ABSTRACT
Praeruptorin A (PA) is a natural coumarin compound from the roots of Radix Peucedani and is commonly used in the treatment of certain respiratory diseases and hypertension. Although previous studies identified relaxant effects of PA on tracheal and arterial preparations, little is known about its vasodilative effects and underlying mechanisms. Here, an organ bath system and tension recording methods were used to prepare and analyze isolated rat thoracic aorta artery rings. Aorta artery rings were pre-contracted with phenylephrine and then incubated with PA, and the possible mechanism of relaxation was investigated by adding inhibitors of nitric oxide synthase (NG-nitro-L-arginine methyl ester, L-NAME), endothelial nitric oxide synthase (L-NG-nitroarginine, L-NNA), cyclooxygenase (indomethacin), guanylyl cyclase (1 H-[1,2,4]oxadiazolo [4,3-a]quinoxalin-1-one, ODQ), and KCa channels (tetraethylammonium, TEA). Our study showed that PA-induced vasodilation was blocked by L-NAME, L-NNA, and ODQ, while CaCl2-induced vasoconstriction was countered by PA. Thus, PA may exert a vasodilatory effect by influencing the amounts of endothelium-derived relaxing factors through endothelial-dependent NO-cGMP and prostacyclin pathways (such as NO and prostacyclin 2). In the rat thoracic aorta, PA reduces vasoconstriction by inhibiting Ca2+ inflow.
Graphical abstract
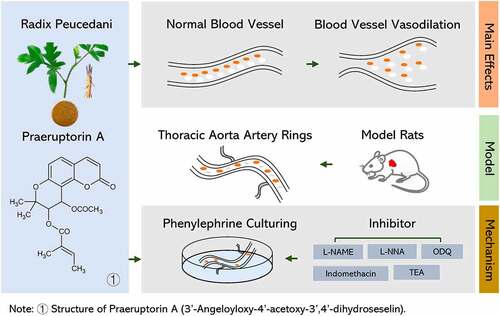
Introduction
Radix Peucedani (in Chinese, Qian Hu), the dried roots of Peucedanum praeruptorum Dunn., were first described in Miscellaneous Records of Famous Physicians (in Chinese, Ming Yi Bie Lu). It has a long history of use in diseases of the lung and respiratory system, such as cough, dyspnea, and upper airway infections [Citation1]. Qian Hu consists of a great number of chemical components, but only a few of these components have been revealed as the main bioactive constituents. These bioactive components include angular-type pyranocoumarins, (+)-praeruptorin A, (±)-praeruptorin B, (+)-praeruptorin B and (+)-praeruptorin E. These compounds have various pharmacological activities, involving vasodilation, neuroprotection, cardioprotection, hepatoprotection, and anti-platelet aggregation effects [Citation2–4]. One of these compounds in particular, praeruptorin A (PA), has been recognized as the main active component of Qian Hu, and it has been shown to have anticancer, antihypertension, and anti-inflammatory properties [Citation5–9].
Endothelial-dependent vasodilation can be decreased gradually by chronic hypertension, diabetes, and cardiovascular disease and by other factors [Citation10]. The chemical entities that mediate endothelium-dependent vasodilation may be divided into calcium antagonists, alpha 1-adrenergic antagonists, cAMP-elevating drugs, and cGMP-elevating agents. It is generally accepted that calcium-dependent phosphorylation of myosin light chains initiates smooth muscle contraction. A variety of evidence suggests that certain vasodilators inhibit calcium-dependent phosphorylation of myosin light chains and smooth muscle contraction via activation of cAMP and cGMP-dependent protein phosphorylation [Citation11].
Analogues of L-arginine are widely used inhibitors of nitric oxide synthase (NOS) activity both in vitro and in vivo, with NG-nitro-L-arginine methyl ester (L-NAME) being a commonly used analogue [Citation12]. Acute and chronic L-NAME treatment leads to changes in blood pressure and vascular reactivity due to decreased nitric oxide (NO) bioavailability [Citation13]. NO is of major importance in the control of systemic vessels, but not coronary resistance vessels. At rest and during exercise, and after treatment with L-NG-nitroarginine (L-NNA), the cyclooxygenase pathway has been found to be involved in myocardial reactive hyperemia and in the residual relaxation response to bradykinin of isolated coronary arteries [Citation14].
