ABSTRACT
Study has suggested that long non-coding RNA DOCK9 antisense RNA2 (LncRNA DOCK9-AS2) may play an important role in atherosclerosis, but the specific role is unclear. In this article, we aim to explore the role and mechanism of DOCK9-AS2 in the proliferation and migration of vascular smooth muscle cells (VSMCs) in atherosclerosis. VSMCs were treated with oxidized low densitylipoprotein (ox-LDL) for 24 h to establish the model of atherosclerosis in vitro. Gain- and loss-of function experiments were conducted. Cell Counting Kit-8 (CCK-8) assay and Ki67 staining were used to evaluate the ability cell proliferation. Transwell assay and immunofluorescence staining of N-Cadherin and E-cadherin were carried out to detect cell migration. RNA immunoprecipitation (RIP) experiment, pull down assay and mRNA stability analysis were used to assess the relationship of DOCK9-AS2, Wnt5a and LIN28B. Western blot analysis was used to measure the protein expression levels. The results showed that DOCK9-AS2 knockdown inhibited the proliferation and migration of ox-LDL-induced VSMCs. Further study on the interaction between DOCK9-AS2, Wnt5a and LIN28B revealed that LIN28B could both directly interact with DOCK9-AS2 and Wnt5a, and DOCK9-AS2 regulated Wnt5a by targeting LIN28B. In addition, Overexpression of Wnt5a partly abolished the inhibitory effects of LIN28B knockdown or DOCK9-AS2 knockdown on cell proliferation and migration induced by in ox-LDL-induced proliferation and migration. In conclusion, the results showed that DOCK9-AS2 promoted the proliferation and migration of vascular smooth muscle cells in atherosclerosis through regulating Wnt5a by targeting LIN28B.
Background
Atherosclerosis is the common pathological basis of cardiovascular diseases and is one of the most common causes of death in the elderly [Citation1]. Considering the complexity and severity of atherosclerosis, it is of great significance to explore the pathogenesis of atherosclerosis and seek new therapeutic targets and drugs. In the occurrence and development of atherosclerosis, vascular smooth muscle cells (VSMCs) play a leading role in the structural and function changes of the arterial wall, and their proliferation and migration will accelerate the process of atherosclerosis [Citation2,Citation3]. Therefore, improving the function of vascular smooth muscle will slow down the development of atherosclerosis to a large extent.
Long non-coding RNA (lncRNA) is a group of non-protein-coding RNAs greater than 200 nucleotides, and is involved in biological and pathological processes in vivo or in vitro [Citation4]. Recently, more and more lncRNAs that interfere with the process of atherosclerosis have been discovered and identified in atherosclerotic cells (such as VSMCs, endothelial cells (ECs) and monocytes/macrophages), indicating that lncRNA plays a more and more important role in the development of atherosclerosis [Citation5–8]. DOCK9 antisense RNA2 (DOCK9-AS2) is identified as an important lncRNA related to atherosclerosis based on public datasets, and may become a new treatment and prognostic target in atherosclerosis [Citation6]. In papillary thyroid carcinoma, lncRNA DOCK9-AS2 activated Wnt/β-catenin pathway to aggravate stemness, proliferation, migration, and invasion [Citation9]. However, whether DOCK9-AS2 plays a role in atherosclerosis through Wnt signal pathway is unclear.
Wnt5a, a secreted glycoprotein, belongs to a family of highly conserved proteins that regulate important processes such as cell fate specification, embryonic development, cell proliferation, migration, and differentiation in a variety of organisms [Citation10,Citation11]. In atherosclerosis, Wnt5a also plays an important role. In inflammatory diseases including atherosclerosis, the expression of WNT5 is up-regulated, and inhibition of Wnt5a can delay the process of atherosclerosis by anti-inflammatory effect [Citation12–14]. Inhibiting Wnt5a also decreases foam cell formation in atherosclerosis [Citation15]. In addition, Wnt5a reduces the accumulation of cholesterol in lipid-loaded cells by regulating the mRNA expression of Caveolin-1 and ATP-Binding Cassette Transporter 1 (ABCA1), which are involved in reverse cholesterol transport [Citation12,Citation16]. Therefore, targeting Wnt5a may have great clinical implications in atherosclerosis.
Therefore, in this study, we aims to investigate the specific role of DOCK9-AS2 in the proliferation and migration of vascular smooth muscle cells in in vitro model of atherosclerosis, and to explore whether it played a role by regulating Wnt5a.
Methods
Cell culture
Human aortic vascular smooth muscle cells (VSMCs) were obtained from the American Tissue Culture Collection (ATCC; cat. no. CRL-1999). The cells were cultured in an incubator containing 5% CO2 at 37°C and maintained in Dulbecco’s Modified Eagle Medium (DMEM; Hyclone, South Logan, UT, USA) containing 10% fetal bovine serum (FBS; Gibco, Grand Island, NY, USA) and 1% penicillin/streptomycin (Beyotime, Beijing, China).
