Abstract
Thermal stabilities of relaxor-PbTiO3 single crystals are of great importance due to their limited operational temperatures in devices. Herein, to elucidate how poling conditions influence thermal stabilities, we investigated direct current (DC) and alternating current (AC) poled Pb(Mg1/3Nb2/3)O3-0.29PbTiO3 (PMN-29PT) crystals grown by solid-state crystal growth, which guarantees more compositional uniformity. Our experiments show that the dielectric permittivity of the DC and AC poled crystals are 3560 and 4970, respectively, after annealing at 99.6°C, implying AC-poling prevails in enhancing thermal stabilities of relaxor-PbTiO3 single crystals. The mechanism behind the dissimilar thermal stabilities can be attributed to different domain configurations.
GRAPHICAL ABSTRACT
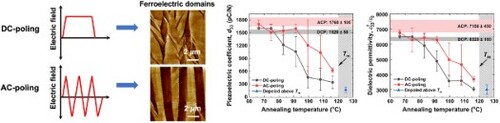
IMPACT STATEMENT
Alternating-current poling is of useful for not only enhancing piezoelectric and dielectric properties but also improving the thermal stabilities of relaxor-PbTiO3 single crystals.
1. Introduction
Piezoelectric materials that convert mechanical energy into electrical energy and vice versa have been widely used as actuators and transducers for plenty of electromechanical devices [Citation1,Citation2]. Specifically, the developments of relaxor-PbTiO3 (PT) single crystals, e.g. Pb(Zn1/3Nb2/3)O3-PbTiO3 (PZN-PT) and Pb(Mg1/3-Nb2/3)O3-PbTiO3 (PMN-PT), have achieved splendid performances for state-of-the-art applications [Citation3,Citation4]. With the growth of Sm-doped PMN-PT single crystals, piezoelectric materials have been enhanced to obtain large electric field-induced responses [Citation5]. However, fatal drawbacks, i.e. deteriorated stabilities against external stimuli such as temperatures and electric field, arise in spite of their exceptionally high piezoelectric and dielectric properties at a room temperature. As a result, many researchers have improved the operational temperature (so-called depolarization temperature, Td) of relaxor-PT single crystals by either designing ternary solid-solution systems or incorporating dopants into single crystals [Citation6,Citation7]. Nevertheless, the trade-off between enhancing piezoelectricity and improving thermal stabilities has hampered their versatile applications [Citation8–10]. Therefore, to meet increasing demands on relaxor-PT single crystals, feasible alternatives for enhancing both piezoelectricity and thermal stability should be studied.
High piezoelectricity of ferroelectric-based piezoelectric materials is only active when ferroelectric domains are aligned by applying electric fields (poling) [Citation11,Citation12]. Otherwise, ferroelectric domains tend to be randomly oriented and thus cancel out net macroscopic piezoelectricity. However, it is well accepted that poled domain configurations are rearranged when a temperature passes through phase transformation temperatures (depoling) [Citation13,Citation14]. That is why thermal stability is just as significant as the improvement of piezoelectric and dielectric properties, especially for practical applications, given that self-heating under operations is unavoidable and depolarizes crystals, thus causing malfunction in fabricated devices [Citation15].
So far, in the case of relaxor-PT single crystals, it is commonly accepted that rhombohedral-to-tetragonal transformation temperatures (TR-T) are considered to be equivalent to Td [Citation13,Citation14]. However, it should be noted that a discrepancy between a phase transformation temperature obtained from temperature-dependent permittivity and actual Td deserves more investigation. Anton et al. claimed that the inflection points of the real part of permittivity, or the peaks in dielectric loss, are fairly deviated from Td determined by a rapid drop in remanent polarization [Citation16]. In fact, an electric current associated with the depolarization of relaxor-PT single crystals is actually detected away from their phase transformation temperature [Citation17]. In addition, the difference between these temperatures is considerably dependent on the type of long-ranged ferroelectric order, and even electric fields applied in a poling direction shift the depolarization temperature of piezoelectric materials due to changes in crystallographic symmetries [Citation18]. On the basis of these supportive references, Td should be originated from complex reasons in that depolarization is highly correlated with long-ranged ferroelectric order or macroscopic domain configurations [Citation19]. Therefore, in order to explore correlations between domain structures and thermal stabilities, we adopted a domain engineering approach to overcome the aforementioned reliability issues.
Since demonstrations by Yamamoto et al andYamashita et al. [Citation20,Citation21], alternating current (AC) poling as a domain engineering method has been actively featured owing to its further enhanced piezoelectric and dielectric properties compared to its conventional direct current (DC) counterpart. It is generally accepted that the piezoelectric and dielectric properties of relaxor-PT single crystals can be improved from 20 to 100% by performing AC-poling [Citation22]. In the case of AC-poled PMN-PT single crystals, their piezoelectric and dielectric permittivity are enhanced to 2800 pC/N (+80%) and 8550 (+55%), respectively, when compared to their optimized DC-poled properties [Citation20,Citation21]. So far, conditions for AC-poling have been considerably optimized with respect to various parameters [Citation23–34]. Even though the domain size dependence of piezoelectricity associated with AC-poling still remains unsolved [Citation35], its origins have been attributed to the formation of stripe-like 109° domains parallel to electrodes [Citation28,Citation36]. Given that DC-poling leads to the formation of both 71° and 109° domains consisting of crystallographic rhombohedral phases [Citation36], the depolarization behaviors of DC-poled crystals should be dissimilar to that of AC-poled crystals in which only 109° domains comprised of mixed rhombohedral and monoclinic phases exist [Citation28]. Such argument was well manifested by a thermally stimulated depolarization current (TSDC) which is an insightful technique to track the presence of ferroelectric symmetries [Citation37]. However, how piezoelectric and dielectric properties of AC-poled crystals are really degraded by thermal annealing has not been studied yet. In this work, we present comparative experiments with the aim of showing how the properties of DC- and AC-poled crystals grown by a solid-state crystal growth (SSCG) method persist against annealing temperatures. Compared to the DC-poled crystals, the AC-poled crystals possess a better thermal stability given that the averaged ϵT33/ϵ0 of the AC-poled crystals (4970) is 1.4 times higher than that of the DC-poled crystals (3560) after annealing at 99.6°C. A feasible explanation for the dissimilar thermal stabilities is differences in domain configurations confirmed by a piezoresponse force microscopy (PFM). It is commonly accepted that domain configurations are highly correlated with thermal stabilities of ferroelectric materials [Citation19,Citation30,Citation38,Citation39]. In this regard, non-uniform domain configurations of DC-poled crystals (71° + 109° domains) likely have worse thermal stabilities than uniform domain configurations of AC-poled crystals (109° domains) due to their more randomly distributed depolarization fields originated from an incomplete compensation of bound charges at domain walls [Citation40]. We suggest that not only phase transformation temperatures but also domain configurations may contribute to the thermal stabilities of relaxor-PT single crystals. We hope this work gives insight towards understanding correlations between domain structures and thermal stabilities.
