ABSTRACT
Microvascular dysfunction causes mortality in the presence of sepsis and multi-organ failure. Previous studies have demonstrated that exogenous administration of exosomes from adipose-derived mesenchymal stem cells (ADSCs) protects against sepsis, improves organ function, decreases vascular leakage and increases survival. However, the underlying regulatory mechanism was largely unknown. Therefore, in this study, a mouse sepsis model based on cecal ligation and puncture (CLP) was constructed. Exosomes from various ADSCs were intravenously administered at 4 h post CLP. Treatment with ADSC exosomes (Exo), particularly those with hypoxic pretreatment (HExo), enhanced survival, suppressed renal vascular leakage and decreased kidney dysfunction in septic mice. HExo ameliorated sepsis-induced increases in chemokine and cytokine plasma levels. Furthermore, the HExo circRNA content, determined through next-generation sequencing, revealed abundant mmu_circ_0001295. Further studies demonstrated that downregulation of exosomal mmu_circ_0001295 suppressed the exosomes’ protective effects against sepsis. HExo prevented microvascular dysfunction, thus potentially improving sepsis outcomes via mmu_circ_0001295 delivery. In summary, the data indicated that HExo elongate sepsis-induced renal injury through delivering mmu_circ_0001295.
Graphical abstract
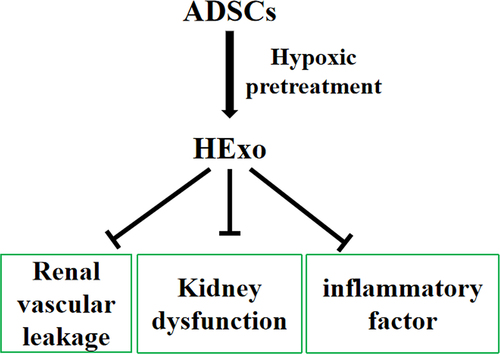
Introduction
Sepsis is a major underlying reason for acute kidney injury (AKI) among seriously ill patients [Citation1], accounting for 40–50% of the AKI [Citation2]. AKI development in patients with sepsis increases in-hospital death risk 6- to 8-fold [Citation3]. Among survivors, the risk of chronic kidney disease progression is high [Citation4]. The mechanisms through which sepsis leads to AKI are not fully known, thus leading to nonspecific treatments. In some cases, AKI may occur without overt hypoperfusion signs, thus suggesting the existence of other underlying mechanisms.
ADSCs are a family of pluripotent mesenchymal stem cells (MSCs) that are often used in clinical stem cell therapy [Citation5,Citation6]. MSCs decrease organ damage through homing to injured tissue [Citation7] and secreting paracrine signals in sepsis [Citation8]. In addition, MSC-derived exosomes, 30–120 nm microvesicles containing various macromolecules, are important paracrine factors that with essential functions during the clinic treatment [Citation9–11]. ADSCs, compared with bone marrow-derived MSCs, can be collected in greater abundance more easily, through minimally invasive approaches [Citation12]. Thus, ADSC exosomes (Exo) and ADSCs have potential clinical applications. Previous studies have shown that exosomes from hypoxia-treated, compared with untreated, ADSCs have greater therapeutic effects in enhancing angiogenesis [Citation13]. Nevertheless, the detailed mechanisms underlying ADSC therapy for sepsis are unknown.
Exosomes belong to a class of secreted vesicles, which contain various biologically active molecules including proteins, mRNAs and non-coding circularized RNAs (circRNAs) [Citation14]. The circRNAs are single-stranded RNA molecules produced by non-canonical pre-messenger RNA splicing. The downstream donor sites are ‘back-spliced’ to upstream acceptor sites. For instance, the end of exon 4 covalently binds the exon 4 start [Citation15]. Generally, circRNA abundance is lower than that of its corresponding linear mRNA [Citation16–18]; thus, circRNA formation might not strongly influence cellular physiology [Citation19]. The circRNA functions are largely unclear. Therefore, the current study aimed to clarify the Exo regulatory mechanisms in sepsis.