Reverse transcription polymerase chain reaction (RT-PCR)
Total RNA was extracted from VSMCs using Trizol reagents (Vazyme Biotech Co.,Ltd). The Nanodrop was used to quantify the RNA. Then reverse transcription was conducted by using Reverse Transcription system (Takara Biotechnology Co., Ltd., Dalian, China) to synthesize complementary DNA (cDNA). Subsequently, the SYBR Green PCR kit (Vazyme Biotech Co.,Ltd) was applied for quantitative (q) PCR analysis. The expression level was analyzed using 2−ΔΔCt method. Glyceraldehyde-phosphate dehydrogenase (GAPDH) served as the internal control. The primers used in this study were listed in .
Table 1. Primers used for qRT-PCR
Cell transfection and treatment
Short hairpin RNA negative control (shNC), short hairpin RNA targeting DOCK9-AS2 (shDOCK9-AS2; knockdown of lncRNA DOCK9-AS2), shWnt5a (knockdown of Wnt5a), shLIN28B (knockdown of LIN28B), pcDNA3.1 (empty vector), pc-Wnt5a (the overexpression vector of Wnt5a) obtained from Shanghai GenePharma Inc. VSMCs were seed in 6-well plates. When reaching 60–70% confluence, VSMCs were transfected with 50 nmol/l shNC, shDOCK9-AS2, shWnt5a, shLIN28B, pcDNA3.1 or pc-Wnt5a using Lipofectamine 3000 reagents (Invitrogen; Thermo Fisher Scientific, Inc.) according to the manufacturer’s instructions. After the cells were cultured with Opti-MEM serum-free medium for 6–8 h, cells were washed and continued to be cultured in DMEM medium with 10% FBS. At 48 h post transient transfection, the transfection efficiency was detected by qRT-PCR or Western blot assay.
After VSMCs were transfected or untransfected, VSMCs were treated with 100 μg/ml ox-LDL for 24 h to establish the model of atherosclerosis in vitro.
Cell counting kit-8 (CCK-8) assay
CCK-8 kit (Vazyme Biotech Co.,Ltd) was employed to assess the cell viability. The cells were plated in 96-well plates for 24 h, 48 h and 72 h, respectively. At different time points, 10 μl CCK-8 solution was added into each well. After 1–4 h of incubation at 37°C, the absorbance at 450 nm was measured via a microplate reader (Promega Corporation).
Immunofluorescence
The cells were inoculated in 24-well culture plates with aseptic cover slices. After indicated treatments, cells were fixed with 4% paraformaldehyde for 0.5 h at room temperature and punched with 0.2% Triton X-100 for 30 min at room temperature. Then, the cells were sealed with 5% bovine serum albumin (BSA) for 1 h, and incubated with primary antibodies, including Ki67 (cat. no. 12,075; Cell Signaling Technology, Inc.), E-cadherin (cat. no. 14472S; Cell Signaling Technology, Inc.), and N-cadherin (cat. no. 14215S; Cell Signaling Technology, Inc.), overnight at 4 °C, followed by incubation with Alexa Fluor 594-conjugated secondary antibody (cat. no. A-11012; Invitrogen; Thermo Fisher Scientific, Inc.) for 2 h in the dark. Cell nuclei was stained with DAPI for 5 min in the dark at room temperature. Fluorescence microscope (Olympus Corporation) was used to obtain the pictures, and the fluorescence images were quantified using Image J software (National Institutes of Health).
Biotinylated RNA pull down assay
RNA pull-down assay was conducted to identify the interaction between LIN28B/LIN28/IGF2BP3 and DOCK9-AS2/Wnt5a with based on the previous method [Citation17]. In brief, Biotin-labeled DOCK9-AS2 and its antisense were transcribed in vitro with the biotin RNA Labeling mix (Roche, Switzerland) and T7 RNA polymerase (Promega Corporation, Madison, WI, USA). The marked RNA was purified and renatured with Annealing Buffer for RNA oligos (Beytotime, Shanghai, China) and heating at 90 °C for 2 min. Subsequently, the RNA was incubated on ice for 2 min and at room temperature for 30 min. M-280 streptavidin magnetic beads (Invitrogen; Thermo Fisher Scientific, Inc.) were used to pull down the biotinylated transcripts for 2 h at room temperature. Cell lysates were incubated with the M-280 streptavidin magnetic beads pre-coated with RNase-free BSA and yeast tRNA (Signa-Aldrich, MO, USA) at 4 °C for 30 min. Then, the beads-RNA-proteins were washed, and the proteins were precipitated and detected by Western blot analysis.
Nuclear and cytoplasmic isolation
Nucleocytoplasmic separation was carried out using Nuclei EZ Lysis buffer (Sigma, St Louis, MO) according to the manufacturer’s instructions. The expression patterns of DOCK9-AS2, GAPDH and U6 in cytoplasm and nuclear fractions were measured by qRT-PCR analysis, respectively.
mRNA stability analysis
Cells were treated with Actinomycin D, and qRT-PCR was used to assess the level of Wnt5a. The mRNA decay kinetics was assessed by One-phase exponential decay curve analysis (Sigma Plot) and the time corresponding to 50% remaining mRNA was considered as mRNA half- life according to a previously reported study [Citation18].
