nasal and temporal direction as the ordinate. Each quadrant chose three areas from the optic disc of 1/6, 1/2, 5/6 retinal radius; photos were taken using a fluorescent microscope. Afterward, the total number of RGCs in each field was calculated.
Western blotting
RIPA lysis buffer (Beyotime, Nanjing, China) was used to extract proteins from RGC-5 cells and retinal tissues of mice. BCA Protein Assay Kit (Thermo Fisher Scientific, USA) was applied to determine concentration of isolated proteins. Protein samples were loaded at 12% SDS-PAGE and then transferred onto PVDF membranes (Millipore, USA). Subsequently, the membranes were incubated with primary antibodies at 4°C overnight, which included anti-microtubule-associated protein 1 light chain 3B (LC3 B) (1:500; ab239416; Abcam), anti-Beclin-1 (1:500; ab210498; Abcam), anti-autophagy-related gene 5 (ATG5) (1:500; ab238092; Abcam), anti-phospho protein kinase B (pAKT) (1:500; ab38449; Abcam), anti-protein kinase B (AKT) (1:500; ab8805; Abcam), anti-phospho phosphoinositide 3-kinase (pPI3K) (p85, 1:1000; ab182651; Abcam), anti-phosphoinositide 3-kinase (PI3K) (p85; 1:1000; ab191606; Abcam) and β-actin (1:1000; ab8227; Abcam). LC3 is a ubiquitin-like molecule that is the mammalian homologue of the Atg8 encoded product in yeast [Citation26,Citation27]. LC3 has three isotypes including LC3A, LC3B, and LC3C, while only LC3B-II is correlated with increased levels of autophagic vesicles. After translation, the unprocessed form of LC3 is proteolytically cleaved by Atg4 protease and leads to formation of LC3-I with a carboxyterminal exposed glycine. When autophagy is activated, the exposed glycine of LC3-I is conjugated to the highly lipophilic phosphatidylethanolamine moiety to generate LC3-II, which is used as an autophagy marker [Citation28,Citation29]. Next, the membranes were incubated with secondary antibody horseradish peroxidase-labeled immunoglobulin G (ab6721; 1:1000; Abcam) at room temperature for 2 h. The protein bands were visualized by enhanced chemiluminescence reagent (Bio-Rad) and analyzed by the ImageJ software.
Statistical analysis
All collective data were analyzed using SPSS version 21.0 statistics software (IBM Corp., Armonk, NY). The measurement data were expressed as the mean ± standard deviation. The differences between two groups were compared using t test and comparisons among multiple groups were analyzed by one‐way analysis of variance. If these tests were not used, the rank sum test was used. P < 0.05 was considered statistically significant.
Results
NMDA induces autophagy and injury of RGCs
We first explored the autophagy and PI3K pathways in RGCs by NMDA treatment. NMDA with different concentrations (0, 50, 100, or 150 μmol/L) was used to treat RGCs. According to MTT assay results, the viability of RGCs was gradually decreased with NMDA concentration increasing (). Subsequently, the expression of autophagy-related proteins (LC3-I, LC3-II, Beclin-1, and ATG5) and PI3K/AKT signaling-associated proteins (pAKT, AKT, pPI3K, and PI3K) in RGCs were detected by Western blotting. As demonstrated, the protein expression of LC3-II, Beclin-1, and ATG5 in RGCs was increased with NMDA concentration increasing, suggesting that NMDA induces autophagy of RGCs. Furthermore, the protein expression of p-AKT and p-PI3K in RGCs was declined with the increased concentration of NMDA (), which indicated that NMDA inhibited the PI3K/AKT signaling in RGCs. All these results revealed that 150 μmol/L of NMDA has the best effects on inducing cell injury, autophagy, and inactivating PI3K/AKT signaling in RGCs. Thus, 150 μmol/L of NMDA was used in the following experiments.