2. Experimental methods
(001)- oriented Pb(Mg1/3Nb2/3)O3-0.29PbTiO3 (PMN-29PT) single crystals were grown by a SSCG method by Ceracomp Co. Ltd. (Cheonan, Republic of Korea). The detail growth method can be found in the following literature [Citation41]. To ensure repeatability, at least 4 different single crystals were prepared and tested for each annealing temperature. The crystals were cut into the kt mode (3 × 3 × 0.205t mm3) with electrodes sputtered on the (001) planes of the single crystals to measure electrical properties. Poling was performed in a silicon oil-bathed customized fixture at a room temperature to avoid electrical breakdown or an electric shortcut. Before the poling, the crystals were always annealed at 250°C for 15 min to eliminate any previous poling states. The DC-poling was conducted by applying electric field of 10 kV/cm for 300 s. The AC-poling was performed using bi-polar triangle signals generated and amplified by a function generator (Agilent 33250A, Santa Clara, CA, USA) and a high-voltage amplifier (Trek, 609B, New York, NY, USA), respectively. A peak-to-peak electric field of 20 kVpp/cm, energetically equivalent to 5.87 kVrms/cm, was induced with 20 cycles at 1 Hz. As depicted in Figure , the annealing (depoling) processes were performed within a precise temperature controller (LTS420, Linkam, UK). A temperature was elevated up to annealing temperatures (T = 66.4, 74.7, 83.0, 91.3, 99.6, 107.9, 116.2, and 124.5°C) with a heating rate of 3°C/min and then maintained for 600 s. After the heating, cooling down to room temperature was performed inside the temperature controller before measurements. During the cooling, a heating element was immediately turned off without opening the stage. After cooling down to 25°C, piezoelectric coefficient (d33) and free dielectric permittivity (ϵT33/ϵ0) were obtained by a quasi-static piezo d33 meter (Model ZJ-4B, Chinese Academy of Science, China) and multi-frequency LCR meter (Agilent 4294A, Santa Clara, CA, USA) at 1 kHz, respectively. The impedance spectra of kt mode (8.5 MHz to 11.5 MHz) were also measured using the same LCR meter at a room temperature. Dielectric spectra were measured as a function of temperatures using a customized furnace with an impedance analyzer (HP4192A, Agilent, USA). To characterize the domain configurations of the crystals after the poling, their freshly fractured (010) surfaces of the crystals were scanned using a piezoresponse force microscopy (PFM) (Dimension Icon, Bruker, Santa Barbara, CA, USA).
3. Results and discussion
A readily-conducted approach to evaluating the depolarization temperature of given crystals has been the identification of phase transformation temperatures from temperature-dependent dielectric permittivity. To determine phase transformation temperatures, the dielectric permittivity and loss of DC- and AC- poled PMN-PT single crystals were measured as a function of temperatures (Figure ). Here, only one peak (81.4°C) was observed in the case of the DC-poled crystals before their Tm (120.1°C), at which the maximum permittivity was detected. On the other hand, two peaks can be discerned at 71.3°C and 77.9°C before their Tm (121.0°C) for the AC-poled crystals. In contrast to the similarity in their Tm, the AC-poled crystals exhibit relatively sharper peaks than the DC-poled crystals wherein the plateau-like peak was found. On the basis of publications regarding the phase diagram [Citation42] and structural analyses [Citation28,Citation43] of PMN-PT single crystals, their thermal evolution are investigated. It is commonly accepted that AC-poling partially induces a monoclinic phase (M) from an initial rhombohedral phase (R) [Citation28,Citation43]. Therefore, its crystallographic symmetry at the room temperature should be M + R. At the first peak (71.3°C), some tetragonal phases begin to evolve from the initial phase coexistence state (M + R → M + R + T) [Citation28]. Then, all ferroelectric phases are completely transformed into tetragonal phases at the second peak (77.9°C). The last peak corresponds to Tm at which all ferroelectric phases tend to be transformed to paraelectric phases (cubic). On the other hand, the initial rhombohedral phases of the DC-poled crystals are transformed to tetragonal phases and cubic phases at the first (81.4°C) and second (120.1°C) peak, respectively [Citation28,Citation43]. These phase transformation temperatures in the temperature-dependent dielectric permittivity have been recognized as their depolarization temperatures according to the conventional idea. In this sense, the depolarization temperature of the AC-poled crystals, at least, is considered to be lower than that of the DC-poled samples, which makes AC-poling worse than DC-poling in terms of thermal stability