Importantly, NO has both inhibitory and excitatory effects on all types of respiratory neurons. Inhibitory effects involve soluble guanylyl cyclase, and they are blocked with 1 H-[1,2,4] oxadiazolo [4,3-a] quinoxalin-1-one (ODQ), whereas excitation is antagonized by uric acid and possibly mediated via peroxynitrite [Citation15]. In addition, indomethacin may have more potent vasoconstrictive activity and unique direct neuronal or NO-dependent inhibitory pathway activity [Citation16]. In blood vessels, NO causes hyperpolarization, suggesting that ATP-dependent potassium channels are involved the entire vasorelaxation process. Other calcium-dependent potassium channels (KCa) that are inhibited by small concentrations of tetraethylammonium (TEA) are likely to be involved in this same general vasorelaxant effect. Hence, we predict that multiple compounds with inhibitory activity, such as L-NAME (a nitric oxide synthase inhibitor), L-NNA (an endothelial nitric oxide synthase inhibitor), indomethacin (a cyclooxygenase inhibitor), ODQ (a guanylyl cyclase inhibitor, and TEA (a KCa channel inhibitor, might have substantive impacts on vasorelaxation.
Although it is known that PA induces tracheal smooth muscle relaxation after pre-constriction with KCl and acetylcholine [Citation3] and may open KATP channels [Citation17], the mechanisms behind these effects remain obscure. Therefore, in this study we aimed to explore the possible factors involved in the vasorelaxant effect of PA with a rat thoracic aorta ring model and by investigating interactions with important endothelium-dependent vasodilators.
Materials and methods
Animals
Adult male Sprague-Dawley rats weighing 260 ± 10 g were obtained from the Animal Breeding Center of Beijing Vital River Laboratories Company (Beijing, China). All rats were housed at 22 ± 2°C with a relative humidity of 50 ± 10% and a 12 h light/12 h dark cycle. The animals had free access to water and food. All animal experiments were approved by the Ethics Committee of the China Academy of Chinese Medical Sciences (No.20162002) and were performed according to the Guide for the Care and Use of Laboratory Animals (Council, 2010).
Drugs
The drugs used in this study were: PA (PHL83553, Sigma, USA), indomethacin (PHR1247, Sigma, USA), L-phenylephrine chloride (PE) (PHR1017, Sigma, USA), acetylcholine chloride (A2662, Sigma, USA), L-NAME (N5752, Sigma, USA), L-NG-nitroarginine (L-NNA) (N5501, Sigma, USA), 1 H-[1,2,4]oxadiazolo[4,3-a]quinoxalin-1-one (ODQ) (495,320, Sigma, USA). Indomethacin was dissolved in 0.1 M Na2CO3 solution. ODQ and PA were prepared in 20% dimethyl sulfoxide (DMSO). Others were dissolved in distilled water. The final concentration of DMSO in the bath was less than 0.5%, which had no influence on vasodilatation [Citation12].
Preparation of isolated rat thoracic aortas
An organ bath system (ALC-M, Shanghai, China), an electronic analytical balance (BP110S, Sartorius, Germany), and a tension recording method were used to prepare isolated rat thoracic aorta artery rings. The thoracic aorta was dissociated, and then connective and fat tissue were removed in a 4°C K-H solution (118.96 M NaCl, 4.73 mM KCl, 1.17 mM KH2PO4, 1.17 mM MgSO4, 25.0 M NaHCO3, 1.35 M CaCl2, and 11.1 M glucose, pH 7.4). Aorta artery rings (2 mm) were suspended by cotton threads in an organ bath containing 5 mL K-H solution, maintained at 37 ± 0.1°C and gassed with 95% O2 + 5% CO2. Rings were first stabilized with a 1.0 g weight to establish a tension of 9.8 mN for 1 h, and the solution was changed every 20 min during this period [Citation18]. The isometric contraction was recorded by a force transducer (MPA 2000, Shanghai, China) coupled to an amplifier-recorder and also to a personal computer equipped with an analog-to-digital converter board. In endothelium removal experiments, the endothelial layer was removed by gently rubbing the intimal surfaces of vessels with a cotton ball. The presence of functional endothelium was assessed by applying acetylcholine (1 μM), which induced more than 70% relaxation of vessels after precontraction with 1 μM PE. Less than 10% of relaxation caused by acetylcholine after PE pretreatment was considered to indicate endothelium denudation [Citation19,Citation20].
