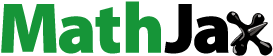
ABSTRACT
To observe the application of two instruments for the quantitative detection of total chlorine in the water in the hemodialysis industry, thereby evaluating the accuracy of the two tools and the consistency of their results, and evaluating their practical significance for the safety of hemodialysis treatment. Two methods, based on diethyl-p-phenylenediamine spectrophotometry and amperometric methods, were employed to detect the total chlorine concentration in running water and in activated carbon tank effluent. Correlation analysis was performed to evaluate the accuracy of the two instruments. The Bland–Altman test was used to evaluate the consistency of the two methods. The total chlorine tester showed high accuracy and good repeatability in terms of detecting the total chlorine concentration in running water and activated carbon tank effluent. The residual chlorine sensor had high accuracy and good repeatability for detecting the concentrations of total and free chlorine, respectively, in running water. When detecting the concentrations of total and free chlorine in the effluent of the activated carbon tank, the two test results showed a moderate correlation. The two detection methods had good consistency for the detection of total chlorine concentrations in running water and activated carbon tank effluent. The two reviewed methods can monitor changes in the total chlorine in running water and activated carbon tank effluent. It is important to take timely measures when the total chlorine concentration of the activated carbon tank effluent reaches a certain warning value, and therefore to better ensure the safety of hemodialysis treatment.
Graphical abstract
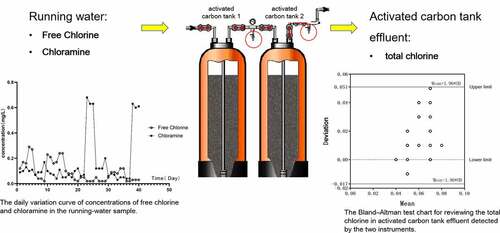
1. Background
Urban waterworks typically add chlorine to domestic water to kill or inhibit pathogenic microorganisms in the water. Following chlorination, the concentration of available chlorine in water is known as ‘residual’ or ‘total’ chlorine. In its existing form, chlorine can be divided into free and combined chlorine. The former refers to chlorine in the form of dissolved elemental chlorine, hypochlorous acid, or hypochlorite ions, while the latter denotes chlorine in the form of chloramine and organic chlorine. The combined chlorine in running water is primarily chloramine.
During hemodialysis treatment, all chlorine in the water must be removed using an activated carbon device. Free chlorine damages the reverse osmosis membrane and shortens its service life [Citation1], while chloramine can oxidize human hemoglobin to methemoglobin to induce hemolytic anemia [Citation2]. Several global reports are available on hemolytic anemia in dialysis patients caused by excessive chloramine [Citation3–8]. At present, the bulk of dialysis treatments in Beijing employ visual colorimetry to detect the total chlorine content in water. However, the subjective factors of this method can have a significant impact on its results. Also, changes in the total chlorine cannot be monitored and the quantitative detection of total chlorine is rarely used in the hemodialysis industry. The current main quantitative detection methods for total chlorine in water are N, N-1, 4-diethyl-p-phenylenediamine (DPD) titration, DPD spectrophotometry and the amperometric method. The DPD titration method is mainly used in the laboratory. DPD spectrophotometry is recommended by the International Organization for Standardization (ISO) as the standard method for the determination of free and total chlorine in water in many countries [Citation9]. The amperometric method is an electrochemical technique with a wide range of applications. Electrochemical biosensors have been shown to be a promising solution for the detection of microRNAs with high sensitivity, simplicity, low cost, and easy miniaturization for bioanalysis and early tumor diagnosis [Citation10,Citation11].
In this study, two instruments that can quickly and quantitatively detect the level of total chlorine on-site were used to detect the total chlorine concentration in running water and in activated carbon tank effluent. We observe daily changes in free chlorine and chloramine concentrations in running water, evaluated the accuracy of the two methods and the consistency of the test results, and explored the correlation between free chlorine and chloramine in running water. The study holds important clinical regulatory significance for ensuring the safety of hemodialysis treatment.