Materials and methods
Exo isolation and identification
Exo were isolated as previously described [Citation20]. ADSCs were cultivated in FBS-free endothelial cell growth medium-2 MV supplemented with 1× serum replacement solution (PeproTech, Rocky Hill, USA) for 2 d. After the cells reached 80–90% confluence, they were rinsed with phosphate-buffered saline (PBS). The conditioned culture medium was removed, and the cells were centrifuged at 300 × g for 10 min and then 2,000 × g for another 10 min to eliminate apoptotic cells and cellular debris. After centrifugation at 10,000 × g for 0.5 h, the supernatant was passed through a 0.22 μm filter (Millipore, Billerica, MA, USA), and 15 mL was transferred to Amicon Ultra-15 Centrifugal Filter Units (100 kDa; Millipore), which were centrifuged at 4,000 × g to concentrate samples to ~1 mL. The ultrafiltration units were washed twice with PBS, and the supernatant was filtered at 100,000 × g. Exosome pellets were resuspended in 500 μL PBS, and subsequent processes were performed at 4°C. Exosome protein concentrations were determined with a Pierce BCA Protein Assay Kit (Thermo Fisher Scientific, Waltham, MA, USA). Exosomes were maintained at −80°C until later analysis via Western blotting together with transmission electron microscopy. The total exosome number and size distribution were analyzed in the NTA software (ZetaView 8.04.02).
For mmu_circ_0001295 silencing analysis, small interfering (si) RNAs against mmu_circ_000129 [si-mmu_circ_000129 (Shanghai GenePharma Co., Ltd.)] were used for ADSC transfection with Lipofectamine 2000 (Invitrogen; Thermo Fisher Scientific, Inc.) before Exo isolation.
Sepsis model and treatment
The animal experiment was approved by the Ethics Committee of Shanghai Pudong Hospital. C57BL/6 N mice (6 weeks of age) were housed in a germ-free environment, according to the Guide for Care and Use of Laboratory Animals released by the NIH. The CLP was performed according to a published study [Citation21]. The cecum was ligated at the colon juncture with a 5–0 silk ligature suture to maintain intestinal continuity, then was punctured twice with 22 G needle. The animals were subcutaneously fluid-resuscitated with saline. The sham group was operated upon in the same manner as that with CLP except for cecum ligation and puncture.
To investigate survival, we randomly divided the mice randomly into four groups: sham, CLP-Exo, CLP-PBS, CLP-HExo and CLP-si-circ-HExo. Four-hour post CLP surgery, the mice were intravenously injected with PBS and exosomes (2 mg/kg body weight). The dose was based on prior data [Citation22]. Mice were treated with imipenem (25 mg/kg, subcutaneously) at 6 h, 1 d and 2 d after CLP. Survival rates were monitored for 7 d.
Kidney vascular leakage detection
Vascular leakage was quantified via Evans blue dye assays in kidney tissue [Citation23]. A 1% Evans blue dye solution (Sigma-Aldrich, St. Louis, USA) was administered in saline by tail vein injection. After 40 min, we sacrificed mice and perfused their kidney tissues, which were then collected. Kidney tissues were weighed and placed in 1 mL of formamide (Avantor, Center Valley, PA, USA) at 60°C for 1 d in preparation for Evans blue dye analysis. The samples were centrifuged at 2,000 rpm for 10 min, and the supernatant was collected. Evans blue dye concentrations in the supernatant were determined according to the absorbance at 620 nm, detected with a plate reader, and computed on the basis of a standard curve.
Cytokine/chemokine and organ function measurement
The whole blood from mice was collected in every group 1 d after surgery, then transferred to a tube containing EDTA (BD Vacutainer, Franklin Lakes, USA). Plasma was separated through 1800 rpm centrifugation for 5 min, then stored at −80°C until subsequent use. ALT, BUN and AST plasma levels, used as indicators of kidney and liver function, were detected with ELISA kits (BioAssay Systems, Atlanta, USA).