Effects of PA on vasorelaxation in endothelium-denuded vessel rings
PE (1 μM) was employed as the contractile agent. After stable contraction was established, constant volumes of increasing concentrations of PA (2.77 × 10−7 to 2.04 × 10−4 M) were cumulatively applied every 10 min. Vasodilatation of the endothelium-denuded vessels caused by PA was analyzed by comparing responses to those of intact vessel rings derived from the same aorta.
Endothelium-derived relaxing factor (EDRF) inhibitors in PA-induced vasorelaxation
Sustained contraction was induced in intact aorta rings by the addition of PE (1 µM) after 15 min incubation with L-NAME (300 μM), an endothelial nitric oxide synthase inhibitor (L-NNA, 300 μM), or a nonselective inhibitor of cyclooxygenase (COX) (indomethacin, 10 μM). PA (2.77 × 10−7 to 2.04 × 10−4 M) was then cumulatively applied after stable contraction was established. Thus, concentration-response curves to PA (2.77 × 10−7 to 2.04 × 10−4 M) were obtained. Control experiments were performed without pre-incubation with L-NAME, L-NAA, or indomethacin.
Effect of guanylyl cyclase inhibition on PA-induced vasorelaxation
Sustained contraction was induced by PE (1 µM) in intact aorta rings that were previously incubated for 15 min with ODQ (10 μM), a soluble guanylyl cyclase inhibitor [Citation21]. Then, concentration-response curves to PA (2.77 × 10−7 to 2.04 × 10−4 M) were obtained according to the method described above. The same experiment protocol was applied to the control group, except for incubation with ODQ [Citation22].
Effect of KCa channel inhibition on PA-induced vasorelaxation
Sustained contraction after PE (1 µM) incubation was obtained in intact aorta rings that were pretreated with tetraethylammonium (TEA, 10 mM), a KCa channel blocker, for 15 min, and concentration-response curves to PA (2.77 × 10−7 to 2.04 × 10−4 M) were subsequently obtained based on the methods described above. The same experimental protocol without incubation with TEA was used for the control group.
Role of calcium in PA-induced vasorelaxation
Denuded and intact rings were incubated in K-H solution without calcium for 1 h [Citation23]. During this period, the solution was changed every 20 min. Aorta rings were first incubated with PA (2.76 × 10−[Citation5] M) for 10 min, and then CaCl2 (6.00 × 10−5 to 4.55 × 10−3 M) was cumulatively applied every 5 min after contractile responses were induced by 1 μM PE and 60 mM KCl. Dose-response curves of aorta rings were recorded. The control group was conducted with the same experiment protocol in the absence of PA.
Data analysis
All data are presented as means ± SD. Relaxation rate was expressed as a percentage of the vasodilative response induced by PA to vasoconstriction caused by various contractile agents. The maximal relaxation response of PA was expressed as a percentage (Emax) and as an EC50, which is the concentration of the test compound that causes 50% of its maximal response (The equation of EC50 from GraphPad Prism: Y = Bottom+ (Top-Bottom)/(1 + 10^((LogEC50-X)*Hill Slope))). Values of responses to PA were calculated by GraphPad Prism software. Relaxation potency was expressed as the negative logarithm of the EC50 (pEC50). Two-way repeated measured ANOVA followed by Bonferroni’s multiple comparison was used for data analysis [Citation24]. Differences for which p< 0.05 were considered statistically significant.
