ABSTRACT
It has been reported that circHIPK3 can be downregulated by high glucose, suggesting its potential involvement in diabetes and diabetic complications. This study aimed to explore the role of circHIPK3 in diabetic cardiomyopathy (DC). PTEN is a kind of tumor suppressor gene, which is very commonly lost in human cancer. We detected the expression of circHIPK3 and PTEN in plasma samples from DC patients, diabetic patients without complications diabetes mellitus (DM) and health controls by RT-qPCR and ELISA. In vitro cell experiment, AC16 cells (cardiomyocytes) were treated with high glucose, followed by expression analysis of circHIPK3 and PTEN mRNA by RT-qPCR. CircHIPK3 or PTEN expression vector were used to overexpress circHIPK3 and PTEN in AC16 cells to explore the relationship between them. The role of circHIPK3 and PTEN in regulating the apoptosis of AC16 cells was analyzed by cell apoptosis assay. The result showed that CircHIPK3 was downregulated in diabetes and further downregulated in DC. In AC16 cells, high glucose treatment decreased the expression levels of circHIPK3. Across DC samples, the expression of circHIPK3 was inversely correlated with PTEN. In AC16 cells, overexpression of circHIPK3 decreased the expression levels of PTEN. CircHIPK3 may suppress AC16 cell apoptosis induced by high glucose and inhibited the role of PTEN in cell apoptosis. Therefore, circHIPK3 may downregulate PTEN to protect cardiomyocytes from high glucose-induced cell apoptosis.
Graphical abstracts
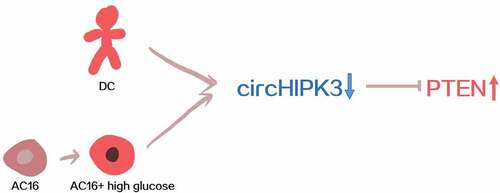
Introduction
Diabetes, also known as DM, is the most common type of metabolic disorder worldwide [Citation1–3]. It is estimated that more than 10.5% of the population in the US are suffering from DM [Citation4]. Even worse, with the growth of aging population and changes in people’s dietary structure, the incidence of DM is expected to be increasing in the near future [Citation5,Citation6]. DM itself is not a lethal condition, however, long-term of DM will inevitably cause damages to most of the important organs of the human body, including the heart [Citation7,Citation8]. DC refers to a disorder in the heart muscle of DM patients [Citation9]. DC significantly affects the circulation, eventually leading to heart failure that is related to high mortality [Citation10].
PTEN (Phosphatase and tensin homologue deleted on chromosome 10, also called MMAC1, mutated in multiple advanced cancers 1) is a tumor suppressor as it can regulate cell proliferation, cell growth, and prevent tumor formation. In addition, studies have shown that PTEN can regulate the PI3K signaling pathway, which is a key factor in the negative regulation of insulin [Citation11]. Studies have also shown that PTEN is not only the target gene of miR-10b-5p, but the downstream gene of miR-10b-5p as well, which regulates the apoptosis of cardiomyocytes induced by hypoxia. Hypoxia inducible factor-1 α (HIF-1α) regulates the expression of tumor suppressive gene PTEN, thus reducing the apoptosis of cardiomyocytes after hypoxia [Citation12,Citation13].
With protein coding capacity, circular RNAs (circRNAs) participate in human diseases mainly by indirectly affecting protein accumulation [Citation14–18]. A recent study showed that circHIPK3 (also known as circRNA homeodomain-interacting protein kinase 3) has protective effects on high glucose-induced cell injury [Citation19], suggesting the involvement of circHIPK3 in diabetic complications. Meanwhile, preliminary microarray analysis data showed an inverse correlation between circHIPK3 and PTEN, which may promote DC by inducing cell apoptosis [Citation20]. In order to explore the interaction between PTEN and circHIPK3 in DC, the content of circHIPK3 and PTEN in patients’ serum and high glucose culture of cardiomyocytes in vitro were detected in this study, and through overexpression plasmid transfection experiment to find the regulatory relationship between circHIPK3 and PTEN.
