ABSTRACT
Adipose-derived mesenchymal stem cells (ADSCs) are a class of pluripotent stem cells isolated from the adipose tissue; they can differentiate into osteoblasts after induction and play an important role in bone repair. EGFL6 protein is secreted by adipocytes and osteoblasts and can promote endothelial cell migration and angiogenesis. This study aimed to explore the effect of recombinant EGFL6 protein on the osteogenic differentiation of ADSCs. The cells were incubated with fluorescein isothiocyanate-conjugated antibodies and analyzed by flow cytometry. Alizarin red staining and alkaline phosphatase staining were used to detect the osteogenic differentiation ability. mRNA expression was analyzed by real-time quantitative polymerase chain reaction (RT-qPCR). Protein expression was determined using Western blotting. The osteogenic differentiation ability of ADSCs isolated from the adipose tissue was significantly weakened after EGFL6 knockdown; this ability was restored upon the addition of EGFL6 recombinant protein. BMP2 knockdown inhibited the effect of EGFL6 recombinant protein on osteogenic differentiation. EGFL6 recombinant protein promoted osteogenic differentiation of ADSCs through the BMP2/SMAD4 signaling pathway. This may provide a potential target for the osteogenic differentiation of ADSCs.
1. Introduction
Bone metabolism is a dynamic balanced process in which osteoclasts (OCs) absorb old bone and osteoblasts (OBs) to form new bone [Citation1]. The imbalance between OC and OB induces metabolic bone diseases such as osteoporosis. With the aging population, the incidence of osteoporosis is increasing every year [Citation2]. Additionally, osteoporosis-induced bone fractures reduce the quality of life of the elderly population [Citation3]. Therefore, promotion of bone formation has been the focus of research in recent years. Bone formation is generally regulated by various signaling pathways, including BMP/Smad, PI3K/AKT, Wnt/β-catenin, MAPK, Notch, and Hedgehog [Citation4–7]. Identification of upstream regulatory factors may provide new therapeutic targets for metabolic bone diseases.
Patient-derived cell therapy is an effective method for treating bone-related diseases. Human mesenchymal stem cells (MSCs) are abundant autologous cells that can differentiate into multiple cell lines in vitro and in vivo and have been recognized as an ideal stem cell source for cell therapy [Citation8,Citation9]. Adipose stem cells (ADSCs) are MSCs present in the adipose tissue and can differentiate into various cells and tissues under specific induction conditions [Citation10]. Experiments have shown that ADSCs have therapeutic effects on various diseases such as skeletal and muscle diseases [Citation11], cardiovascular diseases [Citation12], and autoimmune diseases [Citation13]. ADSCs can differentiate into osteoblasts, lipids, and chondroblasts, similar to classic bone marrow-derived mesenchymal stem cells (BMSCs) [Citation14]. In addition, compared to BMSCs, ADSCs have the characteristics of extensive sources, easy materials, and simple operation [Citation15,Citation16]. Therefore, ADSCs have great potential in the treatment of bone-related diseases.
Epidermal growth factor-like domain protein 6 (EGFL6), located on chromosome Xp22, is a member of the EGF repeat domain superfamily [Citation17]. EGFL6 has been reported to be expressed in early developing adipose tissue, is secreted by adipocytes, and promotes proliferation of adjacent vascular endothelial cells [Citation18,Citation19]. Studies have shown that EGFL6 is abnormally expressed in some tumor tissues [Citation20,Citation21]. In addition, EGFL6 can be secreted by osteoblast-like cells to promote endothelial cell migration and angiogenesis through the ERK signaling pathway [Citation22]. To date, the potential expression and role of EGFL6 in bone biology remain to be elucidated.
Therefore, this study aimed to explore the function and role of EGFL6 in osteogenic differentiation. We hypothesized that EGFL6 promoted the osteogenic differentiation of ADSCs through the BMP2/SMAD4 signaling pathway.
2. Materials and methods
2.1 Cell culture
The adipose tissues were obtained from seven donors, and ADSCs were isolated according to the modified protocol reported by Zuk et al. [Citation23]. The ADSCs were cultured in osteogenic medium (OM) consisting of proliferation medium, 100 nM dexamethasone (Sigma-Aldrich), 20 μM L-ascorbic acid (Wako Pure Chemical Industries Ltd), 10 mM β-glycerol phosphate (Sigma-Aldrich), and 1% (v/v) penicillin/streptomycin. The cells were maintained in humidity of 95% with 5% CO2 at 37°C. ADSCs at passage 3 were selected for further experiments.