Results
Experimental workflow
provides a schematic representation of our experimental approach. To explore the relaxant effect of PA from the spectrum of the endothelium, we followed this workflow to profile the differential protein expression in isolated rat thoracic aorta rings. The aorta artery rings were then pre-contracted, and PE was added. After that, the pre-contractive model was incubated with PA, and possible mechanisms of action were investigated by adding inhibitors to various proteins, including EDRF (L-NAME), endothelial nitric oxide synthase (L-NNA), COX (indomethacin), guanylyl cyclase (ODQ), and KCa channels (TEA). Ultimately, these studies were designed to reveal the mechanism by which PA induces endothelium-dependent vasorelaxation and smooth muscle relaxation ().
Figure 1. (A) Schematic diagram of experimental procedures. (B) Effect of PA on tension in PE (1 μmol/L) pre-contracted aortic rings with endothelium (+E, n = 5) or without endothelium (-E, n = 5). Relaxation (%) indicates the percentage of PE-induced contraction. Values are expressed as the mean ± SD. *p < 0.05, **p< 0.01, ***p< 0.001 vs the endothelium-denuded rings group.
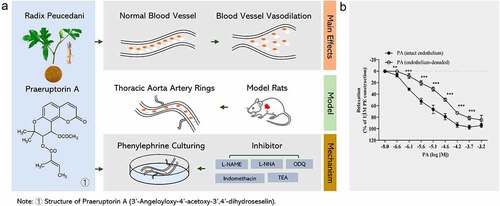
Table 1. Roles of different treatments on vasorelaxant effects of PA after PE-induced contractions in intact thoracic aorta rings
In phenylephrine (PE)-precontracted aortic rings, PA induces vasodilation
PE-induced prolonged contraction in rat thoracic aorta rings was reduced by PA in a manner that is dependent on the concentration of the drug and on the presence of the endothelium. In both endothelium-intact and -denuded rings, the vasoconstriction elicited by 1 M phenylephrine was relaxed by the cumulative addition of doses of PA (2.77 × 10−7 to 2.04 × 10−4 M) in a concentration-dependent manner. In endothelium-denuded vascular rings, the concentration-response curve to PA was dramatically shifted to the right, with a statistically significant reduction in pEC50 after endothelium removal (pEC50 = 4.83 ± 0.14 vs pEC50 = 5.63 ± 0.15, p< 0.001, n = 5) ().
Effects of L-name and indomethacin on praeruptorin-induced vasorelaxation
The PA-induced vasorelaxant response was reduced by both L-NAME () and indomethacin (). After treatment with 300 μM L-NAME, the concentration-response curve to PA was significantly shifted to the right with a reduction in pEC50 (pEC50 = 4.57 ± 0.07 vs pEC50 = 4.86 ± 0.09, p< 0.01, n = 4). In the presence of 10 μM indomethacin, the concentration-response curve of PA was shifted to the right with a reduction in pEC50 (pEC50 = 4.58 ± 0.11 vs pEC50 = 4.99 ± 0.28, p< 0.05, n= 4), indicating that indomethacin suppressed the vasorelaxant effects of PA. Furthermore, there was no significant difference observed in the vasorelaxant effect of PA following L-NNA administration as compared to the control group (pEC50 = 4.90 ± 0.29 vs pEC50 = 5.02 ± 0.25, p > 0.05).
Figure 2. The relaxant effect of PA on PE (1 μM)-pre-contracted aortic rings in the presence or absence (control(PA), n = 4) of (A) 300 μM L-NAME, (B) 10 μM indomethacin, (C) 10 μM ODQ and (D) 10 μM on vasorelaxant effects of PA. Values are expressed as the mean ± SD. *p< 0.05, **p< 0.01, ***p< 0.001 vs the control group.
