ABSTRACT
Long non-coding RNA RGMB-AS1 (RGMB antisense RNA 1) plays a crucial role in tumor progression. However, its underlying mechanism in nasopharyngeal carcinoma (NPC) remains unclear. In this study, we analyzed the clinical significance of lncRNA RGMB-AS1 as a possible potential marker in NPC, and investigated the effect and mechanism of lncRNA RGMB-AS1 on proliferation, migration and epithelial mesenchymal transformation (EMT) of NPC by directly binding Forkhead box A1 (FOXA1) in vitro and in vivo. In conclusion, LncRNA RGMB-AS1 inhibits malignant behaviors and EMT by regulating FOXA1, and lncRNA RGMB-AS1 may be an important indicator of clinical prognosis.
Graphical Abstract
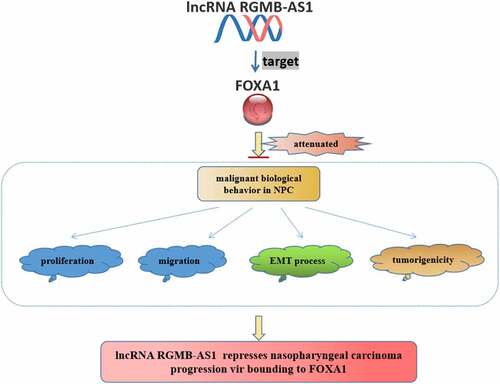
1 Introduction
Nasopharyngeal carcinoma (NPC) is one of the most common tumors in the head and neck, mainly concentrated in Southeast Asia, and it is the most common invasive squamous cell carcinoma [Citation1]. Early NPC patients may have symptoms such as bleeding in the nose, tinnitus and nasal congestion, etc. However, due to the lack of specificity of early symptoms, approximately 70% of patients at the first diagnosis have entered locally advanced stage [Citation2]. Moreover, the rates of local recurrence and distant metastasis are high, and the survival time is much lower than that of early NPC patients [Citation3].
Long noncoding RNAs (lncRNAs) play a crucial part in the development and progression of cancer [Citation4]. The evolutionary conservatism, diversity and complexity of lncRNAs indicate that they have a vital role in regulating cell growth. Thus, it might be a gold mine to study lncRNAs for basic scientific research, biomarker and drug discovery, and potential therapies. Besides, lncRNAs regulate the development and progression of NPC [Citation5,Citation6]. Therefore, it is necessary to identify specific NPC-related lncRNA molecules and determine strategies for their clinical application.
It is worth noting that, as a tumor suppressor or oncogenic molecule, LncRNA RGMB antisense RNA 1 (RGMB-AS1) refers to the regulation of malignant tumors during the progression of many cancers [Citation7]. For example, lncRNA RGMB-AS1, as an oncogenic molecule, is highly expressed in human non-small cell lung cancer [Citation8]. LncRNA RGMB-AS1 also participates in the development of papillary thyroid carcinoma [Citation9]. Moreover, lncRNA RGMB-AS1 plays an anticancer role in hepatocellular carcinoma [Citation10]. However, the role of lncRNA RGMB-AS1 in NPC remains unknown. Importantly, we proved the binding mode of lncRNA RGMB-AS1 and Forkhead box A1 (FOXA1) through RIP experiment in our previous study, so we speculated that FOXA1 might be involved in the regulatory effect of lncRNA RGMB-AS1 on NPC development. Researches show that FOXA1 expression is decreased in NPC samples, and the up-regulation of FOXA1 in NPC cells inhibits the tumorigenicity in vivo [Citation11,Citation12]. The overexpression of FOXA1 is associated with a good prognosis in NPC patients. FOXA1 plays an important role in epithelial mesenchymal transformation (EMT) through key factors such as E-cadherin, and a positive correlation between E-cadherin and FOXA1 expression was observed in these studies [Citation13]. In summary, although these data suggest that FOXA1 regulates NPC development, further study are required to elucidate the mechanism of its effect on NPC development. Since lncRNA RGMB-AS1 could directly bind to FOXA1 in NPC, this study focuses on the new mechanism of lncRNA RGMB-AS1 on NPC biological function and EMT by regulating FOXA1.
Here, we aimed to evaluate the expression of lncRNA RGMB-AS1 in NPC and explore the new mechanisms of lncRNA RGMB-AS1/FOXA1 axis on biological function and EMT in NPC, to determine whether lncRNA RGMB-AS1 can be used as a gene therapy target for NPC in vitro and in vivo. As a result, we particularly emphasize the new mechanism of lncRNA RGMB-AS1 regulating FOXA1 on biological function and EMT of NPC. We found low expression of lncRNA RGMB-AS1 in NPC tissues and cells. The knockdown of lncRNA RGMB-AS1 promoted tumor proliferation and EMT, while the overexpression of lncRNA RGMB-AS1 had the opposite effect. Further studies showed that lncRNA RGMB-AS1 inhibited tumor proliferation and EMT by directly binding to FOXA1. Therefore, we identified a new potential therapeutic target for NPC treatment.
2 Materials and methods
2.1 Clinical samples
Thirty nasopharyngeal carcinoma patients with complete clinical data who received radical nasopharyngeal carcinoma resection were collected from the Department of General Surgery of the Affiliated Hospital of Guizhou Medical University between February 2019 and February 2020. The nasopharyngeal cancer tissue of patients was set as the experimental group, and the corresponding paracancerous tissue of nasopharyngeal cancer was set as the normal control group. The extracted tissue was immediately frozen in liquid nitrogen at −80°C. The clinical samples was approved by the local Institution Review Board of the Affiliated Hospital of Guizhou Medical University (approval No. ChiCTR-IPR-14005772). Patients and their families participated in the study voluntarily and signed the informed consent.