The small interfering RNA EGFL6 (si-EGFL6 1# and 2#), si-BMP2, and their negative controls (si-nc) were synthesized by GenePharma (Shanghai, China). All these plasmids were transfected into the cells by using Lipofectamine 2000 (Invitrogen, Carlsbad, USA) for 48 h at 37°C.
2.2 Flow cytometry assay
According to a previous study [Citation24], the cells were incubated with fluorescein isothiocyanate-conjugated antibodies (anti-CD90, anti-CD105, anti-CD44, anti-CD14, and anti-CD106) (BioLegend, San Diego, CA, USA) and analyzed using NovoCyte Advanteon B4 Flow Cytometer and NovoSampler Q software (Agilent Technologies Co., Ltd).
2.3 Alizarin red staining
According to a previous study [Citation25], ADSCs were cultured in 35-mm plates for 14 days. After fixation in 95% ethanol, the ADSCs were stained with 1% Alizarin red S (ARS) staining solution (Sigma‐Aldrich) at room temperature for 15 min. To quantify the mineralization levels, densitometric analysis of staining was performed using ImageJ 1.48.
2.4 Alkaline phosphatase staining and activity
According to a previous study [Citation26], cells were fixed with 4% paraformaldehyde (PFA) for 10 min at room temperature. The cells were then seeded and stained with ALP for 20 min. Images were captured using a scanner. The ALP Assay Kit was used to detect ALP activity according to the manufacturer’s protocol.
2.5 RT-qPCR
According to a previous study [Citation27], RNA was extracted from ADSCs using a commercially available kit (Takara, Japan). cDNA was then synthesized, and PCR was carried out using a Real-Time PCR Detection System (Bio-Rad, USA). The sequences of the primers used were as follows: EGFL6: forward: 5′-AAGCTTGGATCCGAATTCAGTATGCAGCCGCCCTGG-3′, reverse: 5′-CTCGAGTCTAGAAGATCTACCTTCTACAGATAAAAAGT-3′; Runx2: forward: 5′-CGCATTCCTCATCCCAGTAT-3′, reverse: 5′-TGTAGGTAAAGGTGGCTGGG-3′; osteocalcin: forward: 5′-GCGCTCTGTCTCTCGTGACCT-3′, reverse, 5′-ATAGATGCGTTTGTAGGCGG-3′; osteopontin: forward: 5′-TCTGATGAGACCGTCACTGC-3′, reverse: 5′-TCTCCTGGCTCTCTTTGGAA-3′; BMP2: forward: 5′-GACTGCGGTCTCCTAAAGGTCG-3′, reverse: 5′- CTGGGGAAGCAGCAACGCTA-3′; SMAD4: forward: 5′- CCATCAGTCTGTCTGCTGCT-3′, reverse: 5′-TGATGCTCTGTCTCGGGTAG-3′; GAPDH: forward: 5′-GAAGGTGAAGGTCGGAGTC-3′, reverse: 5′- GAAGATGGTGATGGGATTTC-3′; and U6: forward: 5′-CTCGCTTCGGCAGCACA-3′, reverse: 5′- AACGCTTCACGAATTTGCGT-3′.
2.6 Western blot
According to a previous study [Citation28], protein extracts were loaded onto 10% SDS gel and electrophoresed. The protein extracts were then transferred to the PVDF membrane (Millipore) and incubated with primary antibodies at 4°C overnight. The next day, the membrane was incubated with secondary antibodies for 2 h at room temperature. Finally, the protein bands were captured using ECL system (Thermo Fisher Scientific, Inc.).
2.7 Statistical analysis
Statistical analyses were performed using GraphPad Prism 7.0 (Graph-Pad Software, CA, USA). Data have been expressed as means ± standard deviation (SD). Differences between two groups were measured using Student’s t-tests. Comparisons between more than two groups were analyzed using analysis of variance. Statistical significance was set at P <0.05.
3. Results
This study demonstrated that EGFL6 knockdown decreased the red calcium nodules, the activity of blue ALP, and the mRNA levels of osteogenic differentiation-related genes. Recombinant EGFL6 promotes osteogenic differentiation of ADSCs by regulating the BMP2/SMAD4 signaling pathway.
