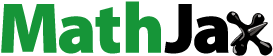
Abstract
A nanolaminated surface layer was produced on interstitial-free steel by surface mechanical rolling treatment. While the microstructure showed relatively high thermal stability during annealing at 500°C, significant grain boundary (GB) migration occurred in the nanolaminated surface layer during chromizing at the same temperature. GB diffusion of Cr was observed under the Harrison B-type regime in the chromized surface layer. The accelerated GB migration was discussed to be influenced by enhanced GB energy and mobility due to the GB diffusion and enrichment of Cr in nanolaminated structures. Meanwhile, the high stresses introduced during sample preparations also enhance the coarsening kinetics.
GRAPHICAL ABSTRACT
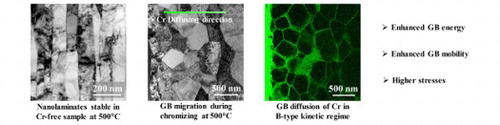
IMPACT STATEMENT
Different from enhanced thermal stability by regular GB segregation, GB enrichment of Cr due to fast GB diffusion from a Cr-coating can accelerate the GB migration in nanolaminated IF steel.
Introduction
Diffusion behavior of nanostructured materials has attracted a lot of studies in recent years, mostly due to its importance in controlling surface alloying and other diffusion-related processes [Citation1–3]. For example, the mean diffusivity in nanostructured Fe was detected to be 7–9 orders of magnitude higher than the bulk diffusivity, due to significantly enhanced diffusion along grain boundaries (GBs) produced by severe plastic deformation (SPD) [Citation1,Citation2,Citation4]. This benefited the surface alloying processes (such as nitriding and chromizing) of steels, so that they could be achieved at much lower temperatures relative to the conventional processes [Citation5,Citation6]. However, nanostructured materials typically tend to coarsen rapidly during annealing or even at room temperature, due to their inherent thermal instability related with a high fraction of GBs [Citation7]. This may decrease the enhancements in diffusion and other properties of nanostructured materials.
Some approaches have been developed to improve the thermal stability of nanostructured materials, for example by forming twin boundaries and low-angle boundaries and by introducing segregation or second phases at GBs [Citation8–11]. In the previous work [Citation12], a gradient nanolaminated (GNL) surface layer with a high thermal stability was produced on IF steel. Its refined microstructure and enhanced hardness kept stable at 500°C, which was much higher than the coarsening temperatures of equiaxed nanostructured samples. Diffusion measurements confirmed that the diffusivity of Cr in the GNL IF steel was still higher than the lattice diffusivity by ∼4 orders of magnitude at 500°C. The significantly enhanced thermal stability and Cr diffusion of the GNL IF steel might facilitate the preparation of steels with high mechanical and corrosion properties.
It is noticed that previous works mostly concentrated on the microstructure and thermal stability of nanostructured samples after isothermal annealing treatments without elemental diffusion [Citation2,Citation12–14], the samples directly after diffusion were seldom studied. As we know, the chemically diffused elements play an important role on the microstructure evolution of base materials by such effects as alloying or GB segregation. The diffusing elements may not only segregate at interfaces, but also change the GB structure inducing phase transitions [Citation15]. Therefore, studies on the microstructure and elemental distribution in nanostructured materials after diffusion are critical for the understanding of diffusion kinetics and resulted properties of them.
In this work, the microstructure and thermal stability of a GNL surface layer were compared on the IF steel samples with and without Cr diffusion. Distinct differences were observed and the underlying mechanisms were analyzed.
Materials and methods
Commercial Ti–Nb IF steel with composition (in wt.%) of 0.0024C, 0.0022N, 0.027Al, 0.01Si, 0.13Mn, 0.060Ti, 0.002Nb and balance Fe was used in this work. Cylindric samples in the annealed coarse-grained state were submitted to surface mechanical rolling treatment (SMRT) as described in Ref. [Citation12]. In this work, the rotation velocity of the sample was 300 rpm and the sliding velocity of the tool tip was 6 mm/min. The process was repeated 4 times with an increased processing depth (15 μm per time). After SMRT, a pure Cr layer of ∼300 nm in thickness was electroplated onto the treated surface. Subsequently, the as-SMRT (i.e. Cr-free) and Cr-coated samples were annealed together in vacuum at 500°C, which was close to the onset temperature of recrystallization [Citation12], so that a more remarkable difference due to mechano-chemical coupling was expected. At lower temperatures, Cr diffusion would be significantly impeded, while at higher temperatures a rapid microstructure evolution due to recrystallization would be expected.
