ABSTRACT
Umbilical cord blood-derived mesenchymal stem cells (UCBMSCs) have been reported to possess cardioprotective effects in diseases. However, its effects on cardiomyopathy remain unclear. This study aimed to the therapeutic effects of UCBMSC transplantation on adriamycin (ADR)-induced cardiomyopathy. UCBMSCs isolated from human UCB were identified by detecting surface markers (CD29, CD90, CD34, and CD45) using flow cytometry. The effect of UCBMSCs on left ventricular end-diastolic dimension (LVEDD), left ventricular systolic end-diastolic diameter (LVESD), left ventricular ejection fraction (LVEF), and left ventricular fraction shortening (LVFS) were determined by echocardiography. Histological changes were observed by HE and Masson staining. The serum levels of collagen-I (Col-I), brain natriuretic peptide (BNP), aspartate aminotransferase (AST), lactate dehydrogenase (LDH), creatine kinase (CK), CK-MB, interleukin (IL)-6, IL-10, and tumor necrosis factor alpha (TNF-α) were measured by corresponding kits. The protein levels of IL-6, IL-10, and TNF-α were measured by Western blotting. The isolated UCBMSCs manifested the positive expression of CD29 and CD90, and the negative expression of CD34 and CD45. UCBMSC transplantation significantly reduced LVEDD and LVESD, and increased LVEF and LVFS in ADR-induced cardiomyopathy model rats. Cardiac injury and high collagen deposition in model rats were alleviated by UCBMSC treatment. Moreover, UCBMSCs decreased the serum levels of Col-I, BNP, AST, LDH, CK, CK-MB, IL-6, IL-10, and TNF-α in model rats. Overall, UCBMSCs exert the therapeutic effects on ADR-induced cardiomyopathy through recovering the myocadiac function and alleviating the inflammatory response.
Graphical Abstract
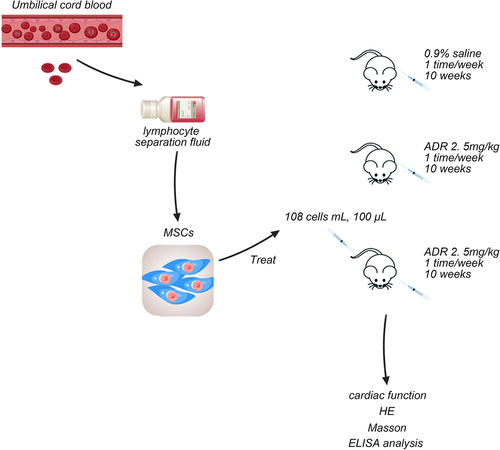
1. Introduction
Cardiomyopathy is the common disease, which is mixed in etiology [Citation1]. Adriamycin (ADR) is a favorable anti-tumor anthracycline antibiotic widely used for the treatment of various cancers [Citation2]. However, the accumulation of ADR products severe cardiotoxic effects, resulting in congestive heart failure and degenerative cardiomyopathy [Citation3]. Once symptoms of cardiomyopathy appear, treatment is difficult and has poor prognosis with a mortality rate of up to 45% within a year [Citation4]. Heart transplantation is the most effective way to treat the patients with advanced cardiomyopathy, but there are many problems in clinical practice, including donor source and economic cost [Citation1]. Therefore, it is of great significance to find novel and effective therapeutic strategies.
Umbilical cord blood (UCB) is the blood left in the umbilical cord and placenta after the umbilical cord is broken [Citation5]. Since the 1920s, UCB has been used in hematopoietic stem cell transplantation. Recent studies have found that UCB-derived mesenchymal stem cells (UCBMSCs) have the advantages of multidirectional differentiation and strong self-renew potential, which can be induced to differentiate into a variety of seed cells for the treatment of diverse diseases [Citation6].
Compared with traditional therapies, a large number of studies have shown that UCBMSCs transplantation has shown great advantages in the treatment of cardiovascular diseases. In recent years, stem cell transplantation for myocardial diseases achieves a series of commendable performance in the both basic and clinical fields. UCBMSCs for cardiac transplantation can promote myocardial regeneration and the recanalization of myocardial infarction, thereby improving left heart ejection fraction and body exercise tolerance [Citation7]. Scientists selected 29 patients with ischemic cardiomyopathy and transplanted UCBMSCs, which showed that transplanting UCBMSCs through peripheral vein and coronary artery can improve the left ventricular remodeling during the treatment of ischemic cardiomyopathy [Citation8]. In addition, UCBMSCs can also reduce the occurrence probability of hypoxia-induced cardiomyocytes and vascular endothelial cell apoptosis, thereby restricting myocardial ischemic injury [Citation9]. UCBMSCs shown the considerable clinical prospects in the treatment of diseases, due to abundant sources, easy collection, no harm to the donor, free from ethical and legal restrictions, stronger differentiation and lower immunogenicity, etc [Citation6]. With further research on hematopoietic stem cells in UCB, it is believed that the clinical application of UCBMSCs will be more prevalent.