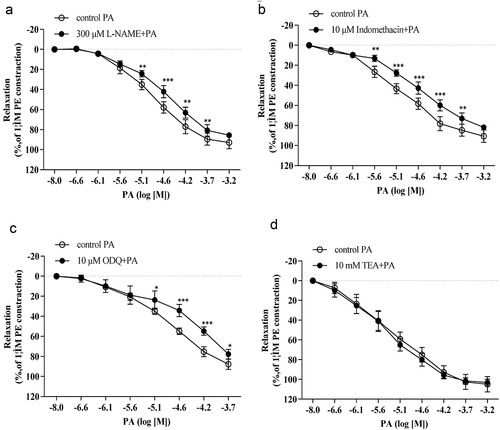
Role of guanylyl cyclase inhibitor and K+ channel blocker in PA-induced vasorelaxation
We examined the vasorelaxant effect of PA after application of a guanylyl cyclase inhibitor (ODQ) and a KCa channel blocker (TEA). As shown in , ODQ inhibited PA-induced vasorelaxation. Further inhibition was observed after the application of 10 μM of ODQ, and the vasorelaxant effects of PA were significantly reduced (pEC50 = 4.40 ± 0.10 vs pEC50 = 4.89 ± 0.16, n = 4, p < 0.01). The data presented in demonstrate that the concentration-response curve for PA was not significantly changed after incubation with 10 mM of TEA.
Effect of PA on calcium-induced vasoconstriction
Sustained contractile responses of 0.09 ± 0.02 g were induced by 60 mM KCl in intact thoracic aorta rings in K-H solution without calcium in the presence or absence of PA (2.76 × 10−[Citation5] M) when CaCl2 (6.00 × 10−5 to 4.55 × 10−3 M) was cumulatively applied every 5 min. As shown in , the maximum contractile response induced by CaCl2 was 1.31 ± 0.12 g; the calcium-induced maximum contraction was reduced by 94.66%, and the contractile response declined to 0.07 ± 0.02 g after incubation with PA (2.76 × 10−[Citation5] M). In addition, the maximum contractile response induced by CaCl2 in endothelium-denuded thoracic aorta rings was 0.90 ± 0.25 g, and this response was reduced by 101.1% after PA (2.76 × 10−[Citation5] M) incubation, where the contractile response decreased to −0.01 ± 0.007 g (). Sustained contractile responses of 0.10 ± 0.03 g were induced by 1 µM PE in intact thoracic aorta rings in K-H solution without calcium. When CaCl2 (6.00 × 10−5 to 4.55 × 10−3 M) was cumulatively applied every 5 min, the maximum contractile response induced by CaCl2 was 1.23 ± 0.29 g. After incubation with PA (2.76 × 10−[Citation5] M), this maximum contractile response was decreased by 60.98%, and the contractile response changed to 0.07 ± 0.02 g (). The maximum contractile response induced by CaCl2 in endothelium-denuded thoracic aorta rings was 1.40 ± 0.24 g, which was reduced by 60.00% after incubation with PA (2.76 × 10−5 M) incubation and the contractile response decreased to 0.56 ± 0.18 g ().
Figure 3. Effects of PA on calcium-induced vasoconstriction. Sustained contractile responses of 0.09 ± 0.02 g were induced by 60 mM KCl in intact thoracic aorta rings in K-H solution without Ca2+, and then CaCl2 (6.00 × 10−5 to 4.55 × 10−3 M) was cumulatively applied every 5 min. (A) Effect of PA on the cumulative contraction induced by influx of extracellular Ca2+ in intact thoracic aorta rings pre-contracted with KCl; (B) Effect of PA on contraction of endothelium-denuded aortic rings; (C) Effect of PA on the cumulative-contraction induced by influx of extracellular Ca2+ in intact thoracic aorta rings pre-contracted with PE; (D) Effect of PA on the cumulative-contraction induced by influx of extracellular Ca2+ in intact endothelium-denuded aorta rings pre-contracted with PE. Values are expressed as mean ± SD. *p < 0.05, **p < 0.01, ***p < 0.001 vs the Cacl2 alone group.