2. Methods
2.1. Study context
The water treatment system of our hospital was selected as the research environment. The system’s nominal water production is 18 L/min. Two activated carbon tanks are connected in a series (specifications, 1465, backwashed twice a week). The concentrations of free and total chlorine in running water and the activated carbon tank effluent were detected, and the concentration of chloramine was calculated by subtracting the free chlorine concentration from the total chlorine concentration.
2.2. Experimental equipment
A China Huankai total chlorine tester (hereafter referred to as a ‘total chlorine tester’), based on DPD spectrophotometry, was used in this study. The measurement range values were as follows: total chlorine, 0–3.00 mg/L; wavelength, 525 nm.
A Palintest residual chlorine sensor (United Kingdom; hereafter referred to as a ‘residual chlorine sensor’), based on the amperometric method, was used in this study. The measurement range values in this regard were as follows: free residual chlorine 0.02–10.00 mg/L; total chlorine, 0.05–00 mg/L; water temperature, 0–100°C.
2.3. Experimental principle
The principle of DPD spectrophotometry refers that under a basicity/alkalinity (pH) of 6.2–6.5, DPD reacts rapidly with free residual chlorine in the water to produce a red color. If excess potassium iodide is present, the combined chlorine (primarily monochloramine) can also react with DPD to show a color when catalyzing potassium iodide. The total chlorine concentration can be obtained by measuring its absorbance with a spectrophotometer.
The amperometric method is an electrochemical technique, the principle of which is to apply a fixed voltage to the working electrode of the chip to make the chemical substances on the chip’s surface react with the residual chlorine in the water; this enables the flow of electrons, i.e., a current is generated, and the size of the current is directly proportional to the concentration of chlorine in the sample. The instrument integrates the generated current strength to obtain an electrical integration area, which reflects the corresponding relationship between the total electricity and the concentration of the substance to be measured. In this way, the concentrations of free and total chlorine in the water can be calculated. The relevant reaction formulas are as follows:
Free chlorine
Total chlorine
2.4. Experimental method
2.4.1. Sampling and measurement
Running water and activated carbon tank effluent were sampled once a day at 8:00 for 40 days. A total chlorine tester was used to detect the total chlorine concentration in running water and activated carbon tank effluent; each sample was tested twice. A residual chlorine sensor was used to detect the concentrations of free and total chlorine in running water, respectively, and of activated carbon tank effluent; each sample was tested twice. The concentration of chloramine was calculated by subtracting the free from the total chlorine concentrations.
2.4.2. Statistical analysis
Data were statistically analyzed using the SPSS Statistics 26 software. Correlation analysis was conducted for the two total chlorine results of the same sample detected by the total chlorine tester to evaluate the tester’s accuracy. Correlation analysis was conducted for the two total chlorine results of the same sample detected by the residual chlorine sensor to evaluate the sensor’s accuracy. A Bland–Altman test was employed to evaluate the consistency of the results for the total chlorine in running water and activated carbon tank effluent samples detected by the two instruments. Correlation analysis was conducted for the concentrations of free chlorine and chloramine in the running water samples, detected and calculated by the residual chlorine sensor, to observe any correlation between free chlorine and chloramine in the running water.
3. Results
3.1 The accuracy of the total chlorine tester and the residual chlorine sensor
Correlation analysis was conducted for the two total chlorine results of the same sample detected by the total chlorine tester. The comparison of the total chlorine results for the running water samples is shown in . Pearson’s correlation coefficient was r = 1, and P < 0.01. The comparison of the total chlorine results for the activated carbon tank effluent samples is shown in . These showed that Pearson’s correlation coefficient was r = 0.948, and P < 0.01. The results indicated that this instrument had high accuracy and good repeatability for detecting the total chlorine concentration of running water and activated carbon tank effluent.
Figure 1. A comparison of the total chlorine test results for the same sample of running water using the total chlorine tester.