RNA immunoprecipitation (RIP)
A Magna RIP kit (Millipore, Billerica, MA, USA) was used for RIP assay. Cells were harvested and lysed in RIP lysis buffer. RIP assay was carried out according to the protocol of the Magna RIP kit. The RNA-protein complexes were immunoprecipitated with an anti-IgG antibody (AP112, Sigma) or anti-Ago2 antibody (ab32381, Abcam). The level of DOCK9-AS2 in immunoprecipitated RNA was isolated, purified, and determined by qRT-PCR analysis.
Transwell assay
For the migration assay, the VSMCs in serum-free DMEM were placed in the upper chamber of Transwell chemotaxis 24-well chamber (BD Biosciences). Lower chamber was filled with DMEM (600 μl) containing 10% FBS. After incubation for 24 h, cells removed from the upper layer to the lower layer. The cells in the lower layer were fixed with 4% paraformaldehyde and stained with 0.1% crystal violet. Cell number was counted using an inverted microscope (Nikon) [Citation19].
Western blot analysis
Radioimmunoprecipitation assay (RIPA) buffer (Beyotime Biotechnology) was used to collect the protein from VSMCs. Then the protein concentration was measured by bicinchoninic acid (BCA) kit (Beyotime Biotechnology). The proteins (30 μg/lane) were separated by 10% sodium dodecyl sulfate polyacrylamide gel and transferred onto a polyvinylidene fluoride (PVDF) membrane (EMd Millipore). After blocked in skimmed milk at room temperature for 2 h, the membranes were incubated with primary antibodies against Wnt5a (1: 1, 000; cat. no. ab179824, Abcam), EIF4A3 (1: 1, 000; cat. no. ab32485, Abcam), FBL (1: 500, cat. no. ab4566, Abcam), NOP 56 (1: 1, 000; cat. no. A18693, ABclonal), FUS (1: 1, 000; cat. no. ab124923, Abcam), IGF2BP3 (1: 1, 000; cat. no. A4444, ABclonal), LIN28 (1: 1, 000; cat. no. ab63740, Abcam), LIN28B (1: 2, 000; cat. no. ab191881, Abcam) and GAPDH (1: 1, 000; cat. no. cat. no. ab8245, Abcam) overnight at 4 °C. On the following day, the membranes were incubated with goat anti-rabbit horseradish peroxidase-conjugated secondary antibody (1:1,000; cat. no. ab6721; Abcam). The protein bands were visualized by enhanced chemiluminescence detection reagents (Beyotime Biotechnology) and analyzed with Image J software (National Institutes of Health). GAPDH served as the internal reference.
Statistical analysis
The experiments were conducted at least three times with three replicates. The data were presented as mean ± standard deviation. GraphPad Prism 8.0 was used to assess the difference between two or more groups compared by Student’s t-test or ANOVA followed with Tukey’s post hoc test. P < 0.05 was considered as statistically significant.
Results
DOCK9-AS2 knockdown inhibited the proliferation and migration of VSMCs in AS
VSMCs was induced by ox-LDL to mimic atherosclerosis in vitro, and DOCK-AS2 was significantly increased after ox-LDL induction. To investigate the role of DOCK9-AS2 in atherosclerosis, the ox-LDL-induced VSMCs were transfected with sh-DOCK9-AS2 or shNC. The results showed that DOCK9-AS2 was successfully inhibited by transfection with shDOCK-AS2 (). A). Then, the CCK-8 assay revealed that ox-LDL greatly improved cell viability in a time-dependent manner, and DOCK9-AS2 knockdown suppressed this elevated cell viability (). B). Immunofluoresence assay exhibited that Ki67 was greatly increased after ox-LDL induction, which was suppressed by DOCK9-AS2 knockdown (). C-D). In addition, we also investigated the effect of DOCK9-AS2 on ox-LDL-induced cell migration in VSMCs. The results in ). E-F showed that DOCK9-AS2 inhibited cell migration rate. Moreover, compared to the control, ox-LDL led to a low expression of E-cadherin and a high expression of N-cadherin; however, these changes were partly hindered by DOCK9-AS2 knockdown (). A-D). The above results indicate that DOCK9-AS2 knockdown inhibited ox-LDL-induced proliferation and migration of VSMCs.
Figure 1. The effect of ox-LDL and DOCK9-AS2 knockdown on the proliferation and migration of VSMCs. (Figure 1a. A) VSMCs were treated with ox-LDL and transfected with shNC or shDOCK9-AS2, and the relative mRNA level of DOCK9-AS2 in VSMCs was detected using qRT-PCR. (Figure 1a. B) The OD value at 450 nm was detected by CCK-8 assay. (Figure 1a. C-D) The expression of Ki67 was assessed by immunofluorescent staining. nuclei were stained with DAPI (blue); Magnification, x200. (Figure 1a. E-F) Cell migration ability was analyzed by Transwell assay. (Figure 1b. A-D) The protein expression of E-Cadherin and N-Cadherin was measured by immunofluorescent staining (nuclei were stained with DAPI; Magnification, x200). *** P < 0.001 vs. the control group; ## P < 0.01, ### P < 0.001 vs. the ox-LDL group.