Based on the evidences mentioned above, we speculated UCBMSCs have the ability for the treatment of ADR-induced cardiomyopathy. The aim of current study is to clarify the therapeutic effects of UCBMSCs on ADR-induced cardiomyopathy via in vivo evaluating the cardiac function, histopathological changes, and inflammatory response. Our findings may provide the potential therapeutic strategy for ADR-induced cardiomyopathy through UCBMSC transplantation.
2. Materials and methods
2.1 UCB collection
This study was approved by the Institutional Review Board of Dalian Municipal Women and Children’s Medical Center. UCB was collected from healthy infants born naturally or cesarean section under aseptic conditions, with maternal informed consent. All UCB samples were separated within 8 h using 20 U/mL heparin (anticoagulant). Obtained UCB was diluted with D-hanks solution at a ratio of 1:1, and slowly superadded to the lymphocyte separation fluid to ensure clear liquid level. The column height ratio of the diluted UCB and human lymphocyte separation fluid was 2:1, which was centrifuged at 2,000 r/min for 20 min to extract UCBMSCs in the white membrane layer. Then, UCBMSCs were collected using d-hanks and centrifuged at 1,000 r/min for 5 min. UCBMSCs were cultured in the low-glucose Dulbecco’s Modified Eagle Medium (DMEM-LG, pH 7.4).
2.2 UCBMSCs growth curve
The growth curve of UCBMSCs was plotted as previously described [Citation10]. UCBMSCs at primary and second passage (P2) were digested with 0.25% trypsin-0.01% EDTA mixture, washed with PBS, and then diluted into 1 × 104 cells/mL with fresh medium changed every 2 days. Trypsinization and cell counting for cells were conducted every day in triplicates for 30 days. The growth curves of primary and P2 UCBMSCs from primary and P2 were plotted according to cell counting. In addition, UCBMSCs at primary inoculation within 48 h and P2 within 24 h were photographed under an inverted LSM880 Airyscan microscope (Carl Zeiss, Germany).
2.3 Flow cytometry (FACS) analysis
The determination of UCBMSC-positive (CD29 and CD90) and negative (CD34 and CD45) surface biomarkers was conducted as previously described [Citation11]. P2 UCBMSCs were resuspended into 1 × 106 cells/mL, and 100 μL samples were incubated with specific fluorescence-conjugated markers (CD29, CD90, CD34, and CD45) in darkness for 30 min. Then, cells were washed with 1 mL phosphate buffered saline (PBS) to remove non-bind antibodies. Cells were transferred to flow cytometry tubes and analyzed using a Cytomics FC 500 with FlowJo software (Beckman Coulter, USA).
2.4 Animals
Male Wistar-Kyoto virus-antibody-free rats (n = 60, aged 8 weeks, weighed 200–250 g) were housed individually in cages with a layer of sawdust. Rats had free access to sterile water and pelleted food. Cages were placed in a room with a relative humidity of 40%–70%, a circumambient temperature of 23 ± 1°C, a 12-h dark/light cycle with a light intensity of 60–80 lux, and a ventilation rate of 10–15 air changes per hour. The experimental rats were purchased from the experimental animal center of Chinese People’s Liberation Army academy of military medical sciences. Animal experiments were approved by the Animal Care and Use Committee of Dalian Municipal Women and Children’s Medical Center.
2.5 Model establishment and treatment
The Wistar-Kyoto rats were randomly divided into three groups (n = 20 per group): control group, model group, and treated group. Rats in the control group were provided with normal feed without ADR and intraperitoneally injected with 0.9% saline for 10 weeks (once a week). Rats in the model group were intraperitoneally injected with 2.5 mg/kg ADR for 10 weeks (once a week) [Citation12]. Rats in the treated group were intraperitoneally injected with 2.5 mg/kg ADR for 10 weeks (once a week), and then treated with 100 μL UCBMSCs (1 × 108 cells/mL) by tail vein injection. Rats were euthanized after anesthesia with 35 mg/kg pentobarbital sodium solution for subsequent experiments. ADR was purchased from Feed Research Institute of Chinese Academy of Agricultural Sciences.