Figure 1. NMDA induces autophagy and injury of RGCs. (a) The viability of RGCs treated with different concentrations (0, 50, 100 or 150 μmol/L) of NMDA was evaluated by MTT assay. (b) The expression of autophagy-related proteins (LC3-I, LC3-II, Beclin-1 and ATG5) in RGCs treated with different concentrations (0, 50, 100 or 150 μmol/L) of NMDA was examined by Western blotting. (c) The expression of PI3K/AKT signaling-associated proteins (pAKT, AKT, pPI3K and PI3K) in RGCs treated with different concentrations (0, 50, 100 or 150 μmol/L) of NMDA was tested by Western blotting. *p < 0.05, ** p < 0.01, ***p < 0.001
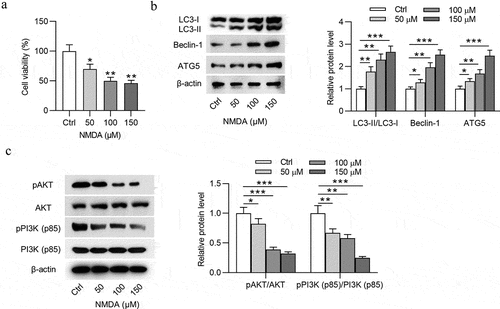
Baicalin inhibits NMDA-induced autophagy, cell injury and oxidative stress of RGCs via activating PI3K/AKT signaling
Impacts of NMDA on the apoptosis, autophagy, and oxidative stress of RGCs were subsequently detected. To mimic glaucoma environment in vitro, NMDA at the concentration of 150 μmol/L was used to treat RGCs. After NMDA stimulation, the viability of RGCs was reduced () and apoptosis rate of RGCs was increased ()). In addition, NMDA treatment increased the protein expression of LC3-II, Beclin-1, and ATG5 () and decreased the protein expression of p-AKT and p-PI3K () in RGCs. As ) showed, NMDA elevated ROS and MDA levels in RGCs ()), indicating that NMDA induced oxidative stress of RGCs. All these findings meant that a cellular model of glaucoma was established successfully. Next, to figure out the role of baicalin in glaucoma in vitro, baicalin was used to treat RGCs after NMDA stimulation. MTT assay revealed that baicalin offset the NMDA-induced decrease in viability of RGCs (). Flow cytometry analysis demonstrated that baicalin attenuated the promotive effects of NMDA on apoptosis of RGCs (). The elevation in LC3-II, Beclin-1, and ATG5 protein levels and decrease in p-AKT and p-PI3K protein levels were caused by NMDA in RGCs and were counteracted by baicalin (). The chemical structure of baicalin (5,6-dihydroxy7-O-glucuronide) is shown in . DCFH-DA staining assay suggested that the increase of ROS and MDA levels in NMDA-treated RGCs was neutralized by baicalin (). Next, to explore whether baicalin can regulate the PI3K/AKT signaling in NMDA-stimulated RGCs, LY294002 was applied to treat RGCs. However, LY294002 treatment was found to reverse all effects of baicalin on cell viability (), cell apoptosis (), autophagy-related protein expression (), PI3K/AKT signaling-associated protein expression (), and ROS and MDA levels () in NMDA-treated RGCs. To sum up, baicalin inhibits autophagy, cell injury and oxidative stress of NMDA-treated RGCs via activating PI3K/AKT signaling.