The inflammatory factors IL-6, IL-1β, TNF-α and MCP-1 in the plasma were analyzed through mouse pro-inflammatory chemokine and cytokine focused 10-plex arrays from Eve Technologies (Calgary, AB, Canada).
Strand-specific RNA-Seq library construction
Total RNA was obtained from hypoxia pretreated ADSC exosomes (HExo) and Exo by using TRIzol reagent (Invitrogen, Carlsbad, CA, USA). Approximately ~3 μg total RNA from every sample was processed with VAHTS Total RNA-seq (H/M/R) Library Prep kits from Illumina (Vazyme Biotech Co., Ltd, Nanjing, China) to remove ribosomal RNA and retain RNAs including mRNAs and noncoding RNAs. The RNA was treated with 40 U RNase R (Epicenter) at 37°C for 3 h, and TRIzol purification was performed. An RNA-seq library was constructed with KAPA Stranded RNA-Seq Library Prep kits (Roche, Basel, Switzerland) and used for NGS (Illumina HiSeq 4000, Aksomics, Inc., Shanghai, China).
Quantitative real-time polymerase chain reaction (RT-qPCR)
TRIzol reagent (Invitrogen, Carlsbad, CA, USA) was used to obtain total RNA from Exo and kidney tissue. RNA purity and concentration were determined with a Nano-Drop 2000 spectrophotometer (Thermo Fisher Scientific, USA). A PrimeScript™ RT reagent Kit (Takara, Japan) was used to synthesize cDNA. The SYBR Green Real-time PCR Master Mix (Toyobo, Japan) was applied to examine mmu_circ_0001295 expression, which was normalized to that of β-actin. The relative mmu_circ_0001295 expression was calculated with the 2− ΔΔCt approach. The primers for mmu_circ_0001295 were mmu_circ_0001295: forward, 5ʹ-CGGCGTAGTCCAGACCATC-3ʹ; reverse, 5ʹ- CAGGTAGATCAGGTACACTGTG-3ʹ; β-actin: forward, 5ʹ-CATGTACGTTGCTATCCAGGC-3ʹ; reverse, 5ʹ-CTCCTTAATGTCACGCACGAT-3ʹ.
Statistical analyses
Data are shown as means ± standard deviation (SD). Statistical analyses were performed in GraphPad Prism (La Jolla, USA) to determine significant differences among groups. P-values ≤0.05 were regarded as statistically significant. Two-tailed Student’s t-test was used to determine significant differences between the two groups, and one-way ANOVA with post hoc Bonferroni test was used to determine significant differences among three or more groups.
Results
Exo treatment decreases CLP-induced mortality in sepsis
ADSCs isolated from adipose tissue were cultured as previously described [Citation20]. Exo from ADSCs and hypoxia pretreated ADSCs were isolated and assayed through nanoparticle tracking analysis. The average exosome size was approximately 80 nm, and >90% of the exosomes were within the 50–100 nm range (), in agreement with the previously reported data [Citation24]. Transmission electron micrography was used to verify that the Exo had cup-shaped or spherical morphology, with diameters of ~100 nm (). Western blotting indicated that the Exo were positive for exosome marker protein expressions, such as CD81 and CD63, which are cellular components ().
Figure 1. Effects of ADSC exosomes on CLP-induced mortality. (a) The number of particles versus particle size, determined by nanoparticle tracking analysis with ZetaView. (b) Transmission electron micrographs showing ADSC exosome (Exo) or hypoxically pretreated ADSC exosome (HExo) morphology. (c) Western blots of CD63 and CD81 expression in Exo and HExo. (d) Mice were subjected to CLP and treated with HExo or Exo (2 mg protein/kg body weight) or PBS. Survival rates were monitored for a total of 168 h (7 days). **p < 0.01 vs. CLP-PBS. #p < 0.05 vs CLP-Exo. N = 12/group.