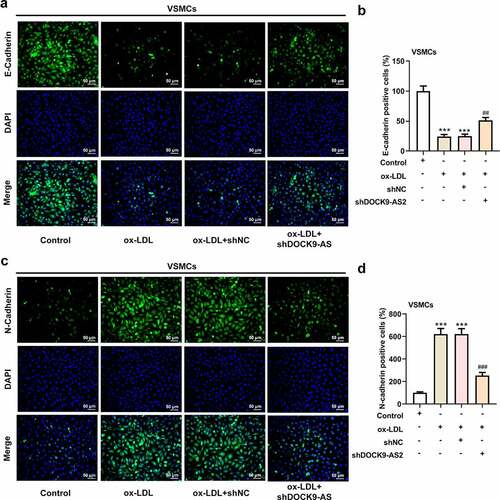
There is no direct interaction between DOCK9-AS2 and Wnt5a
To verify the relationship between lncRNA DOCK9-AS2 and Wnt5a, we tested the mRNA level and protein level of Wnt5a after transfection with shDOCK9-AS2 into VSMCs. The results showed that the expression level of Wnt5a was upregulated after ox-LDL induction, which was obviously inhibited upon DOCK9-AS2 knockdown (. A-B). Thus, we supposed that there was some connection between DOCK9-AS2 and Wnt5a. The results of nucleocytoplasmic separation demonstrated that DOCK9-AS2 revealed a dominant cytoplasmic distribution (. C). Thus, we speculated that DOCK9-AS2 might function at the post-transcriptional level in VSMCs. The results from RIP assay exhibited that There was no direct interaction between DOCK9-AS2 and Wnt5a (. D). However, the Wnt5a mRNA decay is slower in shDOCK9-AS2 group than that in sh-NC group (. E). Finally, the role of Wnt5a in the proliferation and migration of VSMCs was explored. The results indicated Wnt5a knockdown also inhibited the proliferation and migration of ox-LDL-induced VSMCs (. F-J).
Figure 2. The relationship between DOCK9-AS2 and Wnt5a. (a) VSMCs were treated with ox-LDL and transfected with shNC or shDOCK9-AS2, and the relative mRNA level of Wnt5a in VSMCs was detected using qRT-PCR. (b) The protein expression of Wnt5a in VSMCs was measured by Western blot. (c) The distribution of DOCK9-AS2 was assessed by nucleocytoplasmic separation experiment. (d) The direct interaction between DOCK9-AS2 and Wnt5a was assessed by RIP assay. (e) The remaining Wnt5a mRNA with the prolonging of time when ox-LDL-induced VSMCs were transfected with shNC/shDOCK9-AS2. (f) In ox-LDL-induced VSMCs, relative mRNA level of Wnt5a after interference with Wnt5a was assessed by qRT-PCR. (g) The protein level of Wnt5a after interference with Wnt5a was assessed by Western blot. (h) Cell proliferation was detected by CCK-8 assay. (i-g) Cell migration was analyzed by transwell assay. ** P < 0.01, *** P < 0.001 vs. the control group; ### P < 0.001 vs. the ox-LDL group.
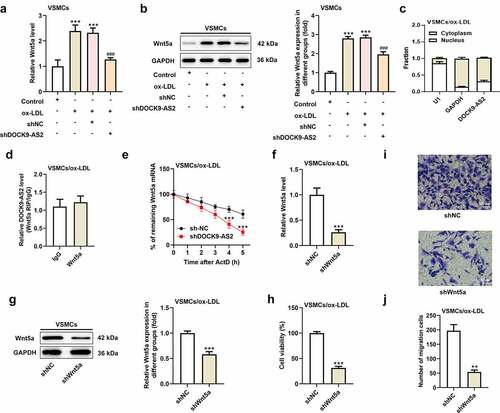
DOCK9-AS2 interacts with Wnt5a by targeting LIN28B
Considering that there was no direct relationship between DOCK9-AS2 and Wnt5a, we used Starbase database to find the targets that combined with DOCK9-AS2 and Wnt5a. Seven targets were found as follows: LIN28B, LIN28, IGF2BP3, FUS, FBL, EIF4A3, and NOP 56. Among these target genes, ox-LDL could up-regulate the protein expression of LIN28B, LIN28 and IGF2BP3 in VSMCs (). A-G). Next, pull down assay was used to validate genes binding to DOCK9-AS2 and Wnt5a. We found that LIN28B interacted with both DOCK9-AS2 and Wnt5a (). A-B). RIP assay further confirmed that LIN28B could bind to both DOCK9-AS2 and Wnt5a (). C-D). Afterward, the ox-LDL-induced VSMCs were transfected with sh-LIN28B and shNC, respectively, and the protein expression of LIN28B was obviously reduced upon sh-LIN28B transfection. In addition, the inhibition of LIN28B also downregulated the protein expression of Wnt5a in ox-LDL-induced VSMCs (). E). Moreover, Wnt5a mRNA decay was also slower in sh-LIN28B group than that in sh-NC group (). F). These results above indicated that LIN28B was an important mediator that both bound to DOCK9-AS2 and Wnt5a.