Cross-sectional morphologies of the SMRT samples in different conditions were characterized by scanning electron microscopy (SEM, FEI Verios 460 instrument) and transmission electron microscopy (TEM, FEI Talos F200X instrument operated at 200 kV). Furthermore, high angle annular dark-field (HAADF) scanning TEM (STEM) imaging and energy disperse spectroscopy (EDS) mapping were performed on the F200X instrument equipped with a Super-X EDS detector. High-resolution STEM (HRSTEM) was examined on an FEI-Titan G2 microscope with double aberration (Cs) correctors operated at 300 kV.
Results and discussion
Clear evidences of plastic deformation can be observed in the surface layer of ∼400 μm in thickness on the SMRT sample, as shown in (a). The deformation degree increases with decreasing depth and significant microstructural refinement is achieved in the near-surface layer. Since previous diffusion measurements [Citation12] indicated that Cr diffused into the SMRT surface layer within a thickness <5 μm at 500°C for 720 min, the microstructure and Cr diffusion behavior are primarily concerned in the near-surface layer (see the inset in (a)) in this work. TEM observations further confirm that the surface layer is characterized by a typical lamellar structure with a high density of dislocations (see (b,c)). Most lamellar boundaries are long and straight, roughly paralleling to the treated surface. It is noticed that most boundaries in the SMRT surface layer are high-angle boundaries (HABs, see supplemental Figure S1). SEM and TEM observations in different samples substantiate a good reproducibility of such kind of microstructure in the near-surface regions after SMRT (also in samples under other conditions hereafter). Statistic measurements reveal that the average thickness of lamellae is ∼69 nm at the top surface.
Figure 1. (a) Cross-sectional SEM image of the SMRT sample, with a magnified view of the boxed region shown by the inset. Cross-sectional (b) bright- and (c) dark-field TEM images of the near-surface layer. The inset in (b) shows the corresponding selected-area electron diffraction pattern. The dashed line in (a) marks the SMRT surface.
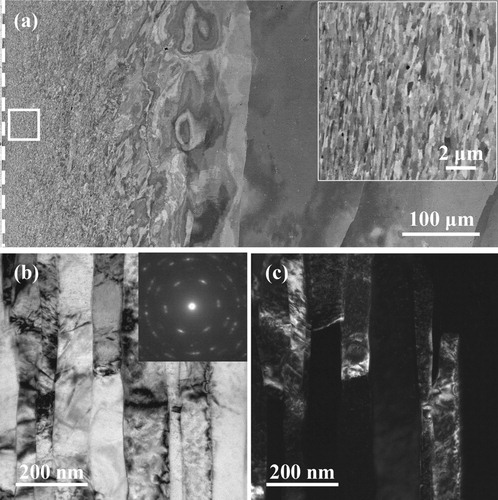
The high thermal stability of the nanolaminated IF steel has been demonstrated in Ref. [Citation12]. As shown in (a), the microstructure in the near-surface layer is still significantly refined after annealing at 500°C for 720 min. The mean lamellar thickness only increases to ∼88 nm, and the boundaries are still dominated by HABs (see supplemental Figure S1). In comparison, very different microstructure is observed in the chromized surface layer after the same annealing process. As shown in (b), grains in the near-surface layer develop into the equiaxed morphology with a mean size of ∼280 nm, also separated mostly by HABs (see Figure S1). With increasing depth, grains are prone to reveal comparable size and morphology with those in the annealed Cr-free sample (see supplemental Figure S2).
Figure 2. Cross-sectional TEM morphologies of the near-surface layers of (a) the Cr-free and (b) chromized SMRT samples treated at 500°C for 720 min. The SMRT surface is at the left side in both images. Arrows in (a) mark triple junctions (Y-junctions).