3.1 Characterization of ADSCs
To identify the adipocytes, we detected the expression of positive markers CD44 (95.38%), CD90 (95.73%), and CD105 (96.26%), while negative markers CD14 as well as CD106 were 3.69% and 2.75%, respectively (). The cells had a typical spindle shape and multidirectional differentiation potential (). After induction, the cells could differentiate in osteogenic and adipogenic directions ().
3.2 EGFL6 knockdown attenuates the osteogenic differentiation of ADSCs
Compared with si-NC group, the mRNA and protein expression of EGFL6 were significantly decreased after si-EGFL6 1# and si-EGFL6 2# transfection, indicating that the transfection was successful, and si-EGFL6 1# was used in subsequent experiments (). Cell staining showed that red calcium nodules and the activity of blue ALP notably decreased after EGFL6 knockdown (). The mRNA levels of osteogenic differentiation-related genes RNUX2, osteopontin (OPN), and osteocalcin (OCN) were remarkably decreased, and the protein expression was also decreased ().
Figure 2. EGFL6 knockdown attenuates the osteogenic differentiation of ADSCs. (a) mRNA expression of EGFL6 in ADSCs. (b) Protein expression of EGFL6 in ADSCs. (c) ARS staining of ADSCs. (d) ALP activity of ADSCs. (e) mRNA expression of RUNX2 in ADSCs. (f) mRNA expression of OPN in ADSCs. (g) mRNA expression of OCN in ADSCs. (h) Protein expression of RUNX2, OPN, and OCN. **P < 0.01, *** P < 0.001 versus si-NC.
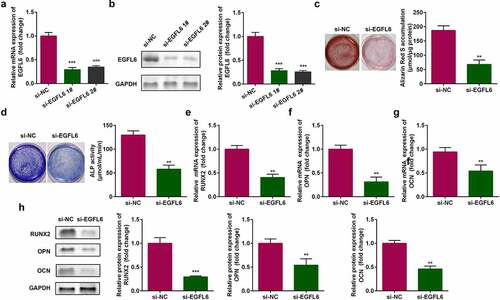
3.3 Recombinant EGFL6 promotes osteogenic differentiation of ADSCs
shows that after EGFL6 knockdown, when the cells were treated with EGFL6 recombinant protein, the red calcium nodules were markedly increased after cell staining, and the activity of blue ALP was also observably enhanced (). In addition, the addition of EGFL6 recombinant protein notably increased the mRNA and protein levels of RNUX2, OPN, and OCN ().
Figure 3. Recombinant EGFL6 promotes osteogenic differentiation of ADSCs. (a) ARS stain of ADSCs. (b) ALP activity of ADSCs. (c) mRNA expression of RUNX2 in ADSCs. (d) mRNA expression of OPN in ADSCs. (e) mRNA expression of OCN in ADSCs. (f) Protein expression of RUNX2, OPN, OCN. ##P < 0.01, **P < 0.01, ***P < 0.001 versus si-NC.
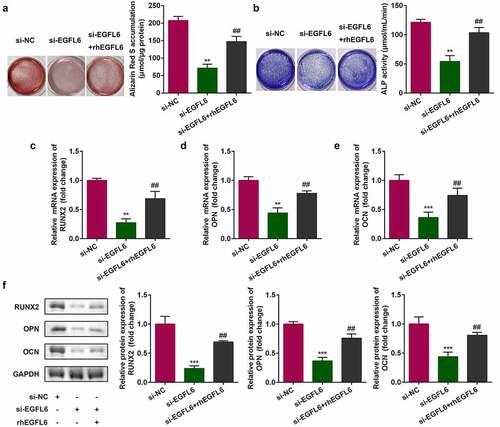
3.4 Recombinant EGFL6 enhances osteogenic differentiation through the BMP2/SMAD4 signaling pathway
In EGFL6 knockdown cells, the expression of BMP2 and SMAD4 decreased, while that of BMP2 and SMAD4 increased noticeably after adding recombinant EGFL6 protein (). Compared with the NC group, the mRNA level and protein expression of BMP2 were significantly decreased after knockdown, and the downstream SMAD4 protein expression was also decreased, indicating that cell transfection was successful. 1# was used for the subsequent experiments (). Compared with the si-NC group, red calcium nodules and the activity of blue ALP were significantly increased after adding recombinant protein EGFL6, while the effect was inhibited by BMP2 knockdown (). In addition, the inhibition of BMP2 in cells treated with recombinant EGFL6 markedly reduced the mRNA level and protein expression of RNUX2, OPN, and OCN ().