2.6 Determination of cardiac function
Rats were anesthetized with an intraperitoneal injection of 35 mg/kg pentobarbital sodium solution. Echocardiography was performed for the determination of cardiac function according to previously reported method [Citation13]. The cardiac function was determined by the measurement of left ventricular end-diastolic dimension (LVEDD), left ventricular systolic end-diastolic diameter (LVESD), left ventricular ejection fraction (LVEF), and left ventricular fraction shortening (LVFS).
2.7 Measurement of biochemical index
Serum samples were collected from rats and the serum levels of collagen I (Col-I), brain natriuretic peptide (BNP), interleukin (IL)-6, IL-10, and tumor necrosis factor alpha (TNF-α) were measured using corresponding enzyme linked immunosorbent assay (ELISA) kits. The levels of aspartate aminotransferase (AST), lactate dehydrogenase (LDH), creatine kinase (CK) and isozyme (CK-MB) in serum were detected using the corresponding kits purchased from the Jiancheng Bioengineering Institute (Nanjing, China) according to the provided instruction.
2.8 Histological staining
Rats were euthanized after anesthesia with 35 mg/kg pentobarbital sodium solution, and the myocardial tissues were harvested for hematoxylin and eosin (H&E) and Masson’s trichrome staining [Citation14]. Myocardial tissues were fixed in 4% paraformaldehyde and 48 h and dehydrated with alcohol. Next, tissues were embedded in paraffin and cut into 5 µm-thickness sections. Staining of tissues was conducted according to the manufacturer’s protocols of HE and Masson staining kits (Bogoo, China). Tissues were then sealed with neutral resin and observed under a fluorescence microscope (Nikon 80i, Japan).
2.9 Western blotting
Western blot analysis was performed as previously described [Citation15].Total protein was extracted from myocardial tissues using lysis buffer (Sigma-Aldrich, MD, USA). Proteins were separated on 10% SDS-PAGE and then transferred onto polyvinylidene fluoride (PVDF) membranes. The membranes were incubated with primary antibodies (anti-IL-6, anti-IL-10, anti-TNF-α, and anti-GAPDH; 1:500; ZSGB-BIO, China) at 4°C overnight. Following that, membranes were incubated with corresponding horseradish peroxidase-conjugated secondary antibodies for 1 h. Protein bands were images an Odyssey Infrared Imaging System with ImageJ software. The relative protein expression was calculated by normalizing to GAPDH.
2.10 Statistical analysis
All data were presented as the mean ± standard error of mean. GraphPad Prism 7.0 was used for statistical analysis. One-way ANOVA and student’s two tailed unpaired t-test were used to compare differenced among groups. In all cases, statistical significance was concluded where the P-value was < 0.05.
3. Results
3.1 Biological identification of UCBMSCs
UCBMSCs, as a novel seed cell for bioengineering, have been gradually accepted as public health [Citation16]. UCBMSCs were isolated from human UCB in this study and were identified. Under the microscope, the newly isolated UCBMSCs showed the round morphology with different sizes. After 7 days of primary culture, the round UCBMSCs were tended to transform into spindle cells. With the culture extension, adherent growth cells gradually formed colonies and were arranged in a vortex pattern with obvious orientation. After 4 weeks, UCBMSCs were fused into monolayer and grew rapidly. In the P2 generation, cell morphology was tended to be uniform spindle fibroblast-like cells ().
Figure 1. The biological identification of umbilical cord blood-derived mesenchymal stem cells (UCBMSCs). (a) The morphology of UCBMSCs. Scale bar = 500 µm. (b) The growth curves of the primary and second passage (P2) UCBMSCs. (c) The FACS analysis for surface antibodies of UCBMSCs (CD29, CD90, CD34, and CD45).