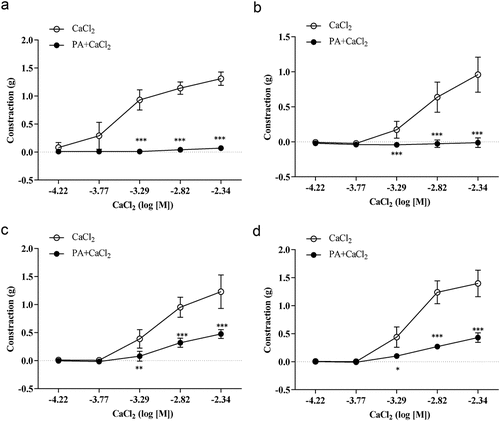
Discussion
Peripheral vascular resistance plays a fundamental role in the regulation of blood pressure. This resistance depends on vascular tone, which is the result of the balance between factors that influence vasoconstriction and vasodilation of smooth muscle cells. Excitatory stimuli increase calcium influx, which results in vascular smooth muscle contraction. However, vasodilation mainly relies on the endothelium. Once endothelial factors, such as NO, are stimulated and released, they reduce intracellular calcium levels and lead to relaxation of vascular smooth muscle [Citation25], also called vasodilation. Here, we explored the relaxant effect of PA with regard to endothelial function.
First of all, we found that PA at concentrations as low as 2.77 × 10−7 M had a vasorelaxant effect in intact rat thoracic aorta rings; however, this effect was significantly reduced after removal of the vascular endothelium. This reduction supports the idea that the endothelium plays an important role in the process by which PA exerts a vasodilative effect.
The mechanisms underlying both vasoconstriction and vasodilation are complicated, and they involve membrane receptors and ion channels. The vascular endothelium plays an important role in the control of vascular tone [Citation15], and it is a source of vasodilators, such as NO, prostacyclin, and other EDRFs [Citation26]. Therefore, to clarify whether the levels of EDRFs, such as NO and Prostaglandin I2 (PGI2), influence the impact of PA on vasodilation, we incubated thoracic aorta artery rings with L-NAME, an NOS inhibitor, and indomethacin, an inhibitor of COX [Citation27,Citation28]. Concentration-response curves to PA were shifted to the right side with decreases of Emax, which suggested that NO plays an important role in PA-induced vasodilation. With regard to PGI2, a COX metabolite, the relaxation induced by PA was significantly attenuated after treatment with indomethacin, which suggested that COX metabolites might be involved in the vasorelaxant response caused by PA. In short, EDRFs, in particular NO and PGI2, may play important roles in PA-induced vasodilation.
Multiple studies have demonstrated that NO-induced relaxation predominantly occurs through activation of soluble guanylyl cyclase in vascular smooth muscle, which leads to accumulation of cGMP and subsequent reduction of Ca2+ influx via cGMP-dependent protein kinase (PKG) activation [Citation29,Citation30]. Since we identified a role of NO in the vasodilative effect of PA, it was natural to further investigate the participation of the NO/cGMP pathway. We incubated preparations with ODQ, an inhibitor of NO-sensitive guanylyl cyclase. The results revealed that the vasorelaxant effects of PA were obviously decreased after ODQ administration, which implied that the NO/cGMP pathway may be involved in the relaxant response induced by PA.
Potassium channel activity may also be involved in vasodilatation via modulation of the cell membrane, inhibition of K+ outflow or blockage of voltage-dependent Ca2+ channels [Citation31,Citation32]. As mentioned above, calcium influx is one of the main factors responsible for vasoconstriction. If vasoconstriction is attenuated, conversely, vasodilation becomes relatively increased. Thus, we constructed an experiment from the perspective of vasoconstriction, mainly as mediated by calcium influx. The major finding of our work is that 2.76 × 10−5 M of PA inhibited CaCl2-induced vasoconstriction in both intact and denuded rat thoracic aorta rings, suggesting that Ca2+ may also contribute to the vasodilative effects of PA, in addition to the effects of the endothelium.