Figure 2. Baicalin inhibits autophagy and injury of NMDA-treated RGCs via activating PI3K/AKT signaling. (a) The viability of RGCs in four groups (Ctrl, NMDA, NMDA+BAI and NMDA+BAI+LY294002) was assessed by MTT assay. (b-c) Apoptosis of RGCs in four groups (Ctrl, NMDA, NMDA+BAI and NMDA+BAI+LY294002) was tested by flow cytometry. (d-e) The expression of autophagy-related proteins (LC3-I, LC3-II, Beclin-1 and ATG5) and PI3K/AKT signaling-associated proteins (pAKT, AKT, pPI3K and PI3K) in RGCs in four groups (Ctrl, NMDA, NMDA+BAI and NMDA+BAI+LY294002) was evaluated by Western blotting. (f) Chemical structure of baicalin was presented. ** p < 0.01, *** p < 0.001
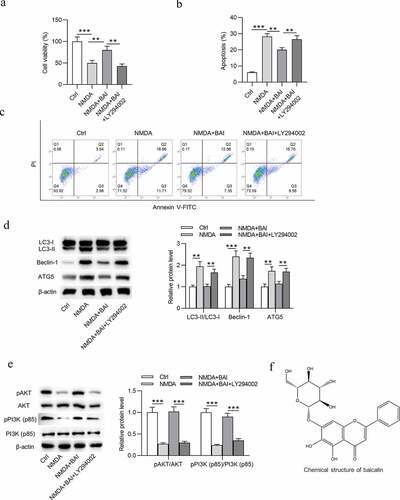
Successful establishment of a mouse model of glaucoma
IOP variation of both eyes of mice in the control group, glaucoma group, and glaucoma+baicalin group was measured. As demonstrated, there was no obvious difference between IOP of right eye and IOP of left eye in the control group (p > 0.05). However, a significant upregulation was detected in IOP of right eye compared with IOP of left eye in the experimental group (p < 0.01). Simultaneously, IOP of right eye in the experimental group was gradually increased with time increasing, while no obvious IOP variation was detected in left eye and right eye of the control group during experimental time. Moreover, baicalin treatment induced the lower IOP of the right eyes in glaucoma mice (). All these data suggested that a mouse model of glaucoma was established successfully, and baicalin can alleviate glaucoma.
Figure 4. Successful establishment of a mouse model of glaucoma. (a) IOP variation in left eye and right eye of mice in the control group (n = 10) was measured at 0, 3, 7, 17, 21, 28 and 42 days. (b) IOP variation in left eye and right eye of mice with glaucoma after baicalin treatment was measured at 0, 3, 7, 17, 21, 28 and 42 days. ** p < 0.01
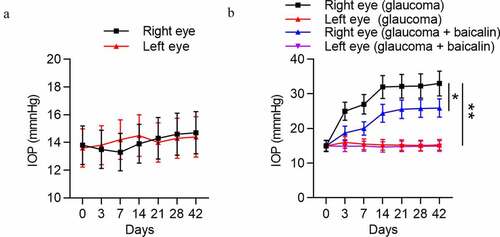
Baicalin increases RGC number and attenuates pathological changes of glaucoma mice
After mice were successfully induced with glaucoma, the pathological changes of retinal tissues in different groups (control, model, Model+NS and Model+BAI) were detected by H&E staining. As indicated, control group exhibited clear retinal layer and compact and neatly arranged RGCs with higher density. However, the structure and morphology of retinal tissues in glaucoma mice changed with indistinct layer of retinas, decrease in thickness of GCL and RGC density (RGCD) (). In addition, the number of RGCs in retinal tissues was decreased in model group compared with control group (). All these findings further confirmed successful establishment of a mouse model of glaucoma. To explore the effects of baicalin in glaucoma mice, baicalin were intraperitoneally injected into the glaucoma mice. Compared with Model+NS group, baicalin administration mitigated pathological changes of retinal tissues in glaucoma mice, evidenced by increase in thickness of GCL and RGCD in Model+BAI group (). Furthermore, reduction in RGC number in Model+NS group was attenuated by baicalin treatment in glaucoma mice (). All in all, baicalin attenuates glaucoma pathological changes and increases the number of RGCs in EIOP-stimulated mice.