Materials and methods
Patients and healthy participants
This study enrolled DC patients (n = 60), diabetic patients without complications (DM group, n = 60), and health controls (the Control group, n = 60) who were admitted at Baoquanling Central Hospital between May 2018 and May 2020. This study was approved by the Ethics Committee of this hospital. Healthy controls exhibited normal functions in systemic physiological exam and participants with previous histories of diabetes were excluded. The following criterion were used to diagnose diabetes: 1) glucose level (2-h plasma) higher than 11.1 mmol/L. 2) plasma glucose under fasting condition was higher than 7.0 mmol/L. DM and DC patients with other severe clinical disorders were excluded. Exclusion criteria: 1) patients who were complicated with severe mental illness and were unable to cooperate. 2) patients with severe hematological diseases. 3) patients with congenital heart disease and other severe organic diseases. 4) patients who were allergic to drugs in this study. 5) patients who initiated therapy within 3 months prior to enrollment. All the three groups included 40 males and 20 females, with the mean age of 53.4 ± 6.6 years old. All participants signed the written informed consent ().
Table 1. Clinical Characteristics of patients included
Plasma and AC16 cell preparation
Prior to therapy, fasting blood (2 ml) was extracted from each participant. To isolate plasma, blood samples were mixed with citric acids to a ratio of 10:1, followed by centrifugation at room temperature for 15 min.
AC16 cells (cardiomyocytes, Sigma-Aldrich, Shanghai, China, serial number TCH-G119) were used in this study. In cardiomyocyte growth medium (90% D/F12 + 12.5% FBS + 2 mM L-Glutamine + 1%P/S, ScienCell Research, Shanghai, China) AC16 cells were cultivated. To evaluate the effects of high glucose on gene expression, AC16 cells were treated with D-glucose (Sigma-Aldrich, Shanghai, China) at dosages of 10, 20, 30 and 40 mM for 48 h prior to the subsequent assays [Citation21].
Cell transfections
The expression vector of circHIPK3 or PTEN was established with pcDNA3.1 vector (Invitrogen, California, USA) as the backbone. To overexpress circHIPK3 and PTEN, AC16 cells were transfected with either 1 μg of expression vector or the same amount of empty vector (negative control group, NC) using lipofectamine 2000 (Invitrogen, California, USA). Untransfected cells were included as the control (C) cells.
RNA isolation
RNAzol (Sigma-Aldrich, Shanghai, China) was applied for total RNA isolation from both plasma samples and AC16 cells, followed by gDNA removal using DNase I (Invitrogen, California, USA) at 37°C for 100 min. RNA samples were separated by 6% urea-PAGE gel to check integrity. The OD 260/280 ratio was checked for all RNA samples to detect RNA purity.
Quantitative reverse transcription polymerase chain reaction (RT-qPCR) assay
The synthesis of cDNA samples was done with SS-III-RT (Invitrogen, California, USA) from RNA samples with satisfactory quality (an OD260/280 ratio close to 2.0 and satisfactory integrity) as the templates. To determine the expression of circHIPK3 and PTEN mRNA, qPCRs were carried out using SYBR Green Master Mix (Bio-Rad) with GAPDH as an internal control. The Ct values of circHIPK3 or PTEN were normalized to GAPDH using the 2-ΔΔCt method. The primer sequences were: circHIPK3, 5’-TATGTTGGTGGATCCTGTTCGGCA-3’ (forward) 5’-TGGTGGGTAGACCAAGA
CTTGTGA-3’ (reverse); PTEN, 5’-CGAACTGGTGTAATGATATGT-3’ (forward) 5’-CATGAACTTGTCTTCCCGT-3’ (reverse).
Enzyme linked immunosorbent assay (ELISA)
The expression levels of PTEN in plasma samples were determined using a human PTEN ELISA Kit (ab206979, Abcam, Hangzhou, China). The sensitivity was 39.9 pg/ml and the assay range was 125 pg/ml – 8000 pg/ml.
Western-blot analysis
RIPA solution (Invitrogen, California, USA) and BCA assay were used for protein extraction and quantification, respectively. Protein samples were first denatured in boiling water for 5 min, followed by separation using 7% SDS-PAGE gels. After gel transfer and blocking, incubation with PTEN (ab31392, Abcam, Hangzhou, China) or GAPDH (ab8245, Abcam, Hangzhou, China) primary antibodies was done at 4°C for overnight. Then, HRP IgG secondary antibody (ab6721, Abcam, Hangzhou, China) was used to incubate the membranes at room temperature for 3 h. ECL (ab65623, Abcam, Hangzhou, China) was used for the production of signals. Quantity One software was used for signal normalizations.