Figure 3. DOCK9-AS2 interact with Wnt5a by targeting LIN28B. (Figure 3a. A-G) The protein expression of EIF4A3, FBL, NCP56, FUS, IGF2BP3, LIN28, LIN28B in VSMCs treated with ox-LDL. (Figure 3b. A) The interaction between DOCK9-AS and LIN28, LIN28B and IGF2BP3 was assessed by biotinylated RNA pull down assay. (Figure 3b. B) The interaction between Wnt5a and LIN28, LIN28B and IGF2BP3 was assessed by biotinylated RNA pull down assay. (Figure 3b. C) The direct interaction between DOCK9-AS2 and LIN28B was measured by RIP. (Figure 3b. D) The direct interaction between Wnt5a and LIN28B was measured by RIP. (Figure 3b. E) The protein level of LIN28B and Wnt5a was observed upon sh-LIN28B transfection in ox-LDL-induced VSMCs. (Figure 3b. F) Wnt5a mRNA decay was observed upon knockdown of LIN28B group ** P < 0.01, *** P < 0.001 vs. the control group or Bio-NC group.
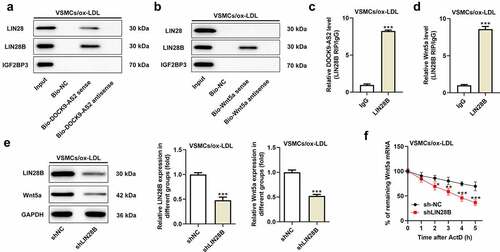
Overexpression of Wnt5a attenuated the reduced cell proliferation and migration induced by LIN28B knockdown
To further explore the important regulatory role of LIN28B in ox-LDL-induced VSMCs, the ox-LDL-induced VSMCs were co-transfected with shLIN28B and pc-Wnt5a or pcDNA3.1. pc-Wnt5a caused a greatly increase of Wnt5a mRNA expression in VSMCs (). A). Next, the CCK-8 assay revealed that interference of LIN28B obviously inhibited cell viability, which was partly reversed by Wnt5a overexpression (). B). In addition, the results in ). C-D showed that Ki67-positive cells were decreased after LIN28B knockdown. Meanwhile, the migrated cells were declined after LIN28B knockdown (). E-F), accompanied with reduced N-cadherin positive cells but elevated E-cadherin positive cells upon LIN28B knockdown (). A-D). The results demonstrated that LIN28B knockdown inhibited cell proliferation and migration. However, these changes were partly hindered by Wnt5a overexpression, indicating that overexpression of Wnt5a could weaken the inhibitory effects of LIN28B knockdown on cell proliferation and migration in ox-LDL-induced VSMCs.
Figure 4. Overexpression of Wnt5a reversed the reduced cell proliferation and migration induced by LIN28B knockdown. (Figure 4a. A) When ox-LDL-induced VSMCs were transfected with pc-Wnt5a or pcDNA3.1, the mRNA expression of Wnt5a was detected using qRT-PCR. ***p < 0.001. (Figure 4a. B) The ox-LDL-induced VSMCs were co-transfected with shLIN28B and pc-Wnt5a or pcDNA3.1, and the ability of cell proliferation was detected by CCK-8 assay. (Figure 4a. C-D) The expression of Ki67 was assessed by immunofluorescent staining. (Figure 4a. E-F) Cell migration was analyzed by transwell assay. (Figure 4b. A-D) The expression of E-Cadherin and N-Cadherin measured by immunofluorescent staining (nuclei were stained with DAPI; Magnification, x200). ** P < 0.01, *** P < 0.001 vs. the shNC group; # P < 0.05, ### P < 0.001 vs. shLIN28B + pcDNA3.1 group.