Figure 5. Baicalin increases RGC number and attenuates pathological changes in retinas of glaucoma mice. (a) The structure and morphology of retina of mice in four groups (control, model, Model+NS and Model+BAI) were presented by H&E staining (scale bar = 25 μm). (b) Thickness of GCL and (c) RGCD of mice in four groups (control, model, Model+NS and Model+BAI) were measured. (d) The number of RGCs in retina tissues of four groups (control, model, Model+NS and Model+BAI) was counted. (e) Counting of RGCs using immunoflurescence staining of brain specific homeobox/POU domain protein 3B (Brn3b) in retina tissues of four groups (control, model, Model+NS and Model+BAI). *p < 0.05, ** p < 0.01, *** p < 0.001
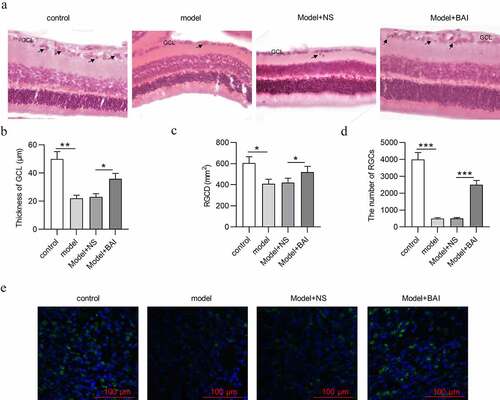
Baicalin inhibits autophagy and activates PI3K/AKT signaling in glaucoma mice
To investigate the regulatory role of baicalin in autophagy and PI3K/AKT signaling in glaucoma mice, the expression of autophagy-related proteins (LC3-I, LC3-II, Beclin-1, and ATG5) and PI3K/AKT signaling-associated proteins (pAKT, AKT, pPI3K and PI3K) in retinal tissues of four groups (control, model, Model+NS and Model+BAI) was detected by Western blotting. As showed, increased protein expression of LC3-II, Beclin-1, and ATG5 and decreased protein expression of p-AKT and p-PI3K was observed in model group compared with control group, suggesting that autophagy was promoted and PI3K/AKT signaling was inactivated in glaucoma mice. However, baicalin treatment attenuated the EIOP-induced increase in protein expression of LC3-II, Beclin-1, and ATG5 in Model+NS group, which indicated that baicalin inhibited autophagy in glaucoma progression (). Additionally, baicalin administration reversed the EIOP-induced decrease in p-AKT and p-PI3K protein levels in Model+NS group, meaning that baicalin activated PI3K/AKT signaling in glaucoma (). To sum up, baicalin inhibits autophagy and activates PI3K/AKT signaling in glaucoma pathogenesis.
Figure 6. Baicalin inhibits autophagy and activates PI3K/AKT signaling in glaucoma mice. (a) The expression of autophagy-related proteins (LC3-I, LC3-II, Beclin-1 and ATG5) and (b) PI3K/AKT signaling-associated proteins (pAKT, AKT, pPI3K and PI3K) in retinal tissues of four groups (control, model, Model+NS and Model+BAI) was detected by Western blotting. **p < 0.01, *** p < 0.001
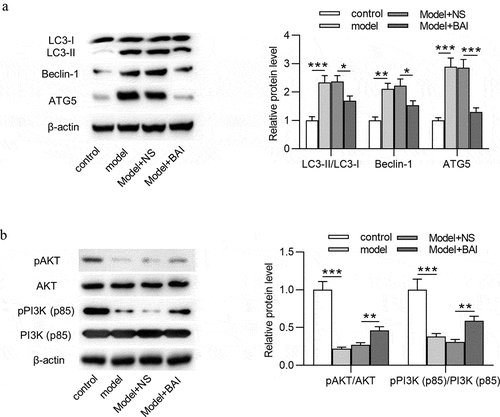
Discussion
NMDA is an important excitatory neurotransmitter L-glutamate homologue in the mammalian central nervous system [Citation30]. Accumulating studies have revealed that NMDA can induce RGC degradation and retinal injury [Citation31,Citation32]. In the present study, NMDA with different concentrations (0, 50, 100, or 150 μmol/L) was used to treat RGCs. Viability of RGCs was gradually decreased with NMDA concentration increasing. As a self-digestion mechanism of cells, autophagy can degrade damaged organelles and proteins in response to cell metabolic stress and also can cause cell death [Citation33]. Elevation in autophagy-related proteins was detected in NMDA-treated RGCs or rats, and RGC autophagy contributed to retinal neuron loss in the ganglion cell layer [Citation34]. Herein, the protein expression of autophagy-related proteins (LC3-II, Beclin-1 and ATG5) in RGCs was concentration-dependently increased by NMDA, suggesting that NMDA induces autophagy of RGCs.