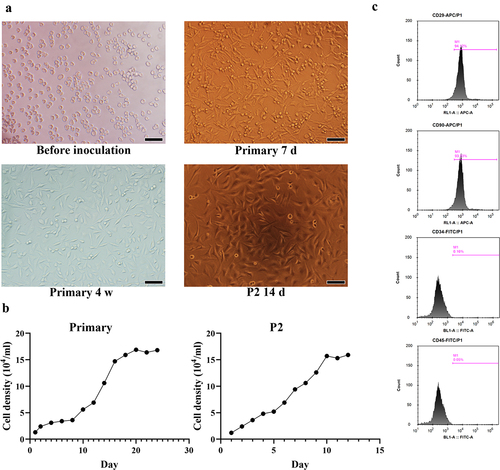
Moreover, the proliferation ability of primary and P2 UCBMSCs were identified by the establishment of growth curve. During the first 8 day of primary UCBMSC culture, there was no significant difference in the cell density. However, from days 9 to 20, cell density presented a remarkable increase. For P2 UCBMSCs, the cell density exhibited a significant increase from 1st to 10th day (). Furthermore, the cell surface antigen markers of UCBMSCs at P2 was analyzed. As displayed in , the extracted UCBMSCs showed the positive expression of CD29 and CD90, and the negative expression of CD34 and CD45.
3.2 Cardiac function was decreased in ADR-induced cardiomyopathy model rats
Cardiomyopathy rat model was established by intraperitoneal injection with ADR. LVEDD, LVESD, LVEF, and LVFS are pre-load dependent parameters indicating the contractility and function of left ventricular. As shown in , the LVEDD and LVESD in ADR-induced cardiomyopathy model rats were remarkably increased, whereas LVEF and LVFS were reduced as compared with control rats (P < 0.001). In addition, Col-I is an important component of the myocardial interstitium, which affects cardiac function. We found that Col-I level was significantly increased in ADR-induced cardiomyopathy model rats when compared with that in control rats (P < 0.05) (). BNP is produced by heart and blood vessels, the level of which will increased when heart failure. In patients with cardiomyopathy, increased ventricular wall stress and increased filling pressure promote the release of BNP in the blood [Citation17]. Consistently, the serum BNP level in ADR-induced cardiomyopathy model rats is significantly higher than that in control rats (P < 0.01) (). Moreover, the histopathological changes in myocardial tissues were detected by HE and Masson staining. HE staining showed that there were many necrotic and fibrotic areas of cardiomyocytes, interstitial edema, inflammatory cell infiltration, and disordered arrangement of cardiomyocytes in myocardial tissues of model rats when compared with that in control rats (). Masson staining is used to stain cardiac collagen, which showed that cardiac tissues from ADR-induced cardiomyopathy model rats manifested higher collagen deposition than that from control rats (). Furthermore, AST, LDH, CK, and CK-MB are myocardial injury markers, and the serum levels of them in ADR-induced cardiomyopathy model rats were significantly higher than those in control rats (P < 0.05) ().
Figure 2. The cardiac function determination of Adriamycin (ADR) induced-cardiomyopathy model rats. (a) The electrocardiograms of control and model rats. (b) The detection of left ventricular end-diastolic dimension (LVEDD), left ventricular systolic end-diastolic diameter (LVESD), left ventricular ejection fraction (LVEF), and left ventricular fraction shortening (LVFS) in control and model rats. ***P < 0.001 vs. the control group.
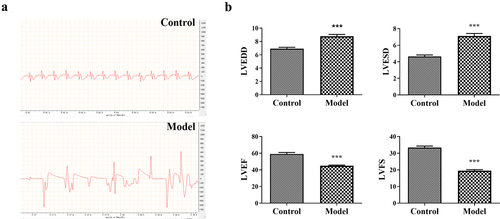
Figure 3. The evaluation of cardiac function and inflammatory response in ADR-induced cardiomyopathy model rats. (a-b) The contents of collagen-I (Col-I) and brain natriuretic peptide (BNP) in rats. (c) HE and Masson staining for the histopathological evaluation of myocadiac tissues in rats. Scale bar = 50 µm. (d-e) The serum levels of aspartate aminotransferase (AST), lactate dehydrogenase (LDH), creatine kinase (CK), CK-MB, interleukin (IL)-6, IL-10, and tumor necrosis factor alpha (TNF-α) in rats. *P < 0.05, **P < 0.01, and ***P < 0.001 vs. the control group.
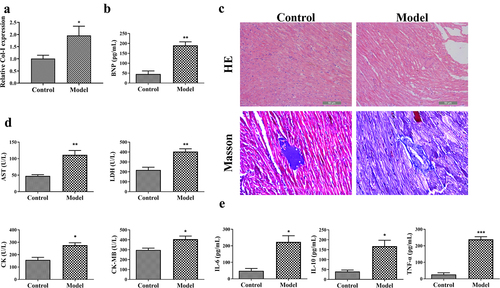
3.3 Inflammatory response was enhanced in ADR-induced cardiomyopathy model rats
Inflammatory response is an important pathological feature of cardiomyopathy. Pro-inflammatory cytokines (IL-6 and TNF-α) and anti-inflammatory effector IL-10 are important cell factors participating in immune response [Citation18]. Our study found that the serum levels of IL-6, IL-10, and TNF-α in ADR-induced cardiomyopathy model rats were significantly increased in comparison to those in control rats (P < 0.05) ().