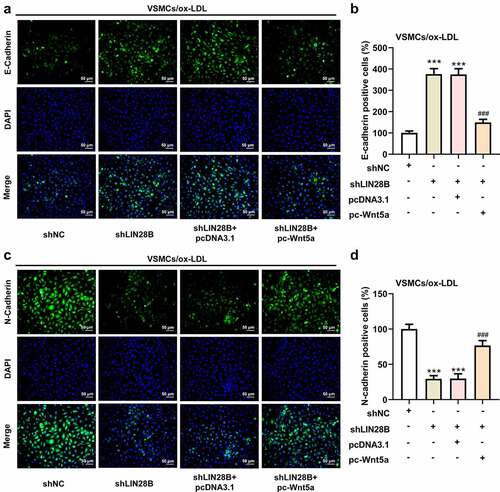
Overexpression of Wnt5a attenuated the reduced cell proliferation and cell migration induced by DOCK9-AS2 knockdown
Finally, we investigated the effect of overexpression of Wnt5 on DOCK9-AS2 knockdown-mediated cell proliferation and migration in ox-LDL-induced VSMCs. The results from CCK-8, immunofluorescence and Transwell assays revealed DOCK9-AS2 knockdown significantly reduced cell viability, the migrated cells, Ki67-positive cells and N-cadherin-positive cells but elevated E-cadherin-positive cells, whereas these alternations were partly abolished by Wnt5a overexpression (). A-E and ). A-D). These results indicated that overexpression of Wnt5a could weaken the inhibitory effects of DOCK9-AS2 knockdown on cell proliferation and migration in ox-LDL-induced VSMCs.
Figure 5. Overexpression of Wnt5a can reverse reduced cell proliferation and cell migration induced by DOCK9-AS2 knockdown. (Figure 5a. A) The ox-LDL-induced VSMCs were co-transfected with shDOCK9-AS2 and pc-Wnt5a or pcDNA3.1. Cell ability detected by CCK-8 assay. (Figure 5a. B-C) The expression of Ki67 was assessed by immunofluorescent staining. (Figure 5a. D-E) Cell migration was analyzed by transwell assay. (Figure 5b. A-D) The protein expression of E-Cadherin and N-Cadherin was measured by immunofluorescent staining (nuclei were stained with DAPI; Magnification, x200). *** P < 0.001 vs. the shNC group; # P < 0.05, ### P < 0.001 vs. shDOCK9-AS2 + pcDNA3.1 group.
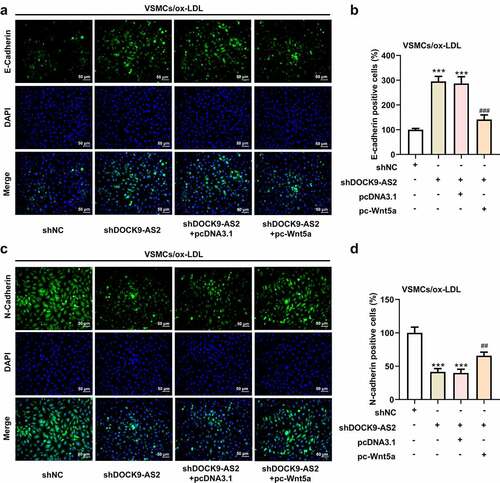
Discussion
Studies have shown that lncRNAs may play an important role in atherosclerosis. Previous study has found that lncRNA DOCK9-AS2 may play an important role in atherosclerosis. In our study, it was found for the first time that interference of DOCK9-AS2 suppressed ox-LDL-induced proliferation and migration in vascular smooth muscle cells. Further research demonstrated that DOCK9-AS2 stabilized Wnt5a by targeting LIN28B.
More and more studies have shown that members of the Wnt family, including Wnt1, Wnt2, Wnt3, Wnt4 and Wnt5a, play an important role in the occurrence and development of AS [Citation12,Citation20–23]. Wnt4 can induces VSMCs proliferation and is associated with intimal thickening [Citation21]. LncRNA H19 can regulate Wnt1/β-catenin signaling to promote proliferation and anti-apoptosis in ox-LDL-stimulated vascular smooth muscle cells [Citation22]. The major members involved in the progression of atherosclerosis are Wnt5a and Wnt1, of which Wnt5a is more widely studied. Therefore, in this study, we investigated the role of Wnt5a in ox-LDL-induced vascular smooth muscle cells. It has been reported that Wnt5a was highly expressed in both of atherosclerotic clinical samples and apoE−/− mice. Wnt5a knockdown significantly inhibited inflammatory response and cholesterol accumulation in atherosclerosis. On the contrary, overexpression of Wnt5a promoted cholesterol accumulation and inflammatory cytokine secretion [Citation12]. Consistent with previous studies, Wnt5a expression was increased in atherosclerotic vascular smooth muscle cells. Overexpression of Wnt5a promoted the proliferation and migration of ox-LDL-induced VSMCs, suggest that Wnt5a might promote the progression of atherosclerosis. Interestingly, Ackers et al. [Citation24] found that Wnt5a might promote differentiation of the human VSMCs into foam cells, indirectly aggravating atherosclerosis, in line with the findings in this study. Of note, the expression of Wnt5a was decreased when DOCK9-AS2 or LIN28B was silenced, indicating a potential regulatory role of DOCK9-AS2/LIN28B on Wnt5a. Further research exhibited that there was no direct interaction between DOCK9-AS2 and Wnt5a, indicating that DOCK9-AS2 might regulate Wnt5a through other pathways. Interestingly, pull down assay revealed that LIN28B was a potential interacted gene that both directly bound to Wnt5a and DOCK9-AS2. The following cellular biological assays exhibited that the inhibitory effects of DOCK9-AS2/LIN28B interference on ox-LDL-induced cell proliferation and migration in VSMCs were partly abolished by Wnt5a overexpression. These results suggested that DOCK9-AS2 might exert its effects on ox-LDL-induced VSMCs through binding to LIN28B, and thus regulating Wnt5a.