PI3K (phosphatidylinositol kinase) is a dimer composed of the regulatory subunit p85 and the catalytic subunit p110 [Citation35]. Multiple evidence has identified that the PI3K/AKT signaling restrains apoptosis and inflammatory response of RGCs in glaucoma development [Citation36,Citation37]. Moreover, increased ROS during oxidative stress can induce autophagy [Citation38]. The PI3K/AKT signaling negatively regulates the oxidative stress response [Citation39] and the autophagy pathway [Citation40]. In this study, the protein expression of p-AKT and p-PI3K in RGCs was decreased by NMDA, meaning that NMDA inhibits the PI3K/AKT signaling in RGCs.
Baicalin was revealed to play a neuroprotective role in glaucoma progression [Citation23,Citation41]. Our findings revealed that baicalin exerted pro-proliferative and anti-apoptotic effects on NMDA-treated RGCs, suggesting that baicalin mitigates NMDA-induced cell injury of RGCs. Baicalin has been reported to regulate autophagy via PI3K/AKT signaling pathway [Citation42,Citation43]. In this study, NMDA-induced the elevation in levels of LC3-II, Beclin-1, and ATG5 in RGCs was counteracted by baicalin, which indicated that baicalin inhibits autophagy of NMDA-treated RGCs. In addition, baicalin reversed the NMDA-induced inhibition in p-AKT and p-PI3K protein levels in RGCs, demonstrating that baicalin activates PI3K/AKT signaling in NMDA-stimulated RGCs. As a key step in glaucoma pathophysiology, oxidative stress is connected to RGC apoptosis and outflow facility dysregulation [Citation44,Citation45]. Our findings showed that the NMDA-induced increase of ROS and MDA levels in RGCs was neutralized by baicalin, indicating that baicalin inhibits oxidative stress in glaucoma in vitro. Next, LY294002 treatment counteracted the effects of baicalin on cell viability, apoptosis, autophagy, ROS, and MDA levels in NMDA-treated RGCs. It can be concluded that baicalin restrains cell injury, autophagy, and oxidative stress via activating PI3K/AKT signaling in an in vitro model of glaucoma. The in vivo data revealed that baicalin offset the decrease in GCL thickness and RGCD in retinas of glaucoma mice. Moreover, the number of RGCs was significantly decreased in glaucoma mice, and then increased by baicalin treatment. Similarly, baicalin also inhibited autophagy and activated PI3K/AKT signaling in vivo. Therefore, baicalin suppresses glaucoma pathogenesis by regulating PI3K/AKT signaling in vitro and in vivo.
Since other Chinese herb medicines, such as lycium barbarum [Citation46], cordyceps cicadae mycelia [Citation47] and resveratrol [Citation48] were reported to alleviate glaucoma, more studies about whether these Chinese herb medicines can regulate PI3K/AKT signaling in glaucoma development should be conducted. In addition to PI3K/AKT signaling, other signal pathways including mitogen-activated protein kinase 1 signaling [Citation49] and nuclear factor kappa B signaling [Citation50], were demonstrated to be modulated by baicalin and were not mentioned in the present study, which is a limitation of our study. Studies on whether baicalin can regulate these signaling pathways in glaucoma pathogenesis also should be performed in the future. Moreover, the methods to induce the in vivo and in vitro models of glaucoma were not consistent, and we will use the NMDA-induced glaucoma mice to explore the influences of baicalin on autophagy and PI3K pathways in glaucoma if necessary.