2.2 Cell cultures and cell transfection
Normal nasopharyngeal epithelial cell line (NP69) and 4 nasopharyngeal carcinoma cell lines (5–8 F, C666-1, CNE-2, and HNE2) were purchased from Wuhan Prosera Life Science and Technology Co., Ltd., while 1 nasopharyngeal carcinoma cell line (6–10B) was purchased from Beijing Beina Institute of Biotechnology. All cells were cultured in Dulbecco modified Eagle medium (DMEM) containing 10% fetal bovine serun (FBS), 100 U/mL penicillin, 100 μg/mL streptomycin. For selection of efficient lncRNA RGMB-AS1 siRNA, the Opti-MEM (Invitrogen, Grand Island, NY, USA) was used. The following siRNAs were transfected on the basis of the Lipofectamine 3000 protocol (Lipo3000, Invitrogen): Negative control of lncRNA RGMB-AS1: 5′-GCUUUACCAGCUCGCUAAUUU-3′; lncRNA RGMB-AS1-1#: 5′-GCUUGGCGUGAGCAAUGCAUU-3′; lncRNA RGMB-AS1-2#: 5′-GCAGCUCGUGGUUUGUACUUU-3′; Negative control of FOXA1: 5′-UUCUCCGAACGUGUCACGUTT-3′; FOXA1 siRNA-1#: 5′-GAGAGAAAAAAUCAACAGCTT-3′; FOXA1 siRNA-2#: 5′-CCGGUCAGCAACAUGAACUTT-3′. The cells were gathered after 72 h for mRNA quantification. In vitro studies used siRNA with the best inhibitory effect, and clone formation assay and nude mouse xenograft studies used the lncRNA RGMB-AS1 shRNA or FOXA1 shRNA lentivirus [Citation14].
2.3 Constructing lentivirus-mediated lncRNA RGMB-AS1/FOXA1 shRNA and lncRNA RGMB-AS1 pcDNA
The pLKO.1-shRNA lncRNA RGMB-AS1/FOXA1 and their control plasmids, as well as packaging plasmids including psPAX2 and PMD2G, were designed and cloned. The untransfected cells were eliminated with 2 µg/mL puromycin (Sigma-Aldrich, St-Louis, Missouri, USA) for 3 weeks to obtain stable cell lines [Citation15]. These lentivirus-based constructs were co-transfected to the cultured CNE-2 and 6–10B cells. 72 h later, the replication-deficient lentivirus particles were packaged and collected for in vivo experiment.
2.4 RT-qPCR
RNA was extracted from cells or tissues using Trizol reagent (Invitrogen, Carlsbad, CA, USA). Briefly, we synthesized cDNA through the Maxima first strand cDNA synthesis kit (Sangon Biotech, Shanghai, China). The ABI 7500 RT-PCR system (Applied Biosystems, Foster City, CA, USA) and the SYBR Premix Ex Taq kit (Biosteel Biotechnology, Shanghai, China) were used for RT-qPCR [Citation16,Citation17]. LncRNA RGMB-AS1 was normalized to GAPDH, while FOXA1, Vimentin and E-cadherin were normalized to β-actin. The primer sequences of RT-qPCR were shown in .
Table 1. The primers used in this study for RT-PCR
2.5 Nuclear/plasmic separation of cells
RNA Subcellular Isolation was used to isolate RNA from the cytoplasm or nucleus of nasopharyngeal cancer cells through the RNA Subcellular Isolation Kit (Active Motif, Carlsbad, CA, USA) on the basis of the corresponding instructions. In short, we collected CNE-2 and 6–10B cells and lysed them on ice for 5 minutes. The cells were then centrifuged at 12000 g for 3 minutes. Finally, the supernatant was gathered and the cytoplasmic RNA was measured, while nuclear RNA was extracted using nuclear precipitation [Citation18].
2.6 CCK-8
Cell viability was monitored using the Cell Counting Kit-8 assay [Citation19] (LaiFuSai Technology, Nanjing, Jiangsu, China). After transfection, CNE-2 and 6–10B cells were grown in 96-well plates. According to the corresponding instructions, we measure cell viability every 24 hours. Finally, the absorbance was read at 450 nm using Powerwave 340 (BioTek Instruments, Winooski, VT, USA).
2.7 Colony experiments
CNE-2 cells and 610-B cells from RPMI-1640 containing 10% FBS were spread into the 6-well plate and cultured in a constant temperature incubator at 5% CO2 and 37°C for 2 weeks. The medium needs to be replaced every three days. After 2 weeks, the colonies were fixed with methanol for 30 min and stained with 0.1% crystal violet (Sigma-Aldrich, USA) for 15 min. Colony counts were calculated using a light microscope (Olympus, Tokyo, Japan) [Citation20].
2.8 Transwell migration experiment
The migration ability of CNE-2 cells and 610-B cells was determined by Transwell [Citation19]. About 5 × 104 cells were inoculated into the upper chambers of transwell inserts in RPMI-1640 medium. The lower chamber was then covered with the medium containing 8% FBS. After incubation for 24 h, the cells were fixed with crystal violet. Subsequently, the cells were counted under a microscope.
2.9 Western blot
The logarithmic growth cells were collected, lysed with RIPA, and the total protein was extracted. Adjust the protein concentration to 4 μg/μL with BCA kit. The total proteins were then isolated by SDS-PAGE and transferred to PVDF membranes (EMD Millipore, Burlington, MA, USA). Finally, protein antibodies were used overnight at 4°C. Primary antibodies: E-cadherin (1:1000), Vimentin (1:1000), FOXA1 (1:1000) and β-actin (1:100,000) were purchased from Abcam (Cambridge, UK). After incubation with sheep anti-rabbit antibody for 1 h at room temperature, the membrane was treated with ECL luminescent solution. Protein expression was represented by gray value [Citation21].
2.10 RIP
Based on the experimental protocol, RNA immunoprecipitation was performed using the Thermo Fisher RIP kit (Thermo Fisher Scientific, Waltham, Massachusetts, USA). FOXA1 antibodies were purchased from Abcam (Cambridge, UK). We treated normal rabbit IgG (Abcam) as the negative control of the RIP procedure [Citation22]. After the target RNA was extracted and purified, the purified RNA was examined by RT-qPCR and Western blot analysis to verify the presence of its binding target.
2.11 Animal experiments
Eighteen BALB/c nude mice aged 4–6 weeks and weighing 20 ± 2 g were purchased from Animal Center of Guizhou Medical University. They were kept in a specefic Pathogen free (SPF) room in the school’s animal house. The experiment was approved by the school experimental Animal Ethics Committee. These nude mice were randomly divided into 3 groups: pcDNA-NC group, pcDNA-RGMB-AS1-1# group and pcDNA-RGMB-AS1-2# group. In the subcutaneous carcinogenic experiment, the right axillas of nude mice in each NC group were subcutaneously injected with stably transfected pcDNA-NC CNE-2 cells. The pcDNA-RGMB-AS1-1# or pcDNA-RGMB-AS1-2# group were respectively injected into the constructed pcDNA-RGMB-AS1-1# or pcDNA-RGMB-AS1-2# CNE-2 cells. Each group were injected with about 4 × 106 cells. 4 weeks after the successful establishment of the model, the nude mice were anesthetized by intraperitoneal injection of pentobarbital sodium (50 mg/kg). The animals were removed from the cervical vertebra and sacrificed. Tumor tissues were taken out for weighing and volume measurement [Citation23]. Later, some tumors were placed in liquid nitrogen quick-freezing and stored in a refrigerator at −80°C for future use. All animal experiment procedures had been approved by the Animal Care & Welfare Committee of GuiZhou Medical University (approval No. 2101395).