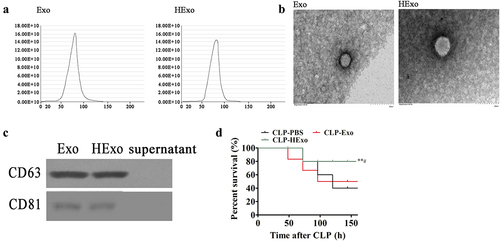
C57BL/6 N mice, as used in our prior work, are well suited to the investigation of CLP-induced sepsis [Citation25]. To confirm whether Exo had therapeutic effects in sepsis, septic mice were treated with Exo or HExo by intravenous injection with 2 mg protein/kg body weight, or PBS as a control, 4 h post CLP surgery. Survival ratios were detected for 7 d. Septic mice treated with HExo showed significantly improved survival rates. In the sham group, no deaths occurred.
HExo decreases renal injury in a CLP-induced sepsis mouse model
CLP-induced sepsis multi-organ dysfunction permeability is a major cause of sepsis mortality. Therefore, we assessed whether Exo might ameliorate organ dysfunction in septic mice. Sepsis induced renal injury by elevating AST, BUN and ALT levels in septic vs. control mouse plasma (). However, treatment with Exo, particularly HExo, significantly elongated the organ injuries. We further explored the effects of Exo on vascular leakage. CLP mice exhibited greater kidney vascular leakage, as estimated according to Evans blue tissue dispersion, but opposite effects were observed after treatment with Exo, particularly HExo ().
Figure 2. Effects of ADSC exosomes on organ dysfunction and vascular leakage in CLP-induced sepsis. (a-c) Plasma levels of AST (a), ALT (b) and BUN (c), 24 hr after CLP. **p < 0.01, ***p < 0.001 vs sham. ##p < 0.01, ###p < 0.001 vs CLP-PBS. (d) Vascular leakage in the kidney, measured via injection of Evans blue dye at 24 hr after CLP. ***p < 0.001 vs sham. ###p < 0.001 vs CLP-PBS.
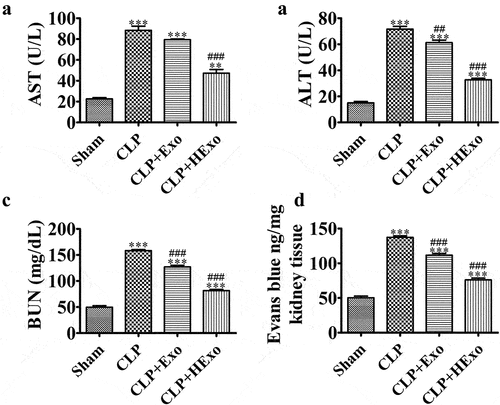
Exo treatment decreases inflammatory factor expression in CLP-induced sepsis
Sepsis is correlated with the systemic inflammatory response, partially through chemokines and cytokines. Therefore, we detected whether treatment with Exo, particularly HExo, might have a greater effect on decreasing inflammatory factor expression in the plasma of septic mice. The CLP significantly increased pro-inflammatory cytokines, including IL-6, TNF-α, IL-1β and the chemokine MCP-1. However, treatment with Exo, particularly HExo, significantly elongated these increases ().
Mmu_circ_0001295 has important functions in HExo mediated protective effects against CLP-induced sepsis
Prior data suggested that Exo are therapeutic in some conditions [Citation26–28], although the role of Exo in sepsis-induced lung injury remains unknown. The circRNAs form a substantial amount of ncRNAs, which function in various pathologies such as sepsis-induced acute lung injury [Citation29,Citation30]. To investigate the therapeutic mechanism, we used NGS to identify the total differential circRNA expression in Exo and HExo (). We used RT-qPCR to validate the six circRNAs that were most significantly upregulated. mmu_circ_0001295 showed the highest expression (). RT-qPCR data also confirmed that mmu_circ_0001295 expression decreased in the plasma under CLP-induced sepsis ().