Cell apoptosis assay
In brief, AC16 cells were cultivated in medium supplemented with 60 mM D-glucose for further 48 h, followed by washing using pre-cold PBS. After that, cells were resuspended in binding buffer, and Annexin V FITC and PI (BioLegend, Shanghai, China) staining was performed for 15 min in dark. Finally, flow cytometry was performed to analyze apoptotic cells.
Statistical analyses
ANOVA Tukey’s test was applied for comparisons. Correlations were analyzed by Pearson’s correlation coefficient. P < 0.05 was considered as statistically significant.
Results
Altered expression of circHIPK3 and PTEN were observed in DM and DC groups
RT-qPCR and ELISA results showed that DM and DC groups exhibited significantly decreased plasma expression levels of circHIPK3 compared to that in the Control group. In addition, plasma expression levels of circHIPK3 were also lower in DC group compared to that in DM group (, n = 3, p < 0.05). In contrast, highest plasma expression levels of PTEN were observed in DC group, followed by DM group, and then the control group (, n = 3, p < 0.05).
Figure 1. Altered expression of circHIPK3 and PTEN were observed in DM and DC groups.
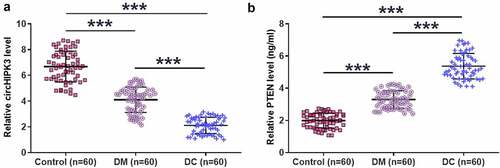
CircHIPK3 was inversely correlated with PTEN only in DC group
There was no difference between the expression of circHIPK3 and PTEN in the Control () and DM () groups. In contrast, circHIPK3 and PTEN were significantly and inversely correlated across DC group (). Therefore, circHIPK3 and PTEN may interact with each other in DC.
High glucose treatment altered the expression of circHIPK3 and PTEN in AC16 cells
To explore the effects of high glucose on gene expression, AC16 cells were treated with D-glucose at dosages of 10, 20, 30, and 40 mM for 48. High glucose treatment decreased the expression levels of circHIPK3 (, n = 3, p < 0.05) and increased the expression levels of PTEN (, n = 3, p < 0.05) in a dose-dependent manner. In addition, the expression of circHIPK3 was not different between 10 mM and 20 mM glucose treatment, and the expression of PTEN was not differed between 30 mM and 30 mM glucose treatment ().
Figure 3. High glucose treatment altered the expression of circHIPK3 and PTEN in AC16 cells.
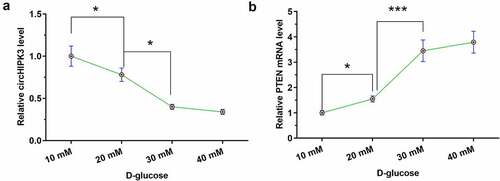
Overexpression of circHIPK3 decreased the expression levels of PTEN in AC16 cells
To analyze the interaction between circHIPK3 and PTEN, AC16 cells were transfected with circHIPK3 or PTEN expression vector, followed by RT-qPCR to determine the expression of circHIPK3 and PTEN. It was observed that circHIPK3 and PTEN were significantly upregulated. Meanwhile, transfected si-circHIPK3 vector into AC16 cells can significantly downregulate the expression of circHIPK3 (, n = 3, p < 0.05). Moreover, overexpression of circHIPK3 decreased the expression levels of PTEN. In contrast, overexpression of PTEN did not alter the expression of circHIPK3 at each time point (, n = 3, p > 0.05). We also detected lower PTEN protein expression levels in the circHIPK3 group than in the other groups, while the highest PTEN protein expression levels were found in the si-circHIPK3 group. (, n = 3, p > 0.05).
Figure 4. Overexpression of circHIPK3 decreased the expression levels of PTEN in AC16 cells.
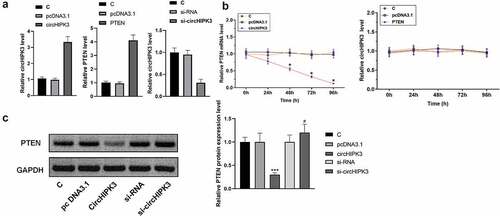
Overexpression of circHIPK3 suppressed the apoptosis of AC16 cells induced by high glucose through PTEN
AC16 cells with transfections were first incubated in medium supplemented with 30 mM D-glucose for 48 h, followed by cell apoptosis assay to analyze cell apoptosis. It was observed that overexpression of PTEN increased cell apoptosis. Moreover, overexpression of circHIPK3 suppressed the apoptosis of AC16 cells induced by high glucose and attenuated the effects of overexpression of PTEN on cell apoptosis (, n = 3, p < 0.05).