2.12 Immunohistochemical (IHC) staining
IHC was conducted using the two-step EnVision method [Citation24]. The determination was carried out in accordance with the manufacturer’s instructions. Anti-Ki-67 (Abcam; ab15580; 1:200), anti-Vimentin (Abcam; ab92547; 1:1000) and anti-E-Cadherin (Abcam; ab92547; 1:1000) antibody were used to stain the tissues, and then stained in the transplanted tumor tissues transfected with pcDNA-NC or pcDNA-RGMB-AS1. Finally, the optical density (OD) of the sections was measured.
2.13 Statistical analysis
Data results were obtained using SPSS 21.0 software (SPSS Inc., Chicago, IL, USA). All data were expressed as mean ± standard deviation (mean ± SD). The comparison between the two groups was performed by Student’s T test. One-way ANOVA test was used for comparison between multiple groups, LSD test was used for homogeneity of variance, and Tamhane’s T2 test was used for heterogeneity of variance. P < 0.05 was considered as significant difference between groups.
3 Results
3.1 LncRNA RGMB-AS1 and FOXA1 mRNA levels were down-regulated in NPC tissues and cells.
In order to further explore the roles of lncRNA RGMB-AS1 and FOXA1 in NPC development for elucidating the potential mechanism of lncRNA RGMB-AS1 on NPC, here, we firstly focused on the expressions of lncRNA RGMB-AS1, FOXA1 and EMT-related markers in nasopharyngeal carcinoma and their related clinical significance. We analyzed the mRNA expressions of lncRNA RGMB-AS1 and FOXA1 in nasopharyngeal carcinoma tissues and adjacent tissues of 30 patients with nasopharyngeal carcinoma who underwent radical NPC resection. The RT-qPCR results showed that the expression levels of lncRNA RGMB-AS1 and FOXA1 in nasopharyngeal carcinoma tissues were significantly lower than those in their paired paracancerous tissues ( and B). Besides, lncRNA RGMB-AS1 level was found to be positively correlated with FOXA1 level (). We treated NP69 as the normal control group and 5 nasopharyngeal carcinoma cell lines as the experimental group. By contrast, the levels of lncRNA RGMB-AS1 and FOXA1 in nasopharyngeal carcinoma cells were significantly lower than those in NP69 cells ( and E). As shown in , a distinct relationship was found between low lncRNA RGMB-AS1 expression and invasive potential of NPC, and lncRNA RGMB-AS1 expression in tumors was significantly correlated with the presence of Vimentin mutation and E-cadherin mutation. The data highlighted the role of lncRNA RGMB-AS1 as a potential biomarkers in NPC.
Table 2. Correlation between lncRNA RGMB-AS1 expression and clinicopathological factors
Figure 1. LncRNA RGMB-AS1 and FOXA1 mRNA levels were down-regulated in NPC tissues and cells. (a) Expression level of lncRNA RGMB-AS1 in nasopharyngeal carcinoma tissues and corresponding paracancerous tissues of 30 patients with nasopharyngeal carcinoma (n = 30, ***P < 0.001); (b) FOXA1 expression levels in nasopharyngeal carcinoma tissues and corresponding paracancerous tissues of 30 patients with nasopharyngeal carcinoma (n = 30, *P < 0.05); (c) RT-qPCR assay for the correlation between lncRNA RGMB-AS1 level and FOXA1 level; (d-e) The expression levels of lncRNA RGMB-AS1 and FOXA1 in human immortalized normal nasopharyngeal epithelial cell line (NP69) and 5 nasopharyngeal carcinoma cell lines (5–8 F, C666-1, CNE-2, HNE2 and 6–10B) (n = 3, *P < 0.05, **P < 0.01 vs NP69 group). Data were expressed as mean ± SD.
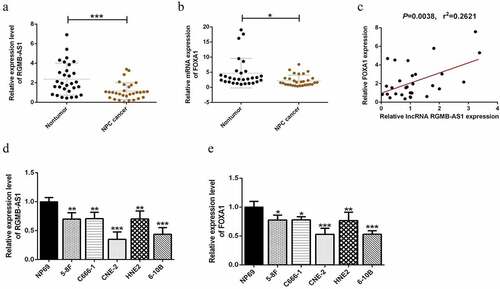
3.2 LncRNA RGMB-AS1 is closely related to the expression levels of E-cadherin and Vimentin in NPC tissues
In order to preliminarily explore whether the EMT process occurred in these NPC tissues, the expression levels of E-cadherin and Vimentin in NPC tissues and their paired paracancer tissues were detected by immunohistochemistry. The results showed that the expression of Vimentin in NPC tissues was significantly increased, while E-cadherin was significantly decreased (). Next, we explored the expression levels of E-cadherin and Vimentin in NPC tissues by RT-qPCR, and analyzed their correlation with the expression of lncRNA RGMB-AS1 respectively. We found that E-cadherin expression in NPC was significantly positively correlated with lncRNA RGMB-AS1 expression, while Vimentin expression was significantly negatively correlated with lncRNA RGMB-AS1 expression ( and E).
Figure 2. LncRNA RGMB-AS1 was closely related to the expression levels of E-cadherin and Vimentin in NPC tissues. (a-c) Expression of EMT-related factors (E-cadherin and Vimentin) in NPC and para-cancer tissues (n = 6, **P < 0.01, ***P < 0.001). (d) Correlation between lncRNA RGMB-AS1 expression level and Vimentin expression level in NPC tissues. (e) Correlation between lncRNA RGMB-AS1 expression level and E-cadherin expression level in NPC tissues.