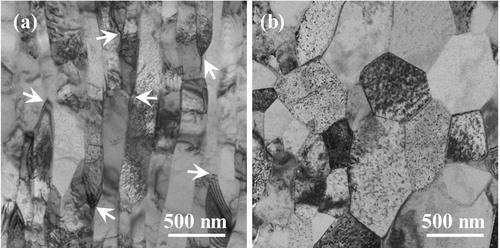
Furthermore, the time dependences of microstructure evolution are compared in the near-surface layers of the chromized- and Cr-free-SMRT samples annealed at 500°C. As shown in supplemental Figure S3, the mean structural size in the chromized sample is always larger than that in the Cr-free sample after the same time and follows approximately a parabolic time dependence. It is noticed that the apparent coarsening rate decreases gradually with time in the chromized samples, and the microstructure keeps relatively stable before 720 min and coarsens fast (possibly due to the onset of recrystallization) after that in the Cr-free samples.
The HAADF-STEM image and EDS mapping of Cr (see ) demonstrate that Cr atoms remarkably enrich at GBs in the chromized surface layer. Quantitative measurements reveal that the Cr concentration can reach ∼30 at.% at GBs adjoining the Cr-coating, and the mean concentration gradually decreases with increasing depth. Furthermore, it is noticed that the widths of the Cr-enriched zones vary around different GBs, and inhomogeneous Cr distributions may be resulted in some grains. As illustrated in (c), the widths of Cr-enriched zones along the lines B, C and D are about 210, 68 and 25 nm, respectively. While the Cr distributions are almost symmetric in both sides across the GB along the lines B and D, it is asymmetric along the line C.
Figure 3. Cross-sectional (a) HAADF-STEM image and (b) EDS Cr mapping in the surface layer of the SMRT sample chromized at 500°C for 720 min. (c) shows the Cr profiles across 3 different GBs as marked in (a), with corresponding zero positions indicated by green dashed lines.
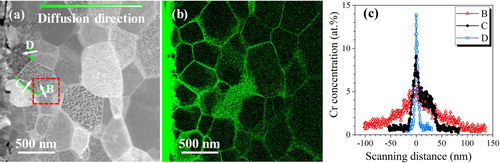
presents a detailed TEM image of solute diffusion in the B-type regime after Harrison’s classification [Citation16] in nanostructured metals (for the first time to the authors’ knowledge). Fast GB diffusion results in the formation of a Cr-enriched network enclosing ferrite grains. The mean diffusion coefficient in the nanolaminated surface layer is estimated to be ∼2.4 × 10−17 m2/s at 500°C for 720 min [Citation12]. Considering the diffusion distance is enlarged due to the zigzag contour of connecting GBs, the GB diffusivity should be higher than this value. In addition, out-diffusion from GBs into adjacent grains results in the formation of Cr-enriched zones around GBs, while the bulk diffusion is not negligible (∼21.7 nm, Dv is the extrapolated bulk diffusion coefficient from Ref. [Citation17] and t is the time). The inhomogeneous Cr distributions around different GBs might be related with different migration kinetics of them, as discussed later.
On annealing, lamellar structures in nanometer or sub-micron scale tend to coarsen and transform into equiaxed configurations [Citation18,Citation19]. However, the gradient nanostructured surface layers typically show higher thermal stability, mainly due to the lower excess free energy of GBs produced by shear deformation with high strain rates [Citation8,Citation10,Citation20]. For example, an enhanced thermal stability of nanograins below a critical grain size was discovered in gradient nanostructured Cu (and Ni), while the GB energy was only half of the conventional GB energy [Citation20]. In addition, numerous triple junctions formed by three lamellar boundaries (Y-junctions) might be pinned by interconnecting and lamellar boundaries (with a low curvature) in the nanolaminated surface layer, as illustrated in (a). This also results in an enhanced stability of the lamellar structure, because the retarded migration of Y-junctions slows down the recovery and recrystallization kinetics [Citation19].
While lamellar grains are rather stable in the Cr-free-SMRT sample and much coarser equiaxed grains form in the chromized-SMRT sample after the same annealing treatment at 500°C, the microstructural observations combining compositional analyses indicate that the significant microstructure evolution should be resulted from the Cr diffusion along GBs in the chromized sample. Thus, a diffusion-accelerated GB migration is suggested in the nanolaminated surface layer. The diffusion-related nature of the GB migration is also confirmed in chromized-SMRT samples at 500°C for shorter times. As shown in and supplemental Figure S4 (180 and 45 min, respectively), similar microstructure and Cr GB enriching are observed in the near-surface layers as in , but the mean grain sizes, Cr concentrations in GBs and diffusion depths are smaller than those in the latter, suggesting a simultaneous occurrence of diffusion and GB migration. The GB migration rate decreases gradually with increasing time (see Figure S3), possibly due to the decreasing Cr GB diffusion rate [Citation12,Citation18].