Discussion
This study mainly explored the involvement of circHIPK3 and its function in DC. We found that circHIPK3 was downregulated in DC and overexpression circHIPK3 may protect AC16 cells from high glucose-induced apoptosis by suppressing the expression of PTEN.
CircHIPK3 is a well-characterized oncogenic RNA in many types of cancer. For instance, circHIPK3 is upregulated in renal cancer and may inhibit the apoptosis of cancer cells by decreasing the expression levels of miR-485-3p [Citation22]. A recent study reported that circHIPK3 was downregulated in endothelial cells by high glucose treatment, which in turn induced cell injury [Citation19]. Consistently, our study also observed decreased expression levels of circHIPK3 in AC16 cells after treatment with high D-glucose treatment. Moreover, downregulation of circHIPK3 was observed in DM, and further downregulation was observed in DC. Therefore, the downregulation of circHIPK3 in DM is likely induced by high glucose treatment, and the further downregulation may result in the development of DC. Our study also showed inhibitory effects of overexpression of circHIPK3 on high-glucose induced apoptosis of AC16 cells. Therefore, overexpression of circHIPK3 may serve as a potential target for the treatment of DC. However, clinical trials are needed to verify our hypothesis.
Although the direct role of PTEN in DC has not been extensively explored, its potential role in heart injury has been studied in animal models [Citation21]. It was observed that PTEN was upregulated in rats with myocardial injury, and the targeting of PTEN by miR-26a reduces myocardial injury [Citation21]. In this study, we observed the upregulation of PTEN in DC. In addition, high glucose treatment also increased the expression levels of PTEN in AC16 cells. Therefore, PTEN is also high glucose-inducible and may promote DC development by increasing cell apoptosis. At present, there is no evidence to prove whether circHIPK3 affects the expression of miR-26a, which is one of the directions we are considering at present, and we will continue to explore it in future experiments and improve the research in this direction.
We showed that overexpression of circHIPK3 decreased the expression levels of both PTEN mRNA and protein. Therefore, circHIPK3 may affect the transcription of PTEN or affect PTEN mRNA stability. However, future studies are still needed to verify this.
In this study, circHIPK3 can negatively regulate the expression of PTEN, and it has been confirmed that PTEN is a downstream protein of circHIPK3. In clinical treatment, the therapeutic effect can be achieved by targeting the expression of PTEN. It is well known that PTEN can regulate the release of insulin [Citation11] and also plays a key role in the cardiovascular system [Citation23]. This may be good news for patients with DC. PTEN may be able to combine the treatment of diabetes and heart disease. The direct regulation of PTEN or the indirect regulation of PTEN by circHIPK3 may be a therapeutic approach for DC.
Conclusion
In conclusion, circHIPK3 is downregulated in DC. In addition, circHIPK3 may downregulate PTEN to suppress the apoptosis of AC16 cells induced by high glucose, thereby improving DC.
Ethical approval and consent to participate
Informed consent was obtained from all individual participants included in the study.
All procedures were approved by Baoquanling Central Hospital Ethics Committee. Procedures operated in this research were completed in keeping with the standards set out in the Announcement of Helsinki and laboratory guidelines of research in China.
Availability of supporting data
The analyzed data sets generated during the study are available from the corresponding author on reasonable request.
Authors’ contributions
Jun Jiang, Guannan Gao, Qiang Pan, Jing Liu, supervised the whole study, data analysis, manuscript preparation.
Yu Tian, Xiaoji Zhang had data collection and analysis, manuscript preparation.
Disclosure statement
No potential conflict of interest was reported by the author(s).
Additional information
Funding
References
- Zimmet P, Alberti KG, Magliano DJ, et al. Diabetes mellitus statistics on prevalence and mortality: facts and fallacies. Nat Rev Endocrinol. 2016 Oct;12(10):616–622.
- Mirzaei H, Hamblin MR. Regulation of glycolysis by non-coding RNAs in cancer: switching on the Warburg effect. Mol Ther Oncolytics. 2020 Dec 16;19:218–239
- Razavi ZS, Tajiknia V, Majidi S, et al. Gynecologic cancers and non-coding RNAs: epigenetic regulators with emerging roles. Crit Rev Oncol Hematol. 2021 Jan;157:103192.