Figure 4. The expression of BMP2 and SMAD4 is positively correlated with EGFL6. (a) Protein expression of BMP2 as well as SMAD4. ##P < 0.01, ***P < 0.001 versus si-NC.
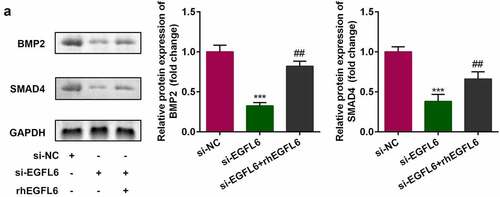
Figure 5. Recombinant EGFL6 enhances osteogenic differentiation through the BMP2/SMAD4 signaling pathway. (a) mRNA expression of BMP2. (b) Protein expression of BMP2 and SMAD4. (c) ARS staining of ADSCs. (d) ALP activity of ADSCs. (e) mRNA expression of RUNX2 in ADSCs. (f) mRNA expression of OPN in ADSCs. (g) mRNA expression of OCN in ADSCs. (h) Protein expression of RUNX2, OPN, and OCN. ##P < 0.01, **P < 0.01, ***P < 0.001 versus si-NC.
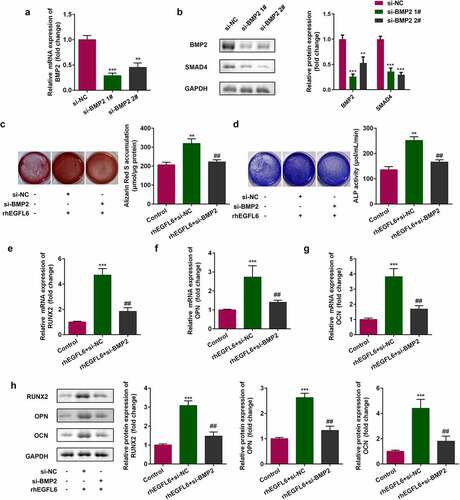
4. Discussion
Osteoblasts are mainly differentiated from mesenchymal stem cells and regulate the synthesis, secretion, and mineralization of the bone matrix and osteoclasts [Citation29,Citation30]. Studies have shown that mesenchymal stem cells can be isolated from the adipose tissue and obtained in large quantities [Citation31] and can be induced into osteoblasts, chondrocytes, adipocytes, and other directional transformation [Citation32–34]. In this study, the osteogenic differentiation of ADSCs was weakened after EGFL6 knockdown, while it was restored after the addition of recombinant EGFL6 protein. In addition, the expression of EGFL6 positively regulated BMP2 and SMAD4 proteins, suggesting that EGFL6 promoted the osteogenic differentiation of ADSCs through the BMP2/SMAD4 signaling pathway.
EGFL6 is a secretory protein with structural homology, and the gene is present on human Xp22 chromosome [Citation35]. It has been reported that EGFL6 is involved in the development of a variety of tumors, such as ovarian cancer [Citation36] and colon cancer [Citation37]; however, its role in the regulation of osteogenic differentiation of ADSCs is rarely studied. In this study, the osteogenic differentiation of cells was significantly weakened upon the knockdown of EGFL6 in ADSCs, whereas the ability of osteogenic differentiation was restored after the addition of EGFL6 recombinant protein. These results indicate that EGFL6 plays an important role in the regulation of the osteogenic differentiation of ADSCs.
BMPs are important members of TGF-β superfamily. BMP2, BMP4, BMP7, and BMP9 play important roles in the regulation of osteoblast differentiation [Citation38]. It has been reported that increasing the mRNA expression of BMP2 can significantly increase the expression of alkaline phosphatase, osteocalcin, Smad, and other osteogenic markers, as well as the formation of mineralized nodules, and the cells are significantly differentiated into osteoblasts [Citation39]. In this study, BMP2 and SMAD4 protein expression was positively correlated with EGFL6, and inhibition of BMP2 expression weakened the promotion of EGFL6 recombinant protein expression on osteogenic differentiation.
In general, EGFL6 recombinant protein can promote the osteogenic differentiation of ADSCs by regulating the BMP2/SMAD4 signaling pathway. Our research provided a novel method and theoretical basis for the clinical treatment of osteoporosis. In the future, we will focus on the clinical application of EGFL6. The role of EGFL6 in ADSCs was further proved by in vivo experiments.