3.4 UCBMSCs induced the cardiac function recovery of ADR-induced cardiomyopathy model rats
To explore the therapeutic effects of UCBMSCs on ADR-induced cardiomyopathy, model rats were transplanted with UCBMSCs by tail vein injection. Compared to that of model rats, LVEDD and LVESD were significantly decreased, while LVEF and LVFS were significantly increased in rats after UCBMSC treatment (P < 0.01) (). In addition, the serum levels of Col-I, BNP, AST, LDH, CK, and CK-MB all presented a decreasing tendency in ADR-induced cardiomyopathy model rats after UCBMSC treatment (P < 0.05) (). Moreover, HE and Masson staining manifested the restored myocadiac injury and reduced collagen deposition in ADR-induced cardiomyopathy model rats treated with UCBMSCs ().
Figure 4. UCBMSCs treatment recovered the cardiac function of ADR-induced cardiomyopathy model rats. The detection of LVEDD, LEVSD, LVEF and LVFS in rats. *P < 0.05, ***P < 0.001 vs. the control group; ##P < 0.01, ###P < 0.001 vs. the model group.
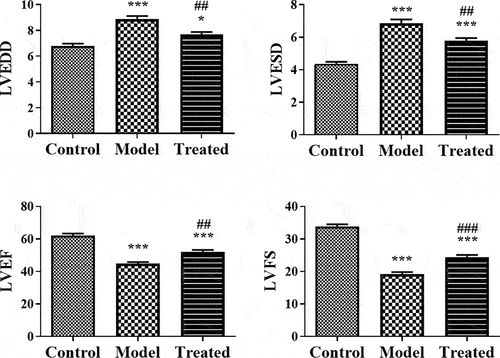
Figure 5. UCBMSCs treatment ameliorated the cardiac injury and inflammatory response of ADR-induced cardiomyopathy model rats. (a-b) The levels of Col-I and BNP in rats. (c) HE and Masson staining for the histopathological evaluation of myocadiac tissues in rats. Scale bar = 50 µm. (d-e) The serum levels of AST, LDH, CK, CK-MB, IL-6, IL-10, and TNF-α in rats. (f) The protein expression of IL-6, IL-10, and TNF-α in rats. *P < 0.05, **P < 0.01, and ***P < 0.001 vs. the control group; #P < 0.05, ##P < 0.01 vs. the model group.
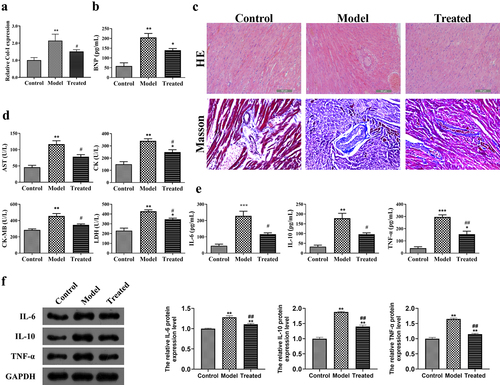
3.5 UCBMSCs ameliorated the inflammatory response in ADR-induced cardiomyopathy model rats
Further, the inflammatory response of ADR-induced cardiomyopathy model rats after UCBMSCs treatment were determined. The serum levels and protein expression of IL-6, IL-10, and TNF-α in model rats were significantly reduced after UCBMSC transplantation (P < 0.05) ().
4. Discussion
Cardiomyopathy is characterized by myocardial dysfunction, which can be caused by the usage of the anti-tumor drug ADR [Citation19]. Currently, treatment for ADR-induced cardiomyopathy mainly depends on antioxidant therapy; however, the therapeutic effects are limited [Citation20]. UCB is collected from the umbilical cord and placenta after childbirth, which is considered as a valuable source of cells for disease treatment [Citation21]. UCBMSCs from umbilical cord blood possess powerful differentiation and immunoregulatory potential, which is a promising source for therapeutic applications [Citation22]. The present study confirmed the therapeutic effects of UCBMSC transplantation on ADR-induced cardiomyopathy via the establishment of rat model.