Figure 4. (a) A cross-sectional TEM morphology of the near-surface layer in the SMRT sample chromized at 500°C for 180 min. (b) HAADF-STEM image and (c) EDS Cr mapping of the boxed region in (a).
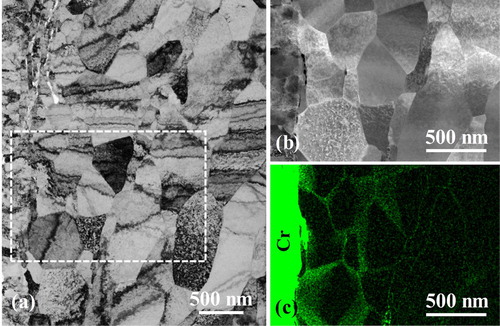
With diffusion and enrichment of Cr, the GB energy (γ) of ferrite will be changed according to [Citation21]
(1)
(1)
where γ0 is the GB energy of solvent materials, Γ is the concentration of solute atoms enriched at GBs, x is the bulk solute concentration, ΔHseg is the segregation enthalpy, R and T are gas constant and temperature, respectively. Taking x as the Cr concentration in the crystalline interiors near GB (∼1 at.%), one may estimate that the GB energy is enhanced to ∼0.99 J/m2 with ∼10 at.% Cr enriched in GBs, by using γ0 = 0.805 J/m2 and ΔHseg = 3.74 kJ/mol for conventional GBs in the Fe–Cr system [Citation22,Citation23]. While such an estimation is rough since the GB energy depends on various factors such as GB type and misorientation, enhanced GB energies due to Cr addition in a Σ3(112) GB have been confirmed in pure Fe and Fe0.98Cr0.02 alloy by first-principles simulation recently [Citation24]. Therefore, a higher driving force for GB migration is expected in the nanolaminated IF steel during chromizing. It is noted that the Cr-enrichment in GB is due to fast GB diffusion from the deposited Cr-coating in this work, being different from a regular GB segregation from adjacent grains. Typically, the GB energy is decreased in a regular case. However, it can be increased in our case, due to a high excess concentration of Cr and the term for entropic penalty of segregation as in Equation (1). Qualitatively, such behavior is confirmed with Ostwald rule of states, when the system transforms to a nearest stable state with a recovered microstructure in the severely deformed surface layer on the cost of increased GB energy due to Cr enrichment.
Meanwhile, the GB diffusion of Cr from the deposited source might also increase the mobilities of GBs and Y-junctions. At low temperatures when lattice diffusion was very small or completely frozen out but GB diffusion was remarkable, a distinct transverse shift of GBs, i.e. diffusion-induced GB migration (DIGM), occurred in some metallic systems [Citation25–27]. During DIGM, the GB migrates towards the adjacent grains, and the solute atoms initially diffused into the GB will be left behind the newly-formed parts of the growing grains. In the chromized surface layer, the widths of some Cr-enriched GB regions are much larger than bulk diffusion distances (see Figures and , Figure S4). Since the possibility that they are not observed edge-on (i.e. parallel to the beam direction) can partially be excluded by HRSTEM observations, the broadening of them should be resulted from DIGM. As shown in , a GB segment has migrated ∼110 nm from its initial position and formed a Cr-enriched region with a peak concentration of ∼5.8 at.%. Some moiré fringes and lattice distortions, possibly related to diffusion-induced stresses (or strains) which dominantly contribute to DIGM [Citation27], can be observed in this region. It is noted that the local stresses are also possibly due to unrelaxed ones induced by SMRT.
Figure 5. (a) HAADF-STEM image, (b) HRSTEM image and (c,d) magnified views (Fourier-filtered images) of the boxed regions in (b). The position of (a) is marked by a red dashed box in (a).