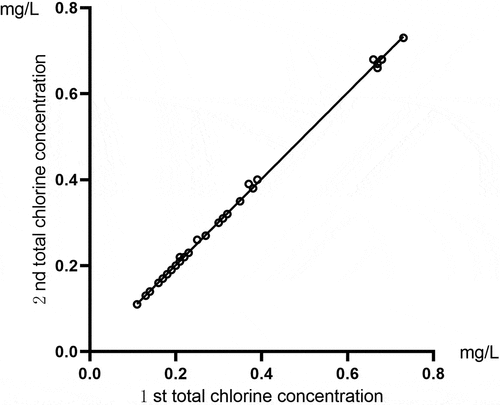
Figure 2. A comparison of the total chlorine test results for the same sample of activated carbon tank effluent using the total chlorine tester.
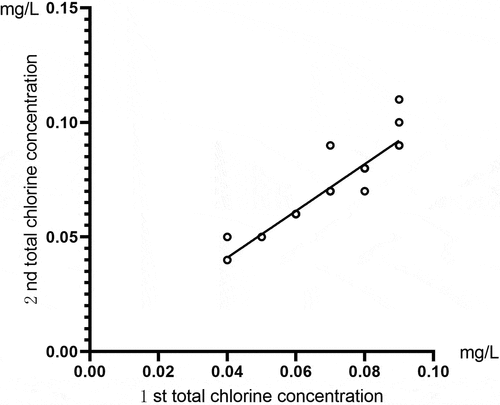
Correlation analysis was conducted for the two total chlorine results for the same sample detected by the residual chlorine sensor. The comparison of the free chlorine results for running water samples is shown in , and the comparison of total chlorine results is shown in . For the free chlorine result, Pearson’s correlation coefficient was r = 0.992, and P < 0.01. For the total chlorine result, Pearson’s correlation coefficient was r = 0.999, and P < 0.01. The comparison of the free chlorine results for activated carbon tank effluent samples is shown in , and the comparison of total chlorine results is shown in . Spearman’s rank correlation analysis was carried out to compare the results for free chlorine, which yielded a correlation coefficient of rs = 0.567, and P < 0.0. The total chlorine result showed a Pearson’s correlation coefficient of r = 0.471, and P < 0.01. These results indicated that this method had high accuracy and good repeatability for detecting the concentrations of total and free chlorine in running water. When detecting these two concentration types in the effluent of an activated carbon tank, the two test results indicated a moderate correlation.
Table 1. Comparison of two free chlorine detection results of the same sample of activated carbon tank effluent by residual chlorine sensor
Figure 3. A comparison of two free chlorine detection results for the same sample of running water using the residual chlorine sensor.
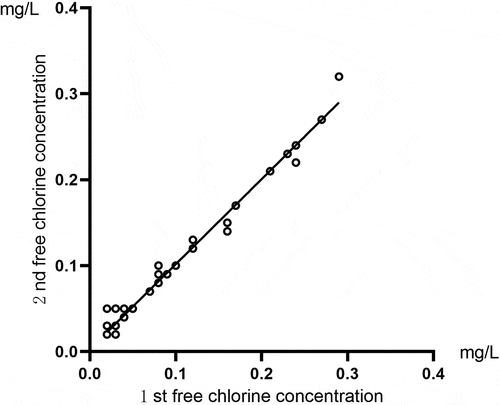
3.2. Comparison of the detected concentrations of total chlorine in running water and activated carbon tank effluent between the two detection methods
A Bland–Altman test was carried out for the concentration of total chlorine in running water measured by the two detection instruments. The detection results are shown in . Only one sample exceeded the 95% consistency limit, suggesting that the two detection methods had good consistency for the detection of total chlorine concentrations in running water.
Figure 6. The Bland–Altman test chart for reviewing the total chlorine in running water detected by the two instruments.