Conclusion
PA appears to be an effective vasodilator in isolated rat thoracic aorta. The endothelium-dependent NO-cGMP pathway, the prostacyclin pathway and reduction of calcium influx may synergistically contribute to PA-mediated vasorelaxation.
Author contributions
H.Y. and XY.L. designed the experiments. ZK.L. SC.W. and FR.Z. performed the experiments, analyzed data and developed the discussion. XY.L. and ZK.L. preparing the figures and wrote the main manuscript. XY.L. and JY. W. edited and proofread the article. HH.X. prepared Fіgures 1,2 and 3
Consent for publication
The authors declare that they all consent to publication.
Data availability of statement
The datasets generated during the current study are available from the corresponding author upon reasonable request.
Ethics approval and consent to participate
The experimental procedures were approved by the China Academy of Chinese Medical Science’s Administrative Panel on Laboratory Animal Care (No.20162002). All animal experiments were performed in accordance with institutional guidelines and ethics.
Additional information
Funding
References
- Xu Z, Wang X, Dai Y, et al. (+/-)-Praeruptorin A enantiomers exert distinct relaxant effects on isolated rat aorta rings dependent on endothelium and nitric oxide synthesis. Chem Biol Interact. 2010;186(2):239–246.
- Chen ZX, Huang BS, She QL, et al. The chemical constituents of Bai-Hua-Qian-Hu, the root of peucedanum praeruptorum Dunn. (Umbelliferae)–four new coumarins (author’s transl). Yao Xue Xue Bao. 1979;14(8):486–496.
- Zhao NC, Jin WB, Zhang XH, et al. Relaxant effects of pyranocoumarin compounds isolated from a Chinese medical plant, Bai-Hua Qian-Hu, on isolated rabbit tracheas and pulmonary arteries. Biol Pharm Bull. 1999;22(9):984–987.
- Song Y, Jing W, Yan R, et al. Research progress of the studies on the roots of peucedanum praeruptorum Dunn (peucedani radix). Pak J Pharm Sci. 2015;28(1):71–81.
- Yu CL, Yu YL, Yang SF, et al. Praeruptorin A reduces metastasis of human hepatocellular carcinoma cells by targeting ERK / MMP1 signaling pathway. Environ Toxicol. 2021;36(4):540–549.
- Yeon JT, Choi SW, Ryu BJ, et al. Praeruptorin A inhibits in vitro migration of preosteoclasts and in vivo bone erosion, possibly due to its potential to target calmodulin. J Nat Prod. 2015;78(4):776–782.
- Yeon JT, Kim KJ, Choi SW, et al. Anti-osteoclastogenic activity of praeruptorin A via inhibition of p38/Akt-c-Fos-NFATc1 signaling and PLCgamma-independent Ca2+ oscillation. PLoS One. 2014;9(2):e88974.
- Wu JY, Fong WF, Zhang JX, et al. Reversal of multidrug resistance in cancer cells by pyranocoumarins isolated from radix peucedani. Eur J Pharmacol. 2003;473(1):9–17.
- Ji Y, Rao MR. Effects of peucedanum praeruptorum extractum on blood pressure, left ventricular hypertrophy and hemodynamic changes in left ventricular hypertrophied hypertensive rats. Zhongguo Zhong Xi Yi Jie He Za Zhi. 1996;16(11):676–678.
- Xiang J, Zhang C, Di T, et al. Salvianolic acid B alleviates diabetic endothelial and mitochondrial dysfunction by down-regulating apoptosis and mitophagy of endothelial cells. Bioengineered. 2022;13(2):3486–3502.
- Walter U, Waldmann R, Nieberding M. Intracellular mechanism of action of vasodilators. Eur Heart J. 1988;9(Suppl H):1–6.
- Wu J, Zhang D, Hu L, et al. Paeoniflorin alleviates NG-nitro-L-arginine methyl ester (L-NAME)-induced gestational hypertension and upregulates silent information regulator 2 related enzyme 1 (SIRT1) to reduce H 2 O 2 -induced endothelial cell damage. Bioengineered. 2022;13(2):2248–2258.