Figure 4. mmu_circ_0001295 plays an important role in HExo’s protective effects against CLP-induced sepsis. (a) Heat map showing the expression of circRNA between Exo and HExo. (b) RT-qPCR detection showing the expression of eight upregulated circRNAs in both Exo and HExo. The data are presented as the mean ± SD. ***p < 0.001 vs Exo. (c) RT-qPCR detection showing the expression of mmu_circ_0001295 in plasma after CLP-induced sepsis. The data are presented as the mean ± SD. ***p < 0.001 vs sham.
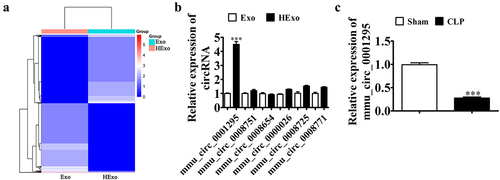
Downregulation of mmu_circ_0001295 decreases the therapeutic effect of Exo on organ dysfunction in CLP-induced sepsis
Mouse survival was detected for 7 d. Septic mice administered HExo showed a significantly increased survival rate. However, downregulation of mmu_circ_0001295 inhibited the therapeutic effect of HExo by decreasing the survival rate in CLP-induced sepsis (). Treatment with Exo, particularly HExo, significantly elongated these organ injuries. However, the downregulation of mmu_circ_0001295 inhibited the therapeutic effect of HExo in elongating these organ injuries (). We also explore the effects of Exo on vascular leakage. The results indicated that downregulation of mmu_circ_0001295 inhibited the therapeutic effect of HExo in blocking CLP-induced kidney vascular leakage, as evaluated via Evans blue tissue dispersion ().
Figure 5. Downregulation of mmu_circ_0001295 decreases the therapeutic effects of ADSC exosomes on organ dysfunction and vascular leakage in CLP-induced sepsis. (a) Mice were subjected to CLP and treated with HExo, si-circ-HExo (2 mg protein/kg body weight) or PBS. Survival rates were monitored for a total of 168 h (7 days). **p < 0.01 vs. CLP-PBS. #p < 0.05 vs CLP-Exo. (b-d) Plasma levels of AST (b), ALT (c) and BUN (d), measured 24 h after CLP. **p < 0.01, ***p < 0.001 vs sham. ###p < 0.001 vs CLP-PBS. (e) Vascular leakage in the kidney, measured via injection of Evans blue dye at 24 hr after CLP. ***p < 0.001 vs sham. ###p < 0.001 vs CLP-PBS.
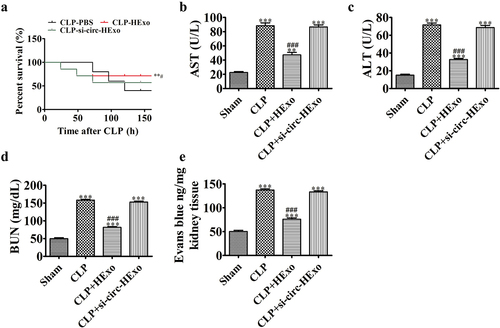
Downregulation of mmu_circ_0001295 decreases the effects of HExo on inhibiting inflammatory factor expression in CLP-induced sepsis
Previous studies have indicated that HExo has a greater effect than Exo on decreasing chemokine and cytokine expression in septic mouse plasma. CLP significantly increased the pro-inflammatory cytokines IL-6, TNF-α and IL-1β, and the chemokine MCP-1. However, the downregulation of mmu_circ_0001295 inhibited the therapeutic effects of HExo in elongating these increases ().
Discussion
Sepsis is systemic inflammation occurring because of host immune response dysregulation during infection. Sepsis affects approximately 30 million people worldwide, causing 6 million deaths every year [Citation31]. AKI is a general sepsis complication, which is correlated with morbidity and mortality [Citation32]. Previous studies have found that exosomes from endothelial progenitor cells improve outcomes in a murine model of sepsis [Citation22]. The current study revealed that Exo from ADSCs ameliorated microvascular dysfunction in CLP-induced mouse sepsis, and Exo from hypoxically pretreated ADSCs had better therapeutic effects. The results indicated that treatment with HExo increased survival, decreased vascular leakage, attenuated multiorgan failure and suppressed levels of circulating chemokines and cytokines. Thus, HExo inhibit multiple targets that play critical roles in sepsis response pathways, including permeability, and cytokine-mediated inflammation.