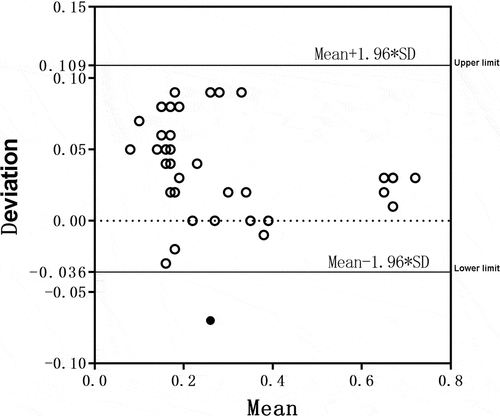
A Bland–Altman test was also conducted for the concentration of total chlorine in activated carbon tank effluent measured by the two detection instruments. The detection results are shown in . All samples were within the 95% consistency limit, suggesting that the two detection methods had good consistency for the detection of total chlorine concentrations in activated carbon tank effluent.
3.3. Correlation analysis between free chlorine and chloramine in running water
The daily variation curve of concentrations of free chlorine and chloramine in the running water sample is shown in . Daily concentrations in this regard varied significantly; the highest concentration of free chlorine was 0.29 mg/L and the lowest was 0.02 mg/L; the highest concentration of chloramine was 0.68 mg/L and the lowest was 0.03 mg/L. The concentrations of the two types are shown as a scatter diagram in . Correlation analysis was also conducted, and the results indicated the presence of a weak negative correlation between them (r = – 0.365, P = 0.020).
4. Discussion
The results of the present study suggested that the total chlorine tester and residual chlorine sensor had good consistency for the detection of total chlorine concentration in running water and activated carbon tank effluent and could be used interchangeably. The total chlorine tester had high accuracy and good repeatability for detecting chlorine in running water and activated carbon tank effluent; however, only the total chlorine concentration in the sample could be detected (not the free chlorine concentration). For detecting the free chlorine concentration, a separate free chlorine detection reagent will be required. The residual chlorine sensor could obtain free chlorine and total chlorine concentration results in a single detection with high detection accuracy and good repeatability for running water samples; however, when detecting the concentrations of total and free chlorine in the effluent of an activated carbon tank, the two test results for the same sample using this instrument were moderately correlated. Accordingly, the detection accuracy must be improved. The reason for this outcome may have been because the concentrations of total and free chlorine in the sample were close to or lower than the minimum measurement range of the instrument (free residual chlorine, 0.02–10.00 mg/L, and total chlorine, 0.05–100 mg/L). This suggested that the detection range of this instrument for low concentration areas required additional improvement to better quantitatively detect concentrations of total and free chlorine in the effluent of activated carbon tanks.
This study revealed that the daily concentrations of free chlorine and chloramine in running water can vary significantly and that there was a weak negative correlation between them. Chloramine concentration could not be calculated from free chlorine concentration, and vice versa. This was consistent with the research results of Wang et al.Citation12. A possible reason for this phenomenon is that the breakpoint chlorination method is typically used in urban waterworks which the time and amount of chlorine and ammonia in water are uncertain. China’s hygienic standard for drinking water (GB5749-2006) [Citation13] stipulates that the contact time between chlorine and free chlorine preparation (free chlorine) with water will be at least 30 min. The limit value of factory water is 4 mg/L, the residual in factory water is ≥0.3 mg/L, and the residual in water at the end of the pipe network is ≥0.05 mg/L. The contact time between monochloramine (total chlorine) and water will be at least 120 min. In this instance, the limit value of factory water is 3 mg/L, the residual in factory water is ≥0.5 mg/L, and the residual in water at the end of the pipe network is ≥0.05 mg/L. To ensure that there is sufficient residual chlorine in the water to inhibit the growth of microorganisms, and particularly to ensure that the residual free chlorine and chloramine in the water at the end of the pipe network meet the requirements, chlorine and ammonia can be added to the water at any time. This will lead to fluctuations in the concentration of free chlorine and chloramine in running water at the end of the pipe network.