UCBMSCs possess multi-lineage differentiation potential with high immunosuppressive activity [Citation22]. The typical morphology of UCBMSCs presents plastic adherent typical fibroblastic population. El-Sherbiny et al. demonstrated that the morphology of undifferentiated UCBMSCs transforms from round to spindle fibroblast-like cells during culture [Citation23]. Consistently, in this study, UCBMSCs were extracted from UCB and presented round morphology. After 14-day culture (P2), the morphology of UCBMSCs transformed to spindle fibroblast-like cells. In addition, CD29 and CD90 are positive biomarkers, while CD34 and CD45 are lacking hematopoietic markers in UCBMSCs [Citation24]. Our study confirmed the positive expression of CD29 and CD90, and the negative expression of CD34 and CD45 in UCBMSCs. These results indicated that UCBMSCs were successfully isolated and cultured, which can be used for the treatment of ADR-induced cardiomyopathy in the subsequent experiments.
To investigated the therapeutic effects of UCBMSC transplantation on ADR-induced cardiomyopathy, the ADR-induced cardiomyopathy rat model was established. Echocardiography aids can contribute to evaluating and determining the cardiac dysfunctional severity of cardiomyopathy. LVEDD, LVESD, LVEF, and LVFS, derived from echocardiography, are critical parameters for the assessment of cardiac function [Citation25]. Higher LVEDD and LVESD values represents more severe cardiac dysfunction, which are negatively correlated with LVEF and LVFS [Citation13]. We found that LVEDD and LVESD were increased in rats with ADR-induced cardiomyopathy, while LVEF and LVFS were decreased. This result is congruent with previous studies, indicating that cardiac function is disordered in ADR-induced cardiomyopathy [Citation26,Citation27]. Col-I and BNP are also two pivotal parameters used to indicate the myocardial function and stretch [Citation12,Citation28,Citation29]. Pang et al. found that the levels of Col-I and BNP were increased in dilated cardiomyopathy [Citation12]. Our study also showed the increased Col-I and BNP levels in ADR-induced cardiomyopathy rats, indicating that myocardial function was disordered. AST, LDH, CK, and CK-MB are essential serum cardiac biomarkers evaluating myocardial injury [Citation30]. It has been reported that the serum levels of AST, LDH, CK, and CK-MB raise in ADR-induced cardiomyopathy, which is in line with our results [Citation31]. HE and Masson staining also showed severe cardiac injury and collagen deposition in myocadiac tissues of ADR-induced cardiomyopathy rats. Furthermore, inflammatory response is also a important pathological feature of cardiomyopathy [Citation32]. Pro-inflammatory cytokines IL-6 and TNF-α appear to make a significant contribution to the pathophysiology of cardiomyopathy and heart failure [Citation33]. In contrast to pro-inflammatory mediators, anti-inflammatory cytokine IL-10 influences the inflammatory activation of monocytes [Citation34]. Our results presented that the serum levels of IL-6, IL-10, and TNF-α were increased in ADR-induced cardiomyopathy rats, proving the severe inflammatory response in ADR-induced cardiomyopathy.
UCBMSCs have a promising application prospect due to their lower immunogenicity, higher proliferation factors, and higher transfection efficiency [Citation9]. Accumulating evidence indicated that UCBMSCs have cardioprotective effects in various diseases. Song et al. demonstrated that UCBMSCs inhibit ferroptosis and attenuate myocardial injury in acute myocardial infarction mice [Citation9]. Lee et al. proved the therapeutic potency of UCBMSCs on myocardial infarction, which is evidenced by decreasing LVEDD and LVESD, and increasing LVEF and LVSF [Citation35]. Zhao et al. indicated that transplantation of UCB-derived cellular fraction improves left ventricular function and remodeling after myocardial ischemia or reperfusion [Citation36]. In the present study, we found that UCBMSCs decreased the LVEDD and LVESD, and increased the LVEF and LVSF in ADR-induced cardiomyopathy rats, indicating UCBMSCs can ameliorate cardiac function in ADR-induced cardiomyopathy. In addition, UCBMSC transplantation reduced the levels of Col-I, BNP, AST, LDH, CK, CK-MB in ADR-induced cardiomyopathy rats. Histopathological staining showed UCBMSCs alleviated cardiac injury and collagen deposition in myocadiac tissues of ADR-induced cardiomyopathy rats. These results indicate that UCBMSCs can recover myocadiac function in ADR-induced cardiomyopathy. Furthermore, we found that UCBMSCs downregulated the serum levels of IL-6, IL-10, and TNF-α in ADR-induced cardiomyopathy rats, indicating that UCBMSCs exerts an anti-inflammatory effect in ADR-induced cardiomyopathy.