circRNA function is indispensable in regulating the microenvironment of sepsis [Citation33]. To detect Exo protective mechanisms in sepsis-induced renal injury, we used high-throughput sequencing to assess circRNA expression between Exo and HExo. The results indicated that HExo had higher mmu_circ_0001295 expression than Exo. These findings suggest that the protective effect of HExo against CLP-induced mouse sepsis may be related to mmu_circ_0001295 delivery. The data also showed that mmu_circ_0001295 was highly abundant in HExo, and its expression decreased in CLP-induced sepsis. Furthermore, we showed that downregulation of mmu_circ_0001295 decreased the therapeutic effects of HExo upon organ dysfunction and vascular leakage in CLP-induced sepsis. mmu_circ_0001295 downregulation also decreased the inhibitory effect of HExo on inflammatory factor expression in CLP-induced sepsis; thus, mmu_circ_0001295 had an essential role in Exo-mediated therapeutic effects. However, the regulatory mechanism still requires further study.
Conclusions
This research illustrated the feasibility of using exosomes as novel therapeutic targets in sepsis-induced renal injury. Exo attenuated the sepsis-induced renal injury through delivering mmu_circ_0001295. However, the regulatory mechanism requires further study.
Disclosure statement
No potential conflict of interest was reported by the author(s).
Additional information
Funding
References
- Hoste EA, Bagshaw SM, Bellomo R, et al. Epidemiology of acute kidney injury in critically ill patients: the multinational AKI-EPI study. Intensive Care Med. 2015;41(8):1411–1423.
- Uchino S, Kellum JA, Bellomo R, et al. Acute renal failure in critically ill patients: a multinational, multicenter study. JAMA. 2005;294(7):813–818.
- Thakar CV, Christianson A, Freyberg R, et al. Incidence and outcomes of acute kidney injury in intensive care units: a Veterans Administration study. Crit Care Med. 2009;37(9):2552–2558.
- Murugan R, Kellum JA. Acute kidney injury: what’s the prognosis? Nat Rev Nephrol. 2011;7(4):209–217.
- Zheng G, Huang L, Tong H, et al. Treatment of acute respiratory distress syndrome with allogeneic adipose-derived mesenchymal stem cells: a randomized, placebo-controlled pilot study. Respir Res. 2014;15:39.
- Gonzalez-Rey E, Anderson P, Gonzalez MA, et al. Human adult stem cells derived from adipose tissue protect against experimental colitis and sepsis. Gut. 2009;58(7):929–939.
- Peled A, Petit I, Kollet O, et al. Dependence of human stem cell engraftment and repopulation of NOD/SCID mice on CXCR4. Science. 1999;283(5403):845–848.
- Crisostomo PR, Markel TA, Wang Y, et al. Surgically relevant aspects of stem cell paracrine effects. Surgery. 2008;143(5):577–581.
- Wu J, Wang Y, Li L. Functional significance of exosomes applied in sepsis: a novel approach to therapy. Biochim Biophys Acta Mol Basis Dis. 2017;1863(1):292–297.
- Katsuda T, Kosaka N, Takeshita F, et al. The therapeutic potential of mesenchymal stem cell-derived extracellular vesicles. Proteomics. 2013;13(10–11):1637–1653.
- Yu B, Zhang X, Li X. Exosomes derived from mesenchymal stem cells. Int J Mol Sci. 2014;15(3):4142–4157.
- Lee RH, Kim B, Choi I, et al. Characterization and expression analysis of mesenchymal stem cells from human bone marrow and adipose tissue. Cell Physiol Biochem. 2004;14(4–6):311–324.
- Han Y, Ren J, Bai Y, et al. Exosomes from hypoxia-treated human adipose-derived mesenchymal stem cells enhance angiogenesis through VEGF/VEGF-R. Int J Biochem Cell Biol. 2019;109:59–68.