Considering that the daily concentrations of free chlorine and chloramine in running water may vary significantly, requirements are needed to guard against the risk of the concentration of free chlorine and chloramine exceeding the removal threshold of an activated carbon tank. When configuring activated carbon tanks, water treatment manufacturers only consider the water consumption of a dialysis machine and not the distance between a dialysis treatment room and waterworks. The concentration of total chlorine in the running water of a hospital dialysis treatment room is closely linked to its distance from the waterworks. If the hospital dialysis room is close to the waterworks, the concentrations of free chlorine and chloramine in the running water will be high, and there will be a risk that the total chlorine will break through the removal threshold of the activated carbon tank. In this study, the maximum concentration of total chlorine in running water detected by the residual chlorine sensor reached 0.7 mg/L, and the corresponding total chlorine concentration of the activated carbon tank effluent was 0.09 mg/L, which was close to the maximum allowable concentration of 0.1 mg/L. To ensure that the total chlorine concentration is absolutely qualified, the Association for the Advancement of Medical Instrumentation recommends setting up two activated carbon tanks in a series [Citation14]. The advantage of this method is that when the total chlorine concentration of the effluent from the first activated carbon tank exceeds the standard, and the total chlorine concentration of the effluent from the second activated carbon tank is normal, the second tank can be used to replace the first activated carbon tank. Then, a new activated carbon tank can be installed as the second activated carbon tank.
The water treatment systems of many dialysis centers in China currently still adopt a method that employs a single activated carbon tank. In these cases, once the total chlorine concentration in the effluent of the activated carbon tank exceeds the standard, it is necessary to terminate the dialysis treatment, discover the cause for this, and take corresponding measures, such as backwashing and replacing the activated carbon filter material. This is likely to affect the patient’s dialysis treatment. Therefore, scholars recommend that once the chlorine in the activated carbon tank effluent is detected as greater than 0.07 mg/L, and no improvement is achieved after backwashing, maintenance, and other treatment (and other reasons are eliminated), the activated carbon filter material must be replaced [Citation15].
Our results showed large daily variability in free chlorine and chloramine concentrations in running water. As the efficacy of activated carbon on removing free chlorine and chloramine in water is affected by multiple factors. such as contact time, pH value and temperature, the chlorine and chloramine concentrations in running water may exceed the its removal threshold. Therefore, quantitatively detecting the total chlorine concentration in running water and activated carbon tank effluent by total chlorine tester and residual chlorine sensor can better monitor these changes and thereby ensure the safety of hemodialysis treatment
This study includes some limitations related to the following: (1) as a cross-sectional study, the current study cannot reflect seasonal variation trends related to residual chlorine concentration in municipal water; (2) this study was a single-center study and thus cannot represent situations in other areas of Beijing; (3) although the water treatment system of our hospital adopts two activated carbon tanks in a series, since there is no sampling port behind the first activated carbon tank, the effluent samples of the activated carbon tank are collected behind the second activated carbon tank, and no difference in the total chlorine concentration of the effluent from the two activated carbon tanks can be observed.
In future studies, the two quantitative detection methods should be used to observe long-term changes in the total chlorine concentration in running water and activated carbon tank effluent, to better understand total chlorine concentration in running water changes with seasons. We also planned to assess the actual removal efficiency of activated carbon tank effluent for free chlorine and chloramines at different water temperatures (i.e. in summer and winter).
5. Conclusion
Compared with the visual colorimetric method currently used in most dialysis centers, both of the quantitative detection methods adopted in this study were able to effect better observation of the change trends in total chlorine levels in running water and activated carbon tank effluent. Large daily variability in free chlorine and chloramine concentrations in running water was observed and there is a risk that free chlorine and chloramine concentrations would breach the charcoal canister removal threshold. A water treatment system with a single activated carbon tank can take timely measures when the total chlorine concentration of the activated carbon tank reaches the limit (e.g. > 0.07 mg/L). The total chlorine concentration of the effluent of the first and second activated carbon tanks can be monitored at the same time, and measures should be taken in time when the effluent of the first activated carbon tank is found to exceed the standard. It is suggested that conditional dialysis centers implement quantitative methods to monitor the total chlorine concentration in running water and activated carbon tank effluent.