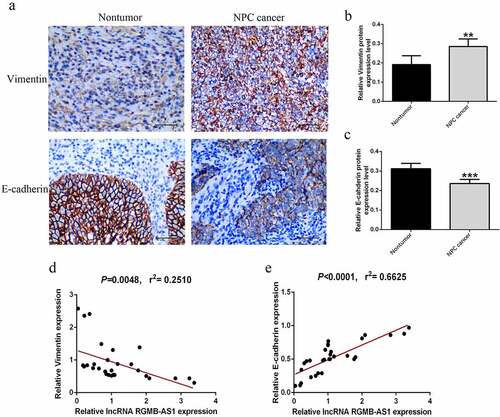
3.3 Effects of overexpression or knockdown of lncRNA RGMB-AS1 on cell proliferation and migration
To further investigate whether the up-regulation of lncRNA RGMB-AS1 expression was related to the inhibition of cell biological behavior in NPC, while the silencing of lncRNA RGMB-AS1 expression had the opposite effect, we silenced and overexpressed lncRNA RGMB-AS1, and preliminarily confirmed the possible mechanism of lncRNA RGMB-AS1 on NPC cell biological behavior. Firstly, the overexpression and silencing effect of lncRNA RGMB-AS1 in CNE-2 cells and 6–10B cells were detected by RT-qPCR. The results showed that the expression of lncRNA RGMB-AS1 was significantly decreased after transfection with siRNA-RGMB-AS1-1#/2#, while the expression of lncRNA RGMB-AS1 was significantly increased after transfection with pcDNA-RGMB-AS1 both in CNE-2 and 6–10B cells (). Then CCK-8 was used to detect the effect of lncRNA RGMB-AS1 on cell proliferation. The results showed that silencing lncRNA RGMB-AS1 expression significantly increased cell proliferation, while overexpressing lncRNA RGMB-AS1 significantly inhibited cell proliferation ( and F). In addition, lentiviral vector-mediated shRNA carrying stable silencing lncRNA RGMB-AS1 gene and vector-mediated pcDNA carrying stable overexpressing lncRNA RGMB-AS1 cell lines were established. Subsequently, clone formation experiment was used to assess the influence of lncRNA RGMB-AS1 on cell clone forming ability. The results showed that lowly expressed lncRNA RGMB-AS1 in CNE-2 and 6–10B cells promoted cell clone formation, while highly expressed lncRNA RGMB-AS1 inhibited cell clone formation ( and J).
Figure 3. Effects of overexpression or knockdown of lncRNA RGMB-AS1 on cell proliferation and migration. (a – d) Expression level of lncRNA RGMB-AS1 in CNE-2 cells (a and c) and 6–10B cells (b and d); (e-f) CCK-8 assay was used to detect the effect of lncRNA RGMB-AS1 on cell proliferation. (g-j) Clone formation assay was used to detect the effect of lncRNA RGMB-AS1 cell proliferation. (k-n) Transwell chamber assay was used to detect the effect of lncRNA RGMB-AS1 on cell migration. (mean ± SD, n = 3; *p < 0.05, **p < 0.01, ***p < 0.001 vs the corresponding negative control group).
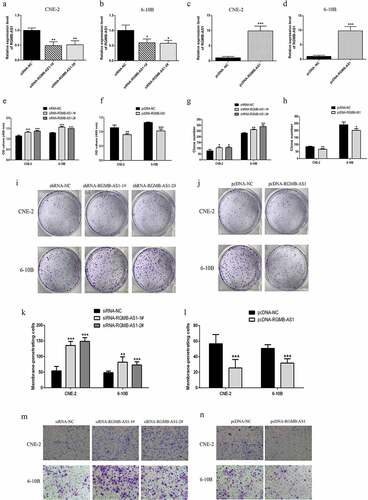
We evaluated the effect of lncRNA RGMB-AS1 on cell migration. Transwell invasion assay showed that the migration ability of cells in the siRNA-RGMB-AS1-1#/2# group was significantly higher than that in the siRNA-NC group. However, the migration ability of cells in the pcDNA-RGMB-AS1 group was significantly lower than that in the pcDNA-NC group ().
3.4 Effects of overexpression or knockdown of lncRNA RGMB-AS1 on cell EMT process
For investigating whether the change of lncRNA RGMB-AS1 level affected the EMT of NPC, we performed Western blot detection. The results showed that, in both of the lowly expressed lncRNA RGMB-AS1 groups, the Vimentin expression levels were significantly increased ( and E), while the expression level of E-cadherin was significantly decreased ( and E). Furthermore, in the highly expressed lncRNA RGMB-AS1 group, the expression level of Vimentin was significantly decreased ( and F), and the expression level of E-cadherin was significantly increased ( and F).
Figure 4. Effects of overexpression or knockdown of lncRNA RGMB-AS1 on cell EMT process. (a-b) The effect of lncRNA RGMB-AS1 silencing on Vimentin(a) and E-cadherin(b) expression; (c-d) Effect of lncRNA RGMB-AS1 overexpression on Vimentin(c) and E-cadherin(d) expression; (e-f) Images of lncRNA RGMB-AS1 silencing (e) or overexpression (f) regulated Vimentin and E-cadherin expression levels. (mean ± SD, n = 3; *p < 0.05, **p < 0.01, ***p < 0.001 vs the corresponding negative control group).
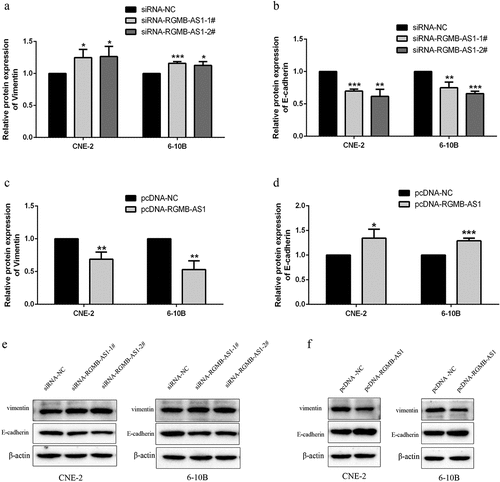
3.5 Effects of the acquisition or loss of lncRNA RGMB-AS1 function on FOXA1 expression
In order to further understand the epigenetic regulation potential mechanisms of lncRNA RGMB-AS1 on FOXA1 expression, RIP detection was used to confirm that lncRNA RGMB-AS1 could directly bind FOXA1 in NPC cells ( and B). Subsequent RT-qPCR was performed to detect the distribution of lncRNA RGMB-AS1 in the nucleus and cytoplasm. The results showed that lncRNA RGMB-AS1 existed in both cytoplasm and nucleus, but was mainly enriched in nucleus, accounting for about 2/3 of the total amount ( and D).
Figure 5. Effects of the acquisition or loss of lncRNA RGMB-AS1 function on FOXA1 expression. (a-b) Determination of the ability of FOXA1 to interact with lncRNA RGMB-AS1 via RIP assay. (c-d) Distribution of lncRNA RGMB-AS1 in the nucleus and cytoplasm of CNE-2 cells (c) and 6–10B cells (d); (e-f) Effects of silencing (e) or overexpression (f) of lncRNA RGMB-AS1 on FOXA1 mRNA expression; G-H. Effects of silencing (g) or overexpression (h) of lncRNA RGMB-AS1 on FOXA1 protein expression; (i-j) Images of silencing (i) or overexpression (j) of lncRNA RGMB-AS1 regulating FOXA1 expression level. (mean ± SD, n = 3; *p < 0.05, **p < 0.01, ***p < 0.001 vs the corresponding negative control group).