5. Conclusion
This study finds that UCBMSCs recovers the myocadiac function and ameliorates the inflammatory response of ADR-induced cardiomyopathy rats. Our findings open the precedent of UCBMSC therapy for ADR-induced cardiomyopathy, and provide the theoretical basis and clinical possibility for UCBMSC therapy.
Availability of data and material
The authors declare that the data supporting the findings of this study are available within the article, or are available from the authors upon request.
Ethics approval
This study was approved by the Institutional Review Board of Dalian Municipal Women and Children’s Medical Center and was followed the principles of the Declaration of Helsinki (No. 20,170,042).
Consent to participate
The study obtained the written consent of the participants.
Conflicts of interest
No potential competing interest was reported by the authors.
Disclosure statement
No potential conflict of interest was reported by the author(s).
Additional information
Funding
References
- Brieler J, Breeden MA, Tucker J. Cardiomyopathy: an Overview. Am Fam Physician. 2017;96(10):640–646.
- Chen C, Lu L, Yan S, et al. Autophagy and doxorubicin resistance in cancer. Anticancer Drugs. 2018;29(1):1–9.
- Nebigil CG, Désaubry L. Updates in anthracycline-mediated cardiotoxicity. Front Pharmacol. 2018;9:1262.
- Schaufelberger M. Cardiomyopathy and pregnancy. Heart. 2019;105(20):1543–1551.
- Allan DS. Using umbilical cord blood for regenerative therapy: proof or promise?, Stem cells (Dayton. Ohio). 2020;38(5):590–595.
- Um S, Ha J, Choi SJ, et al. Prospects for the therapeutic development of umbilical cord blood-derived mesenchymal stem cells. World J Stem Cells. 2020;12(12):1511–1528.
- Roura S, Soler-Botija C, Bagó JR, et al. Postinfarction functional recovery driven by a three-dimensional engineered fibrin patch composed of human umbilical cord blood-derived mesenchymal stem cells. Stem Cells Transl Med. 2015;4(8):956–966.
- Roura S, Gálvez-Montón C, Bayes-Genis A. Umbilical cord blood-derived mesenchymal stem cells: new therapeutic weapons for idiopathic dilated cardiomyopathy? Int J Cardiol. 2014;177(3):809–818.
- Song Y, Wang B, Zhu X, et al. Human umbilical cord blood-derived MSCs exosome attenuate myocardial injury by inhibiting ferroptosis in acute myocardial infarction mice. Cell Biol Toxicol. 2021;37(1):51–64.
- Prat-Vidal C, Roura S, Farré J, et al. Umbilical cord blood-derived stem cells spontaneously express cardiomyogenic traits. Transplant Proc. 2007;39(7):2434–2437.
- Divya MS, Roshin GE, Divya TS, et al. Umbilical cord blood-derived mesenchymal stem cells consist of a unique population of progenitors co-expressing mesenchymal stem cell and neuronal markers capable of instantaneous neuronal differentiation. Stem Cell Res Ther. 2012;3(6):57.
- Pang XF, Lin X, Du JJ, et al. LTBP2 knockdown by siRNA reverses myocardial oxidative stress injury, fibrosis and remodelling during dilated cardiomyopathy. Acta Physiol (Oxf). 2020;228(3):e13377.
- Qiu Z, Chen W, Liu Y, et al. LncRNA AC061961.2 overexpression inhibited endoplasmic reticulum stress induced apoptosis in dilated cardiomyopathy rats and cardiomyocytes via activating wnt/β-catenin pathway. J Recept Signal Transduct Res. 2021;41(5):494–503.
- Chen D, Zhang M. GAS5 regulates diabetic cardiomyopathy via miR‑221‑3p/p27 axis‑associated autophagy. Mol Med Rep. 2021;23(2):135.
- Ruan W, Ji X, Qin Y, et al. Harmine alleviated sepsis-induced cardiac dysfunction by modulating macrophage polarization via the STAT/MAPK/NF-κB pathway, frontiers in cell and developmental biology. Frontiers in Cell and Developmental Biology. 2022;9:792257.
- Wu Q, Chen B, Liang Z. Mesenchymal stem cells as a prospective therapy for the diabetic foot, stem cells international 2016. Stem Cells International. 2016;2016:4612167.