Conclusion
Baicalin restrained the NMDA-induced cell apoptosis, autophagy, and oxidative stress of RGCs by activating the PI3K/AKT signaling in vitro. In vivo studies showed that baicalin activated PI3K/AKT signaling to suppress autophagy, subsequently attenuating the pathological changes in retinal tissues of glaucoma mice. These findings indicated baicalin as a promising drug for glaucoma treatment.
Disclosure statement
The authors report no declarations of interest.
Data availability statement
The datasets used during the current study are available from the corresponding author on reasonable request.
Additional information
Funding
References
- He S, Stankowska DL, Ellis DZ, et al. Targets of neuroprotection in glaucoma. J Ocul Pharmacol Ther. 2018 Jan/Feb;34(1–2):85–106.
- Aref AA, Gedde SJ, Budenz DL. Glaucoma drainage implant surgery. Dev Ophthalmol. 2017;59:43–52.
- Flaxman SR, Bourne RRA, Resnikoff S, et al. Global causes of blindness and distance vision impairment 1990-2020: a systematic review and meta-analysis. Lancet Glob Health. 2017 Dec;5(12):e1221–e1234.
- Bol P. [glaucoma]. Ned Tijdschr Tandheelkd. 2003 Jul;110(7):298–299.
- Thau A, Lloyd M, Freedman S, et al. New classification system for pediatric glaucoma: implications for clinical care and a research registry. Curr Opin Ophthalmol. 2018 Sep;29(5):385–394.
- Toris CB, Zhan G, Fan S, et al. Effects of travoprost on aqueous humor dynamics in patients with elevated intraocular pressure. J Glaucoma. 2007 Mar;16(2):189–195.
- Beier C, Zhang Z, Yurgel M, et al. Projections of iprgcs and conventional rgcs to retinorecipient brain nuclei. J Comp Neurol. 2021 Jun;529(8):1863–1875.
- Wang L, Gong J, Wang J, et al. Long non-coding RNA malat1 alleviates the elevated intraocular pressure (eiop)-induced glaucoma progression via sponging mir-149-5p. Curr Eye Res. 2021 Jun;46(6):903–911.
- Wang Y, Huang C, Zhang H, et al. Autophagy in glaucoma: crosstalk with apoptosis and its implications. Brain Res Bull. 2015;117:1–9.
- Kimura A, Namekata K, Guo X, et al. Targeting oxidative stress for treatment of glaucoma and optic neuritis. Oxid Med Cell Longev. 2017;2017:2817252.
- Bluwol E. [glaucoma treatment]. Rev Prat. 2016 May;66(5):508–513.
- Fekih O, Zgolli HM, Mabrouk S, et al. Malignant glaucoma management: literature review. Tunis Med. 2019 Aug-Sep;97(8–9):945–949.
- Yu H, Chen B, Ren Q. Baicalin relieves hypoxia-aroused h9c2 cell apoptosis by activating nrf2/ho-1-mediated hif1α/bnip3 pathway. Artif Cells Nanomed Biotechnol. 2019 Dec;47(1):3657–3663.
- Huang T, Liu Y, Zhang C. Pharmacokinetics and bioavailability enhancement of baicalin: a review. Eur J Drug Metab Pharmacokinet. 2019 Apr;44(2):159–168.
- Guo LT, Wang SQ, Su J, et al. Baicalin ameliorates neuroinflammation-induced depressive-like behavior through inhibition of toll-like receptor 4 expression via the pi3k/akt/foxo1 pathway. J Neuroinflammation. 2019 May 8;16(1):95.
- Zhou HC, Du R, Wang H, et al. [advance in studies on pharmacokinetics of baicalin]. Zhongguo Zhong Yao Za Zhi. 2018Feb; 432: 684–688.