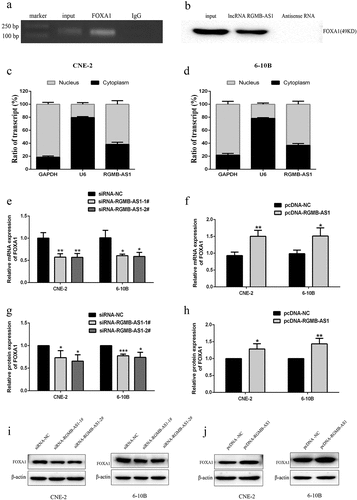
Next, we used RT-qPCR and Western blot to detect the effect of silencing or overexpressing lncRNA RGMB-AS1 on the expression level of FOXA1. The results showed that mRNA () and protein ( and I) expressions of FOXA1 were both significantly lower in cells transfected with the lowly expressed lncRNA RGMB-AS1 than those of cells transfected with siRNA-NC. Besides, the levels of FOXA1 mRNA () and protein ( and J) were significantly higher in cells transfected with the highly expressed lncRNA RGMB-AS1 than those of cells transfected with pcDNA-NC.
3.6 FOXA1 knockdown reversed the inhibition of lncRNA RGMB-AS1 on cell proliferation, migration and EMT process
Subsequently, the effects of lncRNA RGMB-AS1 and FOXA1 on cell proliferation and migration were assessed in vitro. Results indicated that after overexpressing lncRNA RGMB-AS1 in both CNE-2 and 6–10B cells, colony formation ( and B) and migration ( and D) were significantly reduced, while FOXA1 knockdown reversed its reduction effect. These results suggested that lncRNA RGMB-AS1 inhibited the proliferation and migration of NPC cells by promoting FOXA1.
Figure 6. FOXA1 knockdown reversed the inhibition of lncRNA RGMB-AS1 on cell proliferation, migration and EMT process. (a-b) The effect of lncRNA RGMB-AS1 on the clone formation ability of NPC cells by regulating FOXA1. (c-d) The effect of lncRNA RGMB-AS1 on the migration ability of NPC cells by regulating FOXA1. (e-g) The effect of lncRNA RGMB-AS1 on the expression levels of FOXA1 (e) Vimentin (f) and E-cadherin (g) by regulating FOXA1. (h-i) Image of lncRNA RGMB-AS1 regulating FOXA1 expression levels on FOXA1, Vimentin and E-cadherin proteins. (mean ± SD, n = 3; *P < 0.05, **P < 0.01, ***p < 0.001 vs the corresponding negative control group; ΔP<0.05, ΔΔP<0.01, ΔΔΔP<0.001 vs pcDNA-RGMB-AS1+ si-NC group).
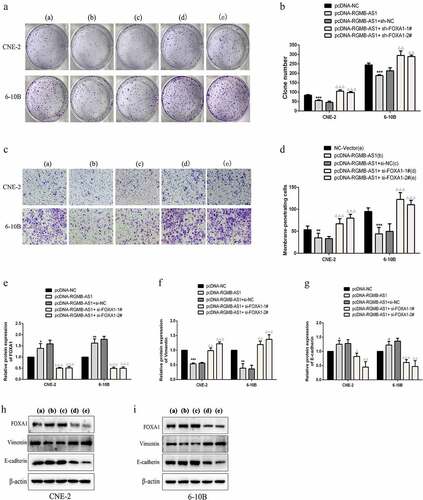
We determined whether the lncRNA RGMB-AS1/FOXA1 regulatory axis regulated EMT in NPC cells. Firstly, Western blotting were employed to examine FOXA1 expression and indicated that overexpression of lncRNA RGMB-AS1 promoted FOXA1 expression, whereas FOXA1 siRNA showed a partial reversal effect on the promoting effect of overexpression of lncRNA RGMB-AS1 (, H and I). Meanwhile, the expressions of EMT markers were determined. The results showed that the overexpression of lncRNA RGMB-AS1 decreased expression of Vimentin and increased E-cadherin expression in NPC cells, while the inhibition of FOXA1 partially offset this effect. (). These results indicated that the lncRNA RGMB-AS1/FOXA1 regulatory axis inhibited the EMT of NPC cells.
3.7 The effect of lncRNA RGMB-AS1 on xenograft growth in NPC nude mice
In order to further investigate the effect of lncRNA RGMB-AS1 overexpression on NPC growth in vivo, the CNE-2 cell line with stable lncRNA RGMB-AS1 overexpression was constructed using pcDNA-RGMB-AS1, and then subcutaneously injected into the right axilla of nude mice to establish the CNE-2 xenograft tumor model in nude mice. The results showed that compared to the control treatment, the tumor volume and the tumor weight in the pcDNA-RGMB-AS1-1# and 2# group were significantly reduced. It suggested that the growth rate of nasopharyngeal carcinoma CNE-2 cell lines after overexpression of lncRNA RGMB-AS1 was lower than that of the negative control group in vivo (). In addition, the expressions of Ki-67 were decreased in nude mice transplanted tumor after overexpression of lncRNA RGMB-AS1 ( and E). These results suggested that up-regulation of lncRNA RGMB-AS1 led to reduced NPC growth.
Figure 7. The effect of lncRNA RGMB-AS1 on FOXA1 xenograft growth in NPC nude mice. (a) Morphology of NPC transplanted tumor in nude mice; (b) The size of NPC transplanted tumor in nude mice; (c) Expression level of lncRNA RGMB-AS1 in NPC xenograft of nude mice; (d) The expression level of FOXA1 in NPC xenograft of nude mice; (e) The expression level of Ki-67 protein in NPC transplanted tumor tissue; (f) Immunohistochemical image of Ki-67 protein expression. (mean ± SD, n = 6; *P < 0.05, **P < 0.01).