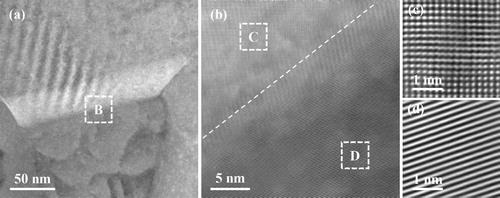
In addition to the enhanced GB energy and mobility by Cr diffusion, high stresses induced by sample preparations might also contribute to the accelerated GB migration in the nanolaminated surface layer. Typically, significant compressive residual stresses are introduced in sample surface layers by SMRT [Citation28]. The stress state will be further enhanced by the high tensile residual stress in the electroplated Cr-layer [Citation29], and to some extent by the expansion mismatch of IF steel and Cr-layer with different thermal expansion coefficients during heating. Thus, a very high stress and even plastic deformation will be achieved in the near-surface region of the chromized sample, as indicated by the formation of some micro-cracks around the coating/substrate interface (see Figures and ). In fact, the microstructure has been already coarsened slightly in the near-surface region of SMRT samples after Cr-electroplating (see supplemental Figure S5), similar with the deformation-induced grain growth in nanostructured metals under compression at room temperature [Citation30]. Therefore, GB migration might be further promoted in nanolaminates due to the high stresses and increased curvature of boundaries, as indicated by that larger grains are formed in deeper sub-surface layers (with less or no Cr, see Figures and , Figure S4) of the chromized samples than in the Cr-free samples after annealing. Meanwhile, a slightly smaller grain size is noticed in Cr-diffused layers than in deeper sub-surface layers in the chromized samples, suggesting a solute drag effect on GB mobility in the Cr-enriched regions.
In this letter, solute distribution as a result of GB diffusion under the B-type kinetic regime was experimentally observed in the chromized nanolaminated surface layer. While a high thermal stability was confirmed in the Cr-free samples at 500°C, a coarser and more equiaxed microstructure was obtained in the Cr-coated samples after the same annealing treatment. The accelerated GB migration was discussed to be induced by enhanced GB energy and mobility due to fast GB diffusion and enrichment of Cr in the severely deformed surface layer from the Cr-coating, in addition to by the high stresses introduced during sample preparations. Further experiments, such as in-situ observations by HRSTEM and EDS, are expected to provide direct evidences relating microstructure evolution and Cr diffusion (or other contributions).
Supplemental Material
Download MS Word (1.7 MB)Acknowledgements
The authors are grateful to Profs. K. Lu and Y. T. Zhu for constructive discussions and to Dr. X. C. Liu for helps with HRSTEM.
Disclosure statement
No potential conflict of interest was reported by the author(s).
Additional information
Funding
References
- Valiev RZ, Islamgaliev RK, Alexandrov IV. Bulk nanostructured materials from severe plastic deformation. Prog Mater Sci. 2000;45(2):103–189. doi: 10.1016/S0079-6425(99)00007-9
- Wang ZB, Lu K. Diffusion and surface alloying of gradient nanostructured metals. Beilstein J Nanotechnol. 2017;8:547–560. doi: 10.3762/bjnano.8.59
- Divinski SV. Grain boundary diffusion in severe plastically deformed metals: state of the art and unresolved issues. Diffus Found. 2015;5:57–73. doi: 10.4028/www.scientific.net/DF.5.57
- Sauvage X, Wilde G, Divinski SV, et al. Grain boundaries in ultrafine grained materials processed by severe plastic deformation and related phenomena. Mater Sci Eng A. 2012;540:1–12. doi: 10.1016/j.msea.2012.01.080
- Tong WP, Tao NR, Wang ZB, et al. Nitriding iron at lower temperatures. Science. 2003;299(5607):686–688. doi: 10.1126/science.1080216
- Lu SD, Wang ZB, Lu K. Enhanced chromizing kinetics of tool steel by means of surface mechanical attrition treatment. Mater Sci Eng A. 2010;527(4–5):995–1002. doi: 10.1016/j.msea.2009.10.030
- Ames M, Markmann J, Karos R, et al. Unraveling the nature of room temperature grain growth in nanocrystalline materials. Acta Mater. 2008;56(16):4255–4266. doi: 10.1016/j.actamat.2008.04.051