Highlights
Free chlorine and chloramine concentrations in running water vary significantly.
The two methods can monitor the changes in total chlorine in running water and activated carbon tank.
The two methods used in this study show good consistency in detecting chlorine concentrations.
Abbreviations
diethyl-p-phenylenediamine (DPD)
MicroRNAs (miRNAs)
Ethics approval and consent to participate
This study was conducted with approval from the Ethics Committee of BeijingTongren Hospital. This study was conducted in accordance with the declaration of Helsinki.
Acknowledgements
We would like to acknowledge the hard and dedicated work of all the staff that implemented the intervention and evaluation components of the study.
Disclosure statement
No potential conflict of interest was reported by the author(s).
Additional information
Funding
References
- Powell J, Luh J, Coronell O. Amide link scission in the polyamide active layers of thin-film composite membranes upon exposure to free chlorine: kinetics and mechanisms. Environ Sci Technol. 2015;49:12136–12144.
- Stief TW. The physiology and pharmacology of singlet oxygen. Med Hypotheses. 2003;60:567–572.
- Fluck S, McKane W, Cairns T, et al. Chloramine-induced haemolysis presenting as erythropoietin resistance. Nephrol Dial Transplant. 1999;14:1687–1691.
- de Oliveira RM, de Los Santos CA, Antonello L, et al. Warning: an anemia outbreak due to chloramines exposure in a clean hemodialysis unit-an issue to be revisited. Ren Fail. 2009;31:81–83.
- Tipple MA, Shusterman N, Bland LA, et al. Illness in hemodialysis paitents after exposured to chloramines contaminated dialysate. ASAIO Trans. 1991;37:588–591.
- Junglee NA, Rahman SU, Wild M, et al. When pure is not so pure: chloramine-related hemolytic anemia in home hemodi-alysis patients. Hemodialysis lnt. 2010;14: 327–332.
- Calderaro RV, Heller L. Outbreak of hemolytic reactions associated with chlorine and chloramines residuals in hemodialysis water. Rev Saude Publica. 2001;35:481–486.
- Zhu CF, Chen YX, Zhang HM. [Clinical analysis of 32 cases of adverse reactions caused by excessive chloramine in dialysis water]. ZheJiang Yi Xue,1998;20:43–44. Chinese.
- ISO7393:1985. Water quality - Determination of free chlorine and total chlorine; 1985.
- Low SS, Ji D, Chai WS, et al. Recent progress in nanomaterials modified electrochemical biosensors for the detection of MicroRNA[J]. Micromachines. 2021;12:1409.
- Low SS, Pan Y, et al. Smartphone-based portable electrochemical biosensing system for detection of circulating microRNA-21 in saliva as a proof-of-concept[J]. Sens Actuators B Chem. 2020;308:127718.
- Wang Y, Cheng XY, Tian AH, et al. [Determination of free chlorine and chloramine in dialysis water]. Zhong Guo Xue Ye Jing Hua. 2011;10(11):588–593. Chinese.
- Ministry of health of the people’s Republic of China, China National Standardization Administration Committee[M]. Hygienic standard for drinking water.Beijing:China Standards Press; 2007. Chinese.
- Association for the Advancement of Medical Instrumentation[M]. AAMI standards and recommended practices. 2008 ed. Arlington (VA): AAMI; 1982.
- Clinical engineering technician group of Management Branch of blood purification center of China Hospital Association. [Guidance on daily work content and routine operation of blood purification clinical engineering technicians]. Zhong Guo Xue Ye Jing Hua. 2016;15(12):641–655. Chinese.