Highlights
1. EGFL6 knockdown attenuated the osteogenic differentiation of ADSCs.
2. Recombinant EGFL6 promotes osteogenic differentiation of ADSCs.
3. The expression of BMP2 and SMAD4 was positively correlated with EGFL6.
Ethical approval
This study protocol was approved by the Ethics Committee of China Medical University.
Disclosure statement
No potential conflict of interest was reported by the authors.
Additional information
Funding
References
- Tsuchiya H, Kitoh H, Sugiura F, et al. Chondrogenesis enhanced by overexpression of sox9 gene in mouse bone marrow-derived mesenchymal stem cells. Biochem Biophys Res Commun. 2003;301(2):338–343.
- Shao H, Wu R, Cao L, et al. Trelagliptin stimulates osteoblastic differentiation by increasing runt-related transcription factor 2 (RUNX2): a therapeutic implication in osteoporosis. Bioengineered. 2021;12(1):960–968.
- Xia WB, He SL, Xu L, et al. Rapidly increasing rates of hip fracture in Beijing, China. J Bone Miner Res. 2012;27(1):125–129.
- Radio NM, Doctor JS, Witt-Enderby PA. Melatonin enhances alkaline phosphatase activity in differentiating human adult mesenchymal stem cells grown in osteogenic medium via MT2 melatonin receptors and the MEK/ERK (1/2) signaling cascade. J Pineal Res. 2006;40(4):332–342.
- Choi YH, Kim YJ, Jeong HM, et al. Akt enhances Runx2 protein stability by regulating Smurf2 function during osteoblast differentiation. FEBS J. 2014;281(16):3656–3666.
- Liu J, Yang J. Uncarboxylated osteocalcin inhibits high glucose-induced ROS production and stimulates osteoblastic differentiation by preventing the activation of PI3K/Akt in MC3T3-E1 cells. Int J Mol Med. 2016;37(1):173–181.
- Lin GL, Hankenson KD. Integration of BMP, Wnt, and notch signaling pathways in osteoblast differentiation. J Cell Biochem. 2011;112(12):3491–3501.
- Wu M, Chen G, Li YP. TGF-beta and BMP signaling in osteoblast, skeletal development, and bone formation, homeostasis and disease. Bone Res. 2016;4:16009.
- Xian L, Wu X, Pang L, et al. Matrix IGF-1 maintains bone mass by activation of mTOR in mesenchymal stem cells. Nat Med. 2012;18(7):1095–1101.
- Jiang W, Zhang J, Zhang X, et al. VAP-PLGA microspheres (VAP-PLGA) promote adipose-derived stem cells (ADSCs)-induced wound healing in chronic skin ulcers in mice via PI3K/Akt/HIF-1α pathway. Bioengineered. 2021;12(2):10264–10284.
- Han DS, Chang HK, Park JH, et al. Consideration of bone regeneration effect of stem cells: comparison between adipose-derived stem cells and demineralized bone matrix. J Craniofac Surg. 2014;25(1):189–195.
- Cai A, Qiu R, Li L, et al. Atorvastatin treatment of rats with ischemia-reperfusion injury improves adipose-derived mesenchymal stem cell migration and survival via the SDF-1alpha/CXCR-4 axis. PLoS One. 2013;8(12):e79100.
- Scruggs BA, Semon JA, Zhang X, et al. Age of the donor reduces the ability of human adipose-derived stem cells to alleviate symptoms in the experimental autoimmune encephalomyelitis mouse model. Stem Cells Transl Med. 2013;2(10):797–807.
- Konno M, Hamabe A, Hasegawa S, et al. Adipose-derived mesenchymal stem cells and regenerative medicine. Dev Growth Differ. 2013;55(3):309–318.
- Zuk PA, Zhu M, Mizuno H, et al. Multilineage cells from human adipose tissue: implications for cell-based therapies. Tissue Eng. 2001;7(2):211–228.
- Caviggioli F, Vinci V, Salval A, et al. Human adipose-derived stem cells: isolation, characterization and applications in surgery. ANZ J Surg. 2009;79:856.
- Yeung G, Mulero JJ, Berntsen RP, et al. Cloning of a novel epidermal growth factor repeat containing gene EGFL6: expressed in tumor and fetal tissues. Genomics. 1999;62(2):304–307.
- Buchner G, Broccoli V, Bulfone A, et al. MAEG, an EGF-repeat containing gene, is a new marker associated with dermatome specification and morphogenesis of its derivatives. Mech Dev. 2000;98:179–182.