LIN28 is a highly conserved RNA binding protein, which contains two homologous genes, LIN28A and LIN28B. LIN28B is widely expressed in a number of tissues an functions in a wide spectrum of biological processes, including organismal growth, metabolism, and tumorigenesis [Citation25–27]. Many studies have shown that LIN28B can promote the proliferation and migration of tumor cells, thus affecting the occurrence of development of various cancers [Citation28]. LIN28B can promote the progression and metastasis of pancreatic ductal adenocarcinoma [Citation29]. LIN28B also regulates transcription and potentiates MYCN-induced neuroblastoma through binding to zinc finger protein 143 (ZNF143) at target gene promotors [Citation30]. In addition, LIN28B-AS1-IGF2BP1 binding promotes hepatocellular carcinoma cell progression [Citation31]. However, up to date, the role of LIN28B in atherosclerosis has not been previously described. Interestingly, Zhang W et al. found an aberrantly high expression of LIN28B in high glucose-induced human umbilical vein endothelial cells, which is a classical vascular inflammatory model of endothelial dysfunction, a primary cause of diabetes-related vascular complications, including atherosclerosis, indicating that LIN28B might be correlated with the pathological process of atherosclerosis [Citation32]. Here, in the present study, LIN28B was expected to be upregulated in ox-LDL-induced VSMCs. In addition, interference of LIN28B was found to suppress cell proliferation and migration in ox-LDL-induced VSMCs, which was partly hindered by Wnt5a, emphasizing the regulatory connection between LIN28B and Wnt5a. Given that LIN28B was the only one protein that both interacted with Wnt5a and DOCK9-AS2, and DOCK9-AS2 directly bound to LIN28B and indirectly regulated the stability of Wnt5a, LIN28B might be the critical protein which act as the bridge between DOCK9-AS2 and Wnt5a, and DOCK9-AS2/LIN28B/Wnt5a might be an effective pathway for ox-LDL-induced cell injury in VSMCs.
Conclusions
In conclusion, our results demonstrate for the first time that DOCK9-AS2 and LIN28B can promote the proliferation and migration of ox-LDL-induced vascular smooth muscle cells, and DOCK9-AS2 stabilize Wnt5a by targeting LIN28B. The findings in this study raised up critical genes in in vitro model for atherosclerosis, and disclosed the novel molecular mechanism.
Availability of Data and Materials
All data generated during this study are included in this published article.
Author’s contributions
ZT raised the concept, designed the experiments, made interpretation to the results, and revised the manuscript;
JS conducted the experiment, analyzed the data, made interpretation to the results and wrote the manuscript;
BZ conducted the experiment and wrote the manuscript
Disclosure statement
No potential conflict of interest was reported by the author(s).
Additional information
Funding
References
- Chistiakov DA, Melnichenko AA, Grechko AV, et al. Potential of anti-inflammatory agents for treatment of atherosclerosis. Exp Mol Pathol. 2018;104(2):114–124.
- Zhu J, Liu B, Wang Z, et al. Exosomes from nicotine-stimulated macrophages accelerate atherosclerosis through miR-21-3p/PTEN-mediated VSMC migration and proliferation. Theranostics. 2019;9(23):6901–6919.
- Basatemur GL, Jorgensen HF, Clarke MCH, et al. Vascular smooth muscle cells in atherosclerosis. Nat Rev Cardiol. 2019;16(12):727–744.
- Yoon JH, Kim J, Gorospe M. Long noncoding RNA turnover. Biochimie. 2015;117:15–21.
- Pan JX. LncRNA H19 promotes atherosclerosis by regulating MAPK and NF-kB signaling pathway. Eur Rev Med Pharmacol Sci. 2017;21(2):322–328.
- Wang CH, Shi HH, Chen LH, et al. Identification of Key lncRNAs Associated With Atherosclerosis Progression Based on Public Datasets. Front Genet. 2019;10:123.
- Fernandez-Ruiz I. Atherosclerosis: a new role for lncRNAs in atherosclerosis. Nat Rev Cardiol. 2018;15(4):195.
- Jian L, Jian D, Chen Q, et al. Long Noncoding RNAs in Atherosclerosis. J Atheroscler Thromb. 2016;23(4):376–384.
- Dai W, Jin X, Han L, et al. Exosomal lncRNA DOCK9-AS2 derived from cancer stem cell-like cells activated Wnt/beta-catenin pathway to aggravate stemness, proliferation, migration, and invasion in papillary thyroid carcinoma. Cell Death Dis. 2020;11(9):743.
- Shao Y, Zheng Q, Wang W, et al. Biological functions of macrophage-derived Wnt5a, and its roles in human diseases. Oncotarget. 2016;7(41):67674–67684.