- Xiao JR, Do CW, To CH. Potential therapeutic effects of baicalein, baicalin, and wogonin in ocular disorders. J Ocul Pharmacol Ther. 2014 Oct;30(8):605–614.
- Chao HM, Chuang MJ, Liu JH, et al. Baicalein protects against retinal ischemia by antioxidation, antiapoptosis, downregulation of hif-1α, vegf, and mmp-9 and upregulation of ho-1. J Ocul Pharmacol Ther. 2013 Jul-Aug;29(6):539–549.
- Chandrasekher G, Ma X, Lallier TE, et al. Delay of corneal epithelial wound healing and induction of keratocyte apoptosis by platelet-activating factor. Invest Ophthalmol Vis Sci. 2002 May;43(5):1422–1428.
- Li SZ, Mao WS, Du XY, et al. [inhibition of rat lens aldose reductase by flavonoids-matteucinol and baicalein]. Yan Ke Xue Bao. 1987Jun; 32: 93–4, 137.
- Maher P, Hanneken A. Flavonoids protect retinal ganglion cells from oxidative stress-induced death. Invest Ophthalmol Vis Sci. 2005 Dec;46(12):4796–4803.
- Tian Y, He Y, Song W, et al. Neuroprotective effect of deferoxamine on n-methyl-d-aspartate-induced excitotoxicity in RGC-5 cells. Acta Biochim Biophys Sin (Shanghai). 2017 Sep 1;49(9):827–834.
- Zhang YY, Li ZD, Jiang N, et al. [The effects and mechanism of baicalin in a mouse acute hypertensive glaucoma model]. Zhonghua Yan Ke Za Zhi. 2020 May 11;56(5):376–382.
- Chen Q, Xi X, Zeng Y, et al. Acteoside inhibits autophagic apoptosis of retinal ganglion cells to rescue glaucoma-induced optic atrophy. J Cell Biochem. 2019 Aug;120(8):13133–13140.
- Nie XG, Fan DS, Huang YX, et al. Downregulation of microRNA-149 in retinal ganglion cells suppresses apoptosis through activation of the pi3k/akt signaling pathway in mice with glaucoma. Am J Physiol Cell Physiol. 2018 Dec 1;315(6):C839–c849.
- He H, Dang Y, Dai F, et al. Post-translational modifications of three members of the human map1lc3 family and detection of a novel type of modification for map1lc3b. J Biol Chem. 2003 Aug 1;278(31):29278–29287.
- Kabeya Y, Mizushima N, Ueno T, et al. Lc3, a mammalian homologue of yeast apg8p, is localized in autophagosome membranes after processing. EMBO J. 2000 Nov 1;19(21):5720–5728.
- Mizushima N. Autophagy: process and function. Genes Dev. 2007 Nov 15;21(22):2861–2873.
- Nakatogawa H, Suzuki K, Kamada Y, et al. Dynamics and diversity in autophagy mechanisms: lessons from yeast. Nat Rev Mol Cell Biol. 2009 Jul;10(7):458–467.
- Shibata K, Imanishi D, Abe K, et al. D-aspartate n-methyltransferase catalyzes biosynthesis of n-methyl-d-aspartate (NMDA), a well-known selective agonist of the NMDA receptor, in mice. Biochim Biophys Acta Proteins Proteom. 2020 Dec;1868(12):140527.
- Fazel MF, Abu IF, Mohamad MHN, et al. Philanthotoxin-343 attenuates retinal and optic nerve injury, and protects visual function in rats with n-methyl-d-aspartate-induced excitotoxicity. PLoS One. 2020;15(7):e0236450.
- Wang S, Gu D, Zhang P, et al. Melanopsin-expressing retinal ganglion cells are relatively resistant to excitotoxicity induced by n-methyl-d-aspartate. Neurosci Lett. 2018 Jan;1(662):368–373.