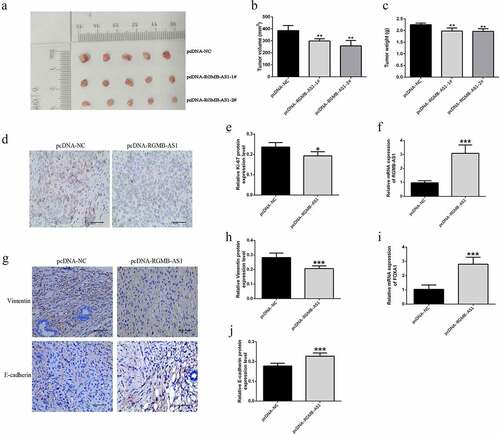
Importantly, the expressions of lncRNA RGMB-AS1 and FOXA1 were all significantly increased in nasopharyngeal carcinoma xenograft mice with overexpression of lncRNA RGMB-AS1 (). Vimentin expression level in pcDNA-RGMB-AS1 group was significantly lower than that in pcDNA-NC group ( and H), while E-cadherin expression level in pcDNA-RGMB-AS1 group was significantly higher than that in pcDNA-NC group ( and J). These results were consistent with our results in vitro.
4 Discussion
In recent years, radiotherapy technology has been further developed, but the 5-year survival rate of NPC patients has not improved very well. Therefore, how to improve the therapeutic effect of NPC is an urgent problem to be solved at present, and efforts should be made in many aspects, such as early diagnosis and finding new therapeutic targets. A great deal of studies have shown that there are some changes of lncRNA expressions in NPC, and some of the identified lncRNAs have important implications for regulating the occurrence of NPC [Citation25]. As described in a prior study, lncRNA RGMB-AS1 is involved in the regulation of malignant tumors during the progression of many cancers [Citation7]. However, the role of lncRNA RGMB-AS1 in NPC remains largely unknown. Therefore, this study was mainly to explore the potential mechanism of lncRNA RGMB-AS1 affecting the biological behavior of NPC cells. The results of this study showed that lncRNA RGMB-AS1 acted as an up-regulated FOXA1 sponge, inhibiting malignant biological behavior and EMT process of NPC cells. Our findings provided a new theoretical basis for clinical application.
NPC has the biological characteristics of high invasiveness and high metastasis [Citation26]. Therefore, the control of metastasis is a key factor to improve the prognosis and prolong the survival time of tumor patients. Studies had shown that lncRNAs regulated the occurrence and development of different types of cancer, including NPC [Citation27,Citation28]. It had been found that lncRNA RGMB-AS1 was also involved in the proliferation, migration and other malignant biological behaviors of various tumors [Citation10,Citation29]. Here, we emphasized the role of lncRNA RGMB-AS1 in NPC. We confirmed that the expression of lncRNA RGBM-AS1 in NPC tissues was significantly lower than that in paracancerous tissues, and this result was also confirmed in 5 cell lines. Then, we provided evidence that overexpression of lncRNA RGMB-AS1 significantly inhibited the proliferation and migration of NPC cells, while knockdown of lncRNA RGMB-AS1 showed the opposite effect. In recent years, some lncRNAs had been proved to affect the occurrence and development of cancer through regulatory mechanism in NPC. For example, lncRNA MSC-AS1 promoted the proliferation of hepatoma carcinoma cells by maintaining PFKFB3 expression [Citation30]. lncRNA RBM24 inhibited NPC development by targeting MALAT1 [Citation31]. These studies suggested that it was of great significance to identify the regulatory mechanisms of specific lncRNAs in NPC for exploring new therapeutic targets. Here, we investigated the subcellular localization of lncRNA RGMB-AS1 in NPC. As is known to all, specific roles of lncRNAs are different when they are in different subcellular localization. Therefore, the function of lncRNAs are related to their unique subcellular localization. For example, lncRNAs in the cytoplasm were mainly involved in gene regulation at the post-transcriptional and translational levels, including interacting with the cytoplasm proteins [Citation32], binding with target mRNA to inhibit their translation [Citation33], and acting as endogenous competing RNAs (ceRNAs) that interact with microRNAs [Citation34]. LncRNAs located in the nucleus were mainly involved in gene regulation at epigenetic and transcriptional levels, including protein regulation in the cell nucleus [Citation35]. We found that lncRNA RGMB-AS1 existed in both cytoplasm and nucleus through plasmic nucleus separation experiments, suggesting that lncRNA RGMB-AS1 may be involved in the malignant biological behavior of NPC through two different pathways: cytoplasm and nucleus. LncRNA RGMB-AS1 mainly distributed in the nucleus, which further suggested that lncRNA RGMB-AS1 inhibited the process of NPC mainly through protein interaction in the nucleus. Subsequently, we confirmed the binding relationship between lncRNA RGMB-AS1 and FOXA1, suggesting a regulatory relationship between them. In this study, further experiments confirmed that lncRNA RGMB-AS1 inhibited the proliferation, migration and EMT of NPC cells by positively regulating FOXA1 gene expression. The same results were confirmed by our in vivo tumor transplant experiment.
In-depth study of lncRNA on EMT process in cancer is of great significance for investigating cell biological behavior [Citation36,Citation37]. EMT is a process in which epithelial cells are transformed into mesenchymal cells by characteristic loss, with decreased expression of epithelial markers (such as E-cadherin) and increased expression of mesenchymal markers (such as Vimentin) [Citation38]. EMT gave the ability of cancer cells to migrate and invade [Citation39], and a large number of lncRNAs were involved in the progress of EMT in NPC [Citation6,Citation40]. E-cadherin acted as a negative regulator of EMT, while Vimentin acted as its positive regulator. Here, FOXA1 knockdown reversed the increased expression of the EMT marker E-cadherin and decreased expression of Vimentin in NPC cells induced by overexpression of lncRNA RGMB-AS1. FOXA1 had also been reported to play a role in a variety of cancers [Citation41,Citation42]. Previous studies have reported that FOXA1 was highly expressed in the normal nasopharyngeal epithelium, but was reduced in NPC samples [Citation11,Citation12]. Restoration of FOXA1 in NPC cells inhibited cell proliferation and invasion in vitro and tumorigenicity in vivo [Citation43,Citation44]. In addition, FOXA1 played an important role in the EMT process of NPC through key factors such as E-cadherin. A positive correlation between E-cadherin and FOXA1 expression was observed in these studies [Citation13]. Several other studies showed that FOXA1 promoted EMT mainly by regulating E-cadherin expression [Citation45,Citation46]. Similar results were fully demonstrated in our study.
These findings suggest that FOXA1 was involved in the inhibition of the proliferation, migration and EMT of lncRNA RGMB-AS1 in NPC cells. Further experiments on xenografts in nude mice showed that lncRNA RGMB-AS1 inhibited tumor growth, which was consistent with in vitro results. Overexpression of lncRNA RGMB-AS1 restricted the growth of CNE-2 xenografts in nude mice and promoted FOXA1 expression. All the above data indicated that lncRNA RGMB-AS1 inhibited the progression of NPC by regulating FOXA1. The results of this study were helpful to further understand the mechanism of NPC metastasis, and provided a new theoretical basis for the prevention and treatment of NPC metastasis, which was expected to be better applied in clinical applications. However, in the near future, more studies are needed to further clarify the true role and function of lncRNA RGMB-AS1 as a prognostic marker in NPC cancer biology, and it may become a necessary condition for the development of new therapies for NPC.