- Arredondo SB, Guerrero FG, Herrera-Soto A, et al. Wnt5a promotes differentiation and development of adult-born neurons in the hippocampus by noncanonical Wnt signaling. Stem Cells. 2020;38(3):422–436.
- Zhang CJ, Zhu N, Liu Z, et al. Wnt5a/Ror2 pathway contributes to the regulation of cholesterol homeostasis and inflammatory response in atherosclerosis. Biochim Biophys Acta Mol Cell Biol Lipids. 2020;1865(2):158547.
- Pashirzad M, Shafiee M, Rahmani F, et al. Role of Wnt5a in the Pathogenesis of Inflammatory Diseases. J Cell Physiol. 2017;232(7):1611–1616.
- Yang L, Chu Y, Wang Y, et al. siRNA-mediated silencing of Wnt5a regulates inflammatory responses in atherosclerosis through the MAPK/NF-kappaB pathways. Int J Mol Med. 2014;34(4):1147–1152.
- Ackers I, Szymanski C, Duckett KJ, et al. Blocking Wnt5a signaling decreases CD36 expression and foam cell formation in atherosclerosis. Cardiovasc Pathol. 2018;34:1–8.
- Qin L, Hu R, Zhu N, et al. The novel role and underlying mechanism of Wnt5a in regulating cellular cholesterol accumulation. Clin Exp Pharmacol Physiol. 2014;41(9):671–678.
- Barnes C, Kanhere A. Identification of RNA-Protein Interactions Through In Vitro RNA Pull-Down Assays. Methods Mol Biol. 2016;1480:99–113.
- Al-Kharashi LA, Al-Mohanna FH, Tulbah A, et al. The DNA methyl-transferase protein DNMT1 enhances tumor-promoting properties of breast stromal fibroblasts. Oncotarget. 2018;9(2):2329–2343.
- Du W, Feng Z, Sun Q. LncRNA LINC00319 accelerates ovarian cancer progression through miR-423-5p/NACC1 pathway. Biochem Biophys Res Commun. 2018;507(1–4):198–202.
- Boucher P, Matz RL, Terrand J. atherosclerosis: gone with the Wnt? Atherosclerosis. 2020;301:15–22.
- Tsaousi A, Williams H, Lyon CA, et al. Wnt4/beta-catenin signaling induces VSMC proliferation and is associated with intimal thickening. Circ Res. 2011;108(4):427–436.
- Zhang L, Cheng H, Yue Y, et al. H19 knockdown suppresses proliferation and induces apoptosis by regulating miR-148b/WNT/beta-catenin in ox-LDL -stimulated vascular smooth muscle cells. J Biomed Sci. 2018;25(1):11.
- Zhuang JB, Li T, Hu XM, et al. Circ_CHFR expedites cell growth, migration and inflammation in ox-LDL-treated human vascular smooth muscle cells via the miR-214-3p/Wnt3/beta-catenin pathway. Eur Rev Med Pharmacol Sci. 2020;24(6):3282–3292.
- Ackers I, Szymanski C, Silver MJ, et al. Oxidized Low-Density Lipoprotein Induces WNT5A Signaling Activation in THP-1 Derived Macrophages and a Human Aortic Vascular Smooth Muscle Cell Line. Front Cardiovasc Med. 2020;7:567837.
- Sato T, Kataoka K, Ito Y, et al. Lin28a/let-7 pathway modulates the Hox code via Polycomb regulation during axial patterning in vertebrates. Elife. 2020;9. DOI:10.7554/eLife.53608.
- Shyh-Chang N, Daley GQ. Lin28: primal regulator of growth and metabolism in stem cells. Cell Stem Cell. 2013;12(4):395–406.
- Farzaneh M, Attari F, Khoshnam SE. Concise Review: LIN28/let-7 Signaling, a Critical Double-Negative Feedback Loop During Pluripotency, Reprogramming, and Tumorigenicity. Cell Reprogram. 2017;19(5):289–293.
- Lovnicki J, Gan Y, Feng T, et al. LIN28B promotes the development of neuroendocrine prostate cancer. J Clin Invest. 2020;130(10):5338–5348.
- Kugel S, Sebastian C, Fitamant J, et al. SIRT6 Suppresses Pancreatic Cancer through Control of Lin28b. Cell. 2016;165(6):1401–1415.
- Tao T, Shi H, Mariani L, et al. LIN28B regulates transcription and potentiates MYCN-induced neuroblastoma through binding to ZNF143 at target gene promotors. Proc Natl Acad Sci U S A. 2020;117(28):16516–16526.
- Zhang J, Hu K, Yang YQ, et al. LIN28B-AS1-IGF2BP1 binding promotes hepatocellular carcinoma cell progression. Cell Death Dis. 2020;11(9):741.
- Zhang W, Sui Y. CircBPTF knockdown ameliorates high glucose-induced inflammatory injuries and oxidative stress by targeting the miR-384/LIN28B axis in human umbilical vein endothelial cells. Mol Cell Biochem. 2020;471(1–2):101–111.