- Lu K. Stabilizing nanostructures in metals using grain and twin boundary architectures. Nat Rev Mater. 2016;1:16019. doi: 10.1038/natrevmats.2016.19
- Chookajorn T, Murdoch HA, Schuh CA. Design of stable nanocrystalline alloys. Science. 2012;337(6097):951–954. doi: 10.1126/science.1224737
- Liu XC, Zhang HW, Lu K. Strain-induced ultrahard and ultrastable nanolaminated structure in nickel. Science. 2013;342(6156):337–340. doi: 10.1126/science.1242578
- Zhang X, Misra A. Superior thermal stability of coherent twin boundaries in nanotwinned metals. Scr Mater. 2012;66(11):860–865. doi: 10.1016/j.scriptamat.2012.01.026
- Xie SL, Wang ZB, Lu K. Diffusion behavior of Cr in gradient nanolaminated surface layer on an interstitial-free steel. J Mater Sci Technol. 2019;35(3):460–464. doi: 10.1016/j.jmst.2018.09.043
- Ribbe J, Schmitz G, Gunderov D, et al. Effect of annealing on percolating porosity in ultrafine-grained copper produced by equal channel angular pressing. Acta Mater. 2013;61(14):5477–5486. doi: 10.1016/j.actamat.2013.05.036
- Wang ZB, Divinski SV, Luo ZP, et al. Revealing interfacial diffusion kinetics in ultra-fine-laminated Ni with low-angle grain boundaries. Mater Res Lett. 2017;5(8):577–583. doi: 10.1080/21663831.2017.1368036
- Frolov T, Divinski SV, Asta M, et al. Effect of interface phase transformations on diffusion and segregation in high-angle grain boundaries. Phys Rev Lett. 2013;110(25):5. doi: 10.1103/PhysRevLett.110.255502
- Harrison LG. Influence of dislocations on diffusion kinetics in solids with particular reference to alkali halides. Trans Faraday Soc. 1961;57(8):1191–2001. doi: 10.1039/tf9615701191
- Bowen AW, Leak GM. Solute diffusion in alpha-iron and gamma-iron. Metall Trans. 1970;1(6):1695–1700. doi: 10.1007/BF02642019
- Yu T, Hansen N. Coarsening kinetics of fine-scale microstructures in deformed materials. Acta Mater. 2016;120:40–45. doi: 10.1016/j.actamat.2016.08.032
- Yu T, Hughes DA, Hansen N, et al. In situ observation of triple junction motion during recovery of heavily deformed aluminum. Acta Mater. 2015;86:269–278. doi: 10.1016/j.actamat.2014.12.014
- Zhou X, Li XY, Lu K. Enhanced thermal stability of nanograined metals below a critical grain size. Science. 2018;360(6388):526–530. doi: 10.1126/science.aar6941
- Weissmüller J. Alloy effects in nanostructures. Nanostr Mater. 1993;3:261–272. doi: 10.1016/0965-9773(93)90088-S
- Murdoch HA, Schuh CA. Estimation of grain boundary segregation enthalpy and its role in stable nanocrystalline alloy design. J Mater Res. 2013;28(16):2154–2163. doi: 10.1557/jmr.2013.211
- Saber M, Kotan H, Koch CC, et al. Thermodynamic stabilization of nanocrystalline binary alloys. J Appl Phys. 2013;113(6):63515-1–10. doi: 10.1063/1.4791704
- Yang Y, Schönecker S, Li W, et al. First-principles study of the Σ3(112) grain boundary in Fe-rich Fe-Cr alloys. Scr Mater. 2020;181:140–143. doi: 10.1016/j.scriptamat.2020.02.029
- Hillert M, Purdy GR. Chemically induced grain boundary migration. Acta Metall. 1978;26:333–340. doi: 10.1016/0001-6160(78)90132-3
- Cahn JW, Pan JD, Balluffi RW. Diffusion induced grain boundary migration. Scr Metall. 1979;13(6):503–509. doi: 10.1016/0036-9748(79)90078-4
- Beke DL, Kaganovskii Y, Katona GL. Interdiffusion along grain boundaries—diffusion induced grain boundary migration, low temperature homogenization and reactions in nanostructured thin films. Prog Mater Sci. 2018;98:625–674. doi: 10.1016/j.pmatsci.2018.07.001
- Huang HW, Wang ZB, Lu J, et al. Fatigue behavior of AISI 316L stainless steel with a gradient nanostructured surface layer. Acta Mater. 2015;87:150–160. doi: 10.1016/j.actamat.2014.12.057
- Pina J, Dias A, François M, et al. Residual stresses and crystallographic texture in hard-chromium electroplated coatings. Surf Coat Techn. 1997;96(2):148–162. doi: 10.1016/S0257-8972(97)00075-3
- Brandstetter S, Zhang K, Escuadro A, et al. Grain coarsening during compression of bulk nanocrystalline nickel and copper. Scr Mater. 2008;58:61–64. doi: 10.1016/j.scriptamat.2007.08.042