- Chen LL. The biogenesis and emerging roles of circular RNAs. Nat Rev Mol Cell Biol. 2016;17(4):205–211.
- Cocquerelle C, Mascrez B, Hetuin D, et al. Mis-splicing yields circular RNA molecules. FASEB J. 1993;7(1):155–160.
- Salzman J, Chen RE, Olsen MN, et al. Cell-type specific features of circular RNA expression. PLoS Genet. 2013;9(9):e1003777.
- Guo JU, Agarwal V, Guo H, et al. Expanded identification and characterization of mammalian circular RNAs. Genome Biol. 2014;15(7):409.
- Salzman J, Gawad C, Wang PL, et al. Circular RNAs are the predominant transcript isoform from hundreds of human genes in diverse cell types. PLoS One. 2012;7(2):e30733.
- Zhang Y, Xue W, Li X, et al. The biogenesis of nascent circular RNAs. Cell Rep. 2016;15(3):611–624.
- Jin J, Shi Y, Gong J, et al. Exosome secreted from adipose-derived stem cells attenuates diabetic nephropathy by promoting autophagy flux and inhibiting apoptosis in podocyte. Stem Cell Res Ther. 2019;10(1):95.
- Raeven P, Zipperle J, Drechsler S. Extracellular vesicles as markers and mediators in sepsis. Theranostics. 2018;8(12):3348–3365.
- Zhou Y, Li P, Goodwin AJ, et al. Exosomes from endothelial progenitor cells improve the outcome of a murine model of sepsis. Mol Ther. 2018;26(5):1375–1384.
- Fan H, Goodwin AJ, Chang E, et al. Endothelial progenitor cells and a stromal cell-derived factor-1alpha analogue synergistically improve survival in sepsis. Am J Respir Crit Care Med. 2014;189(12):1509–1519.
- Gao W, Wang X, Si Y, et al. Exosome derived from ADSCs attenuates ultraviolet B-mediated photoaging in human dermal fibroblasts. Photochem Photobiol. 2021;97(4):795–804.
- Kim D, Kang H. Exercise training modifies gut microbiota with attenuated host responses to sepsis in wild-type mice. FASEB J. 2019;33(4):5772–5781.
- Zhao H, Shang Q, Pan Z, et al. Exosomes from adipose-derived stem cells attenuate adipose inflammation and obesity through polarizing M2 macrophages and Beijing in white adipose tissue. Diabetes. 2018;67(2):235–247.
- Hong P, Yang H, Wu Y, et al. The functions and clinical application potential of exosomes derived from adipose mesenchymal stem cells: a comprehensive review. Stem Cell Res Ther. 2019;10(1):242.
- Qu Y, Zhang Q, Cai X, et al. Exosomes derived from miR-181-5p-modified adipose-derived mesenchymal stem cells prevent liver fibrosis via autophagy activation. J Cell Mol Med. 2017;21(10):2491–2502.
- He Y, Sun Y, Peng J. Circ_0114428 regulates sepsis-induced kidney injury by targeting the miR-495-3p/CRBN axis. Inflammation. 2021;44(4):1464–1477.
- Ma X, Zhu G, Jiao T, et al. Effects of circular RNA Ttc3/miR-148a/Rcan2 axis on inflammation and oxidative stress in rats with acute kidney injury induced by sepsis. Life Sci. 2021;272:119233.
- Fleischmann C, Scherag A, Adhikari NK, et al. Assessment of global incidence and mortality of hospital-treated sepsis. current estimates and limitations. Am J Respir Crit Care Med. 2016;193(3):259–272.
- Gomez H, Ince C, De Backer D, et al. A unified theory of sepsis-induced acute kidney injury: inflammation, microcirculatory dysfunction, bioenergetics, and the tubular cell adaptation to injury. Shock. 2014;41(1):3–11.
- Bao X, Zhang Q, Liu N, et al. Characteristics of circular RNA expression of pulmonary macrophages in mice with sepsis-induced acute lung injury. J Cell Mol Med. 2019;23(10):7111–7115.