- Glick D, Barth S, Macleod KF. Autophagy: cellular and molecular mechanisms. J Pathol. 2010 May;221(1):3–12.
- Li R, Jin Y, Li Q, et al. Mir-93-5p targeting PTEN regulates the NMDA-induced autophagy of retinal ganglion cells via akt/mtor pathway in glaucoma. Biomed Pharmacother. 2018;100:1–7.
- Xie Y, Shi X, Sheng K, et al. Pi3k/akt signaling transduction pathway, erythropoiesis and glycolysis in hypoxia (review). Mol Med Rep. 2019 Feb;19(2):783–791.
- Li RX, Wang Q, Shen ZW, et al. Mtim affects retinal ganglion cells through pi3k/akt signaling pathway. Eur Rev Med Pharmacol Sci. 2020 May;24(10):5209–5216.
- Xu K, Li S, Yang Q, et al. microRNA-145-5p targeting of trim 2 mediates the apoptosis of retinal ganglion cells via the pi3k/akt signaling pathway in glaucoma. J Gene Med.2021Jul;22: e3378.
- Filomeni G, De Zio D, Cecconi F. Oxidative stress and autophagy: the clash between damage and metabolic needs. Cell Death Diff. 2015 Mar;22(3):377–388.
- Huang W, Cao Z, Zhang J, et al. Aflatoxin b(1) promotes autophagy associated with oxidative stress-related pi3k/akt/mtor signaling pathway in mice testis. Environ Pollut. 2019 Dec;255(Pt 2):113317.
- Heras-Sandoval D, Pérez-Rojas JM, Hernández-Damián J, et al. The role of pi3k/akt/mtor pathway in the modulation of autophagy and the clearance of protein aggregates in neurodegeneration. Cell Signal. 2014 Dec;26(12):2694–2701.
- Gong L, Zhu J. Baicalin alleviates oxidative stress damage in trabecular meshwork cells in vitro. Naunyn-Schmiedeberg’s Arch Pharmacol. 2018 Jan;391(1):51–58.
- Zhang Q, Sun J, Wang Y, et al. Antimycobacterial and anti-inflammatory mechanisms of baicalin via induced autophagy in macrophages infected with mycobacterium tuberculosis. Front Microbiol. 2017;8:2142.
- Kang C, Wang L, Kang M, et al. Baicalin alleviates 6-hydroxydopamine-induced neurotoxicity in pc12 cells by down-regulation of microRNA-192-5p. Brain Res. 2019 Apr 1;1708:84–92.
- Kumar DM, Agarwal N. Oxidative stress in glaucoma: a burden of evidence. J Glaucoma. 2007 May;16(3):334–343.
- Li G, Luna C, Liton PB, et al. Sustained stress response after oxidative stress in trabecular meshwork cells. Mol Vis. 2007 Dec;17(13):2282–2288.
- Manthey AL, Chiu K, So KF. Effects of lycium barbarum on the visual system. Int Rev Neurobiol. 2017;135:1–27.
- Horng CT, Yang YL, Chen CC, et al. Intraocular pressure-lowering effect of cordyceps cicadae mycelia extract in a glaucoma rat model. Int J Med Sci. 2021;18(4):1007–1014.
- Pirhan D, Yüksel N, Emre E, et al. Riluzole- and resveratrol-induced delay of retinal ganglion cell death in an experimental model of glaucoma. Curr Eye Res. 2016;41(1):59–69.
- Wang Z, Ma L, Su M, et al. Baicalin induces cellular senescence in human colon cancer cells via upregulation of depp and the activation of ras/raf/mek/erk signaling. Cell Death Dis. 2018 Feb 13;9(2):217.
- Jin X, Liu MY, Zhang DF, et al. Baicalin mitigates cognitive impairment and protects neurons from microglia-mediated neuroinflammation via suppressing nlrp3 inflammasomes and tlr4/nf-κb signaling pathway. CNS Neurosci Ther. 2019 May;25(5):575–590.