- Medina de Chazal H, Del Buono MG, Keyser-Marcus L, et al. Stress cardiomyopathy diagnosis and treatment. JACC State-of-the-Art Review, J Am Coll Cardiol. 2018;72(16):1955–1971.
- Jin S, Yu C, Yu B. Changes of serum IL-6, IL-10 and TNF-α levels in patients with systemic lupus erythematosus and their clinical value. Am J Transl Res. 2021;13(4):2867–2874.
- Wallace KB, Sardão VA, Oliveira PJ. Mitochondrial determinants of doxorubicin-induced cardiomyopathy. Circ Res. 2020;126(7):926–941.
- Singla DK, Johnson TA, Tavakoli Dargani Z. Exosome treatment enhances anti-inflammatory M2 macrophages and reduces inflammation-induced pyroptosis in doxorubicin-induced cardiomyopathy. Cells. 2019;8(10):1224.
- Berglund S, Magalhaes I, Gaballa A, et al. Advances in umbilical cord blood cell therapy: the present and the future. Expert Opin Biol Ther. 2017;17(6):691–699.
- Stiner R, Alexander M, Liu G, et al. Transplantation of stem cells from umbilical cord blood as therapy for type I diabetes. Cell Tissue Res. 2019;378(2):155–162.
- El-Sherbiny M, Eladl MA, Ranade AV, et al. Functional beta-cells derived from umbilical cord blood mesenchymal stem cells for curing rats with streptozotocin-induced diabetes mellitus. Singapore Med J. 2020;61(1):39–45.
- Jin HJ, Bae YK, Kim M, et al. Comparative analysis of human mesenchymal stem cells from bone marrow, adipose tissue, and umbilical cord blood as sources of cell therapy. Int J Mol Sci. 2013;14(9):17986–18001.
- Li TT, Li HY, Cheng J. Changes of serum uric acid and its clinical correlation in children with dilated cardiomyopathy. Transl Pediatr. 2021;10(12):3211–3217.
- He ZH, Shao LQ, Xuan LY, et al. Effect of astragalus injection on cardiomyocyte apoptosis, endoplasmic reticulum stress and expression of connexin in cardiomyopathy rats induced by adriamycin. Zhongguo Ying Yong Sheng Li Xue Za Zhi. 2018;34(2):159–163.
- He L, Xiao J, Fu H, et al. Effect of oxidative stress on ventricular arrhythmia in rabbits with Adriamycin-induced cardiomyopathy. J Huazhong Univ Sci Technolog Med Sci. 2012;32(3):334–339.
- Novack ML, Zevitz ME. Natriuretic peptide b type test. StatPearls, StatPearls Publishing. Treasure Island (FL), 2020.
- Satoh M, Nakamura M, Akatsu T, et al. Myocardial osteopontin expression is associated with collagen fibrillogenesis in human dilated cardiomyopathy. Eur J Heart Fail. 2005;7(5):755–762.
- Hu ZJ, Zhou YQ, Zhang HB, et al. Clinical value of monitoring serum cardiac biomarkers in pulmonary thromboembolism-induced myocardial injury. Nan Fang Yi Ke Da Xue Xue Bao. 2008;28(10):1853–1855.
- Ahmed AZ, Mumbrekar KD, Satyam SM, et al. Chia seed oil ameliorates doxorubicin-induced cardiotoxicity in female wistar rats: an electrocardiographic. Biochemical and Histopathological Approach, Cardiovasc Toxicol. 2021;21(7):533–542.
- Wenzl FA, Ambrosini S, Mohammed SA, et al. Inflammation in metabolic cardiomyopathy. Front Cardiovasc Med. 2021;8:742178.
- Vadlamani L, Abraham, W.t WT. Abraham, Insights into pathogenesis and treatment of cytokines in cardiomyopathy. Curr Cardiol Rep. 2000;2(2):120–128.
- de Oliveira RT, Mamoni RL, Souza JR, et al. Differential expression of cytokines, chemokines and chemokine receptors in patients with coronary artery disease. Int J Cardiol. 2009;136(1):17–26.
- Lee EJ, Park SJ, Kang SK, et al. Spherical bullet formation via E-cadherin promotes therapeutic potency of mesenchymal stem cells derived from human umbilical cord blood for myocardial infarction. Mol Ther. 2012;20(7):1424–1433.
- Zhao L, Cheng G, Choksi K, et al. Transplantation of human umbilical cord blood-derived cellular fraction improves left ventricular function and remodeling after myocardial ischemia/reperfusion. Circ Res. 2019;125(8):759–772.