5 Conclusions
In this study, we reported that a novel lncRNA RGMB-AS1 was down-regulated in NPC. In addition to inhibiting cell viability, proliferation and migration, the overexpression of lncRNA RGMB-AS1 in NPC cells significantly inhibited the EMT process. In addition, the tumorigenic ability of lncRNA RGMB-AS1 was also reduced in vivo. We further demonstrated that the tumor suppressive effect of lncRNA RGMB-AS1 can be mediated through direct binding with FOXA1, which added a new perspective to the role of inhibition of NPC occurrence, and thus provided a potential target for the diagnosis, treatment and prognosis of NPC.
Research highlights
1. The down-regulation of LncRNA RGMB-AS1 expression may be one of the important factors affecting the malignant biological behavior of NPC.
2. LncRNA RGMB-AS1 inhibits NPC proliferation, migration, epithelial mesenchymal transformation and tumorigenesis by regulating FOXA1 in vivo and in vitro.
3. LncRNA RGMB-AS1 may be an important indicator of clinical prognosis.
Abbreviations
Long non-coding RNA: lncRNA; RGMB antisense RNA 1: RGMB-AS1; Nasopharyngeal carcinoma: NPC; Forkhead box A1: FOXA1; EMT: epithelial mesenchymal transformation; Quantificational real-time polymerase chain reaction: RT-qPCR; Complementary deoxyribonucleic acid: cDNA; Small interfering RNA: siRNA; RNA immunoprecipitation: RIP.
Authors’ contributions
TZ and YJ performed the experiments, interpreted the results and contributed to manuscript writing. TZ and XL conceived and designed the current study and contributed to the writing of the manuscript. All authors read and approved the final manuscript. TZ, YJ and XL confirm the authenticity of all raw data.
Availability of Data and Materials
The datasets used or/and analyzed during the current study are available from the corresponding author on reasonable request.
Consent for publication
Not applicable.
Declarations
Ethics committee approval was received for this study from the Ethical Committee of GuiZhou Medical University. The reference number includes number of permit SYXK (黔) 2018-001 and ethical number of animal qualitative qualification 2101395. This clinical trial study has been approved by the Ethics Committee of the Affiliated Hospital of Guizhou Medical University and registered in China Clinical Trial Center (ChiCTR-IPR-14005772). All patients signed informed consent before treatment.
Supplemental Material
Download Zip (133.7 KB)Acknowledgements
None.
Disclosure statement
No potential conflict of interest was reported by the author(s).
Supplementary material
Supplemental data for this article can be accessed here
Additional information
Funding
References
- Chen Y, Chan A, Le Q, et al. Nasopharyngeal carcinoma. Lancet. 2019;394(10192):64–80.
- Agulnik M, Epstein J. Nasopharyngeal carcinoma: current management, future directions and dental implications. Oral Oncol. 2008;44(7):617–627.
- Korbi AE, Tkhayat SB, Bouatay R, et al. Therapeutic outcomes of nasopharyngeal carcinomas: a single-center study conducted at the fattouma bourguiba university hospital in Monastir, Tunisia. Pan Afr Med J. 2021;38:143.
- Nagano T, Fraser P. No-nonsense functions for long noncoding RNAs. Cell. 2011;145(2):178–181.
- Li J, Li D, Zhang X, et al. Long noncoding RNA SLC9A3‑AS1 increases E2F6 expression by sponging microRNA‑486‑5p and thus facilitates the oncogenesis of nasopharyngeal carcinoma. Oncol Rep. 2021;46(2). DOI:10.3892/or.2021.8116
- Du M, Hu X, Jiang X, et al. LncRNA EPB41L4A-AS2 represses nasopharyngeal carcinoma metastasis by binding to YBX1 in the nucleus and sponging MiR-107 in the cytoplasm. Int J Biol Sci. 2021;17(8):1963–1978.
- Xu Z, Xi K. LncRNA RGMB-AS1 promotes laryngeal squamous cell carcinoma cells progression via sponging miR-22/NLRP3 axis. Biomed Pharmacother. 2019;118:109222.
- Li P, Li J, and Yang R, et al. Study on expression of lncRNA RGMB-AS1 and repulsive guidance molecule b in non-small cell lung cancer. Diagn Pathol. 2015;10(63): 1–7.
- Zhang Z, Li SY, Zhang LB. LncRNA RGMB-AS1 is activated by E2F1 and promotes cell proliferation and invasion in papillary thyroid carcinoma. Eur Rev Med Pharmacol Sci. 2018;22(7):1979–1986.
- Sheng N, Li Y, Qian R, et al. The clinical significance and biological function of lncRNA RGMB-AS1 in hepatocellular carcinoma. Biomed Pharmacother. 2018;98:577–584.
- Wang W, Yi M, Chen S, et al. Significance of the NOR1-FOXA1/HDAC2-Slug regulatory network in epithelial-mesenchymal transition of tumor cell. Oncotarget. 2016;7(13):16745–16759.
- Yi M, Yang J, Li W, et al. The NOR1/OSCP1 proteins in cancer: from epigenetic silencing to functional characterization of a novel tumor suppressor. J Cancer. 2017;8(4):626–635.
- Ammous-Boukhris N, Ayadi W, Derbel M, et al. FOXA1 expression in nasopharyngeal carcinoma: association with clinicopathological characteristics and EMT markers. BioMed Research International. 2020;2020:4234632.
- Chen M, Zhuang C, Liu Y, et al. Tetracycline-inducible shRNA targeting antisense long non-coding RNA HIF1A-AS2 represses the malignant phenotypes of bladder cancer. Cancer Lett. 2016;376(1):155–164.
- Ye Y, Peng Y, Li Y, et al. Effect of lincRNA-p21 targeting HIF-1α on biological functions of liver cancer cells. Oncol Lett. 2019;17(6):4964–4968.
- Dhamija S, Menon MB. Measuring lncRNA expression by real-time PCR. Methods Mol Biol. 2021;2348:93–111.
- Ren XY, Yang WB, Tian Y. Overexpression of long noncoding RNA PTPRG-AS1 is associated with poor prognosis in epithelial ovarian cancer. Rev Assoc Med Bras (1992). 2020;66(7):948–953.