- Liese AD, Lawson A, Song HR, et al. Evaluating geographic variation in type 1 and type 2 diabetes mellitus incidence in youth in four US regions. Health Place. 2010 May;16(3):547–556.
- Sinclair A, Saeedi P, Kaundal A, et al. Diabetes and global ageing among 65-99-year-old adults: findings from the international diabetes federation diabetes atlas, 9(th) edition. Diabetes Res Clin Pract. 2020 Apr;162:108078.
- Cheung LTF, Chan RSM, GTC K, et al. Diet quality is inversely associated with obesity in Chinese adults with type 2 diabetes. Nutr J. 2018 Jul 3;17(1):63.
- Forbes JM, Cooper ME. Mechanisms of diabetic complications. Physiol Rev. 2013 Jan;93(1):137–188.
- Sharafati-Chaleshtori R, Nickdasti A, Mortezapour E, et al. Artemisia species as a new candidate for diabetes therapy: a comprehensive review. Curr Mol Med. 2021 Jan 1;21(10):832–849.
- Boudina S, Abel ED, Boudina S, et al. Diabetic cardiomyopathy, causes and effects. Rev Endocr Metab Disord. 2010 Mar;11(1):31–39.
- Jacob M, Chappell D, Rehm M. The ‘third space’–fact or fiction? Best Pract Res Clin Anaesthesiol. 2009 Jun;23(2):145–157.
- Li YZ, Di Cristofano A, Woo M. Metabolic role of PTEN in insulin signaling and resistance. Cold Spring Harb Perspect Med. 2020 Aug 3;10(8):a036137.
- Wu L, Chen Y, Chen Y, et al. Effect of HIF-1α/miR-10b-5p/PTEN on hypoxia-induced cardiomyocyte apoptosis. J Am Heart Assoc. 2019 Sep 17;8(18):e011948.
- Lu S, Strand KA, Mutryn MF, et al. PTEN (Phosphatase and tensin homolog) protects against ang II (angiotensin II)-Induced pathological vascular fibrosis and remodeling-brief report. Arterioscler Thromb Vasc Biol. 2020 Feb;40(2):394–403.
- Chen Y, Li C, Tan C, et al. Circular RNAs: a new frontier in the study of human diseases. J Med Genet. 2016 Jun;53(6):359–365.
- Meng X, Li X, Zhang P, et al. Circular RNA: an emerging key player in RNA world. Brief Bioinf. 2017 Jul 1;18(4):547–557.
- Abbaszadeh-Goudarzi K, Radbakhsh S, Pourhanifeh MH, et al. Circular RNA and diabetes: epigenetic regulator with diagnostic role. Curr Mol Med. 2020;20(7):516–526.
- Borran S, Ahmadi G, Rezaei S, et al. Circular RNAs: new players in thyroid cancer. Pathol Res Pract. 2020 Oct;216(10):153217.
- Nahand JS, Jamshidi S, Hamblin MR, et al. Circular RNAs: new epigenetic signatures in viral infections. Front Microbiol. 2020;11:1853.
- Cao Y, Yuan G, Zhang Y, et al. High glucose-induced circHIPK3 downregulation mediates endothelial cell injury. Biochem Biophys Res Commun. 2018 Dec 9;507(1–4):362–368.
- Cai SS, Tao XW, Long Y, et al. Effect of miR-26a on diabetic rats with myocardial injury by targeting PTEN. Eur Rev Med Pharmacol Sci. 2020 Oct;24(20):10307.
- Yang F, Qin Y, Wang Y, et al. LncRNA KCNQ1OT1 mediates pyroptosis in diabetic cardiomyopathy. Cell Physiol Biochem. 2018;50(4):1230–1244.
- Lai J, Xin J, Fu C, et al. CircHIPK3 promotes proliferation and metastasis and inhibits apoptosis of renal cancer cells by inhibiting MiR-485-3p. Cancer Cell Int. 2020;20(1):248.
- Oudit GY, Sun H, Kerfant BG, et al. The role of phosphoinositide-3 kinase and PTEN in cardiovascular physiology and disease. J Mol Cell Cardiol. 2004 Aug;37(2):449–471.