- Kopincova J, Puzserova A, Bernatova I. L-NAME in the cardiovascular system - nitric oxide synthase activator? Pharmacol Rep. 2012;64(3):511–520.
- Gollasch M, Ried C, Bychkov R, et al. K + currents in human coronary artery vascular smooth muscle cells. Circ Res. 1996;78(4):676–688.
- Dannert MT, Alsasua A, Herradon E, et al. Vasorelaxant effect of Win 55,212-2 in rat aorta: new mechanisms involved. Vascul Pharmacol. 2007;46(1):16–23.
- Lucas S. The pharmacology of indomethacin. Headache. 2016;56(2):436–446.
- Zhang SL, Li JM, Xiao QH, et al. Effect of dl-praeruptorin A on ATP sensitive potassium channels in human cortical neurons. Acta Pharmacol Sin. 2001;22(9):813–816.
- Gan L, Wang ZH, Zhang H, et al. Endothelium-independent vasorelaxant effect of 20(S)-protopanaxadiol on isolated rat thoracic aorta. Acta Pharmacol Sin. 2016;37(12):1555–1562.
- Lopez-Canales JS, Lozano-Cuenca J, Muaoz-Islas E, et al. Mechanisms involved in the vasorelaxant effects produced by the acute application of amfepramone in vitro to rat aortic rings. Braz J Med Biol Res. 2015;48(6):537–544.
- Lozano-Cuenca J, Gonzalez-Hernandez A, Lopez-Canales OA, et al. Possible mechanisms involved in the vasorelaxant effect produced by clobenzorex in aortic segments of rats. Braz J Med Biol Res. 2017;50(9):e5765.
- Brawley L, Shaw AM, MacDonald A. Role of endothelium/nitric oxide in atypical beta-adrenoceptor-mediated relaxation in rat isolated aorta. Eur J Pharmacol. 2000;398(2):285–296.
- Baracat JS, Teixeira CE, Okuyama CE, et al. Relaxing effects induced by the soluble guanylyl cyclase stimulator BAY 41-2272 in human and rabbit corpus cavernosum. Eur J Pharmacol. 2003;477(2):163–169.
- Yan Y, Chen YC, Lin YH, et al. Brazilin isolated from the heartwood of Caesalpinia sappan L induces endothelium-dependent and -independent relaxation of rat aortic rings. Acta Pharmacol Sin. 2015;36(11):1318–1326.
- Lavergne C, Martinez M-J, Trottier C. Empirical model selection in generalized linear mixed effects models. Comput Stat. 2008;23(1):99–109.
- Colussi GL, Di Fabio A, Catena C, et al. Involvement of endothelium-dependent and-independent mechanisms in midazolam-induced vasodilation. Hypertens Res. 2011;34(8):929–934.
- Villar IC, Francis S, Webb A, et al. Novel aspects of endothelium-dependent regulation of vascular tone. Kidney Int. 2006;70(5):840–853.
- Friebe A, Koesling D. Regulation of nitric oxide-sensitive guanylyl cyclase. Circ Res. 2003;93(2):96–105.
- Moncada S, Higgs A, Higgs A. The L-arginine-nitric oxide pathway. N Engl J Med. 1993;329(27):2002–2012.
- Clark SG, Fuchs LC. Role of nitric oxide and Ca++-dependent K+ channels in mediating heterogeneous microvascular responses to acetylcholine in different vascular beds. J Pharmacol Exp Ther. 1997;282(3):1473–1479.
- Robertson BE, Schubert R, Hescheler J, et al. cGMP-dependent protein kinase activates Ca-activated K channels in cerebral artery smooth muscle cells. Am J Physiol. 1993;265(1):C299–303.
- Gollasch M, Hescheler J, Quayle JM, et al. Single calcium channel currents of arterial smooth muscle at physiological calcium concentrations. Am J Physiol. 1992;263(5):C948–52.
- Nelson MT, Quayle JM. Physiological roles and properties of potassium channels in arterial smooth muscle. Am J Physiol. 1995;268(4):C799–822.