- Ye M, Lu H, Tang W, et al. Downregulation of MEG3 promotes neuroblastoma development through FOXO1-mediated autophagy and mTOR-mediated epithelial-mesenchymal transition. Int J Biol Sci. 2020;16(15):3050–3061.
- Wang X, Nie P, Zhu D. LncRNA HOXA10-AS activated by E2F1 facilitates proliferation and migration of nasopharyngeal carcinoma cells through sponging miR-582-3p to upregulate RAB31. Am J Rhinol Allergy. 2022;19458924211064400. DOI:10.1177/19458924211064400
- Han R, Chen S, Wang J, et al. LncRNA UCA1 affects epithelial-mesenchymal transition, invasion, migration and apoptosis of nasopharyngeal carcinoma cells. Cell Cycle. 2019;18(21):3044–3053.
- Zhou C, Cao H, Meng X, et al. Lnc-MEG3 inhibits invasion, migration, and epithelial- mesenchymal transition of nasopharyngeal carcinoma cells by regulating sequestosome 1. Head Neck. 2022;44(1):201–211.
- Wang J, Cai X, Zhang L, et al. Linc01513 inhibits the malignant potential of nasopharyngeal carcinoma by binding to PTBP1. J Cancer. 2021;12(24):7380–7389.
- Tang J, Fu C, Li Y. Long noncoding RNA OIP5-AS1 promotes the disease progression in nasopharyngeal carcinoma by targeting miR-203. BioMed Res Int. 2021;2021:9850928.
- Zhang MY, Zhang ZL, Cui HX, et al. Long non-coding RNA FENDRR inhibits NSCLC cell growth and aggressiveness by sponging miR-761. Eur Rev Med Pharmacol Sci. 2018;22(23):8324–8332.
- Wu Q, Zhao Y, Shi R, et al. LncRNA SNHG16 facilitates nasopharyngeal carcinoma progression by acting as ceRNA to sponge miR-520a-3p and upregulate MAPK1 expression. Cancer Manag Res. 2021;13:4103–4114.
- Lin M, Zhang XL, You R, et al. Neoantigen landscape in metastatic nasopharyngeal carcinoma. Theranostics. 2021;11(13):6427–6444.
- Li X, Lin Y, Yang X, et al. Long noncoding RNA H19 regulates EZH2 expression by interacting with miR-630 and promotes cell invasion in nasopharyngeal carcinoma. Biochem Biophys Res Commun. 2016;473(4):913–919.
- Jin C, Yan B, Lu Q, et al. The role of MALAT1/miR-1/slug axis on radioresistance in nasopharyngeal carcinoma. Tumour Biol. 2016;37(3):4025–4033.
- Pan B, Zhao M, Wang N, et al. LncRNA RGMB-AS1 promotes glioma growth and invasion through miR-1200/HOXB2 axis. Onco Targets Ther. 2019;12:10107–10114.
- Jin X, Qiao L, Fan H, et al. Long non-coding RNA MSC-AS1 facilitates the proliferation and glycolysis of gastric cancer cells by regulating PFKFB3 expression. Int J Med Sci. 2021;18(2):546–554.
- Hua WF, Zhong Q, Xia TL, et al. RBM24 suppresses cancer progression by upregulating miR-25 to target MALAT1 in nasopharyngeal carcinoma. Cell Death Dis. 2016;7(9):e2352.
- Perez CAG, Adachi S, Nong QD. Sense-overlapping lncRNA as a decoy of translational repressor protein for dimorphic gene expression. PLoS Genetics. 2021;17(7):e1009683.
- Wei J, Dou Q, Ba F, et al. Identification of lncRNA and mRNA expression profiles in dorsal root ganglion in rats with cancer-induced bone pain. Biochem Biophys Res Commun. 2021;572:98–104.
- Lin X, Zhuang S, and Chen X, et al. LncRNA ITGB8-AS1 functions as a ceRNA to promote colorectal cancer growth and migration through integrin-mediated focal adhesion signaling. Mol Ther. 2022;30(2):688–702 .
- Li M, Gou H, Tripathi BK, et al. An apela RNA-containing negative feedback loop regulates p53-mediated apoptosis in embryonic stem cells. Cell Stem Cell. 2015;16(6):669–683.
- Güneş CE, Güçlü E, Vural H, et al. Knockdown of lncRNA ZEB2NAT suppresses epithelial mesenchymal transition, metastasis and proliferation in breast cancer cells. Gene. 2021;805:145904.
- Zhang C, J E, and Yu E. LncRNA CASC21 induces HGH1 to mediate colorectal cancer cell proliferation, migration, EMT and stemness. RNA Biol. 2021;18(sup1):369–381.
- Expósito-Villén A, Aránega A E, Franco D. Functional role of non-coding RNAs during epithelial-to-mesenchymal transition. Noncoding RNA. 2018;4(2):14.
- Tsai JH, Yang J. Epithelial-mesenchymal plasticity in carcinoma metastasis. Genes Dev. 2013;27(20):2192–2206.
- Ji Y, Wang M, Li X. The long noncoding RNA NEAT1 targets miR-34a-5p and drives nasopharyngeal carcinoma progression via Wnt/β-catenin signaling. Yonsei Med J. 2019;60(4):336–345.
- Adams EJ, Karthaus WR, Hoover E, et al. FOXA1 mutations alter pioneering activity, differentiation and prostate cancer phenotypes. Nature. 2019;571(7765):408–412.
- Barbieri CE, Baca SC, Lawrence MS, et al. Exome sequencing identifies recurrent SPOP, FOXA1 and MED12 mutations in prostate cancer. Nat Genet. 2012;44(6):685–689.
- Li J, Wang W, Chen S, et al. FOXA1 reprograms the TGF-β-stimulated transcriptional program from a metastasis promoter to a tumor suppressor in nasopharyngeal carcinoma. Cancer Lett. 2019;442:1–14.
- Peng Q, Zhang L, Li J, et al. FOXA1 suppresses the growth, migration, and invasion of nasopharyngeal carcinoma cells through repressing miR-100-5p and miR-125b-5p. J Cancer. 2020;11(9):2485–2495.
- Wang H, Meyer CA, Fei T, et al. A systematic approach identifies FOXA1 as a key factor in the loss of epithelial traits during the epithelial-to-mesenchymal transition in lung cancer. BMC Genomics. 2013;14:680.
- Anzai E, Hirata K, Shibazaki M, et al. FOXA1 induces e-cadherin expression at the protein level via suppression of slug in epithelial breast cancer cells. Biol Pharm Bull. 2017;40(9):1483–1489.