- Oberauer R, Rist W, Lenter MC, et al. EGFL6 is increasingly expressed in human obesity and promotes proliferation of adipose tissue-derived stromal vascular cells. Mol Cell Biochem. 2010;343(1–2):257–269.
- Buckanovich RJ, Sasaroli D, O’Brien-Jenkins A, et al. Tumor vascular proteins as biomarkers in ovarian cancer. J Clin Oncol. 2007;25(7):852–861.
- Wang X, Gong Y, Wang D, et al. Analysis of gene expression profiling in meningioma: deregulated signaling pathways associated with meningioma and EGFL6 overexpression in benign meningioma tissue and serum. PLoS One. 2012;7(12):e52707.
- Chim SM, Qin A, Tickner J, et al. EGFL6 promotes endothelial cell migration and angiogenesis through the activation of extracellular signal-regulated kinase. J Biol Chem. 2011;286(25):22035–22046.
- Zuk PA, Zhu M, Ashjian P, et al. Human adipose tissue is a source of multipotent stem cells. Mol Biol Cell. 2002;13(12):4279–4295.
- Nugraha AP, Rantam FA, Narmada IB, et al. Gingival-derived mesenchymal stem cell from rabbit (Oryctolagus cuniculus): isolation, culture, and characterization. Eur J Dent. 2021;15(2):332–339.
- Bensimon-Brito A, Cardeira J, Dionísio G, et al. Revisiting in vivo staining with alizarin red S – a valuable approach to analyse zebrafish skeletal mineralization during development and regeneration. BMC Dev Biol. 2016;16(1):2.
- Wang Y, Xu W, Maddera L, et al. Alkaline phosphatase-based chromogenic and fluorescence detection method for BaseScope™ in situ hybridization. J Histotechnol. 2019;42(4):193–201.
- Hirotsu Y, Maejima M, Shibusawa M, et al. Analysis of a persistent viral shedding patient infected with SARS-CoV-2 by RT-qPCR, FilmArray Respiratory Panel v2.1, and antigen detection. J Infect Chemother. 2021;27(2):406–409.
- Mishra M, Tiwari S, Gomes AV. Protein purification and analysis: next generation Western blotting techniques. Expert Rev Proteomics. 2017;14(11):1037–1053.
- Seeman E, Delmas PD. Bone quality – the material and structural basis of bone strength and fragility. N Engl J Med. 2006;354(21):2250–2261.
- Hartmann C. A Wnt canon orchestrating osteoblastogenesis. Trends Cell Biol. 2006;16(3):151–158.
- Tapp H, Hanley EN Jr., Patt JC, et al. Adipose-derived stem cells: characterization and current application in orthopaedic tissue repair. Exp Biol Med (Maywood). 2009;234(1):1–9.
- Mahmoudifar N, Doran PM. Osteogenic differentiation and osteochondral tissue engineering using human adipose-derived stem cells. Biotechnol Prog. 2013;29(1):176–185.
- Zaminy A, Ragerdi Kashani I, Barbarestani M, et al. Osteogenic differentiation of rat mesenchymal stem cells from adipose tissue in comparison with bone marrow mesenchymal stem cells: melatonin as a differentiation factor. Iran Biomed J. 2008;12(3):133–141.
- Moioli EK, Chen M, Yang R, et al. Hybrid adipogenic implants from adipose stem cells for soft tissue reconstruction in vivo. Tissue Eng Part A. 2010;16(11):3299–3307.
- Chuang CY, Chen MK, Hsieh MJ, et al. High level of plasma EGFL6 is associated with clinicopathological characteristics in patients with oral squamous cell carcinoma. Int J Med Sci. 2017;14(5):419–424.
- Bai S, Ingram P, Chen YC, et al. EGFL6 regulates the asymmetric division, maintenance, and metastasis of ALDH+ ovarian cancer cells. Cancer Res. 2016;76(21):6396–6409.
- Song K, Su W, Liu Y, et al. Identification of genes with universally upregulated or downregulated expressions in colorectal cancer. J Gastroenterol Hepatol. 2019;34(5):880–889.
- Beederman M, Lamplot JD, Nan G, et al. BMP signaling in mesenchymal stem cell differentiation and bone formation. J Biomed Sci Eng. 2013;6(8):32–52.
- Toom A, Arend A, Gunnarsson D, et al. Bone formation zones in heterotopic ossifications: histologic findings and increased expression of bone morphogenetic protein 2 and transforming growth factors beta2 and beta3. Calcif Tissue Int. 2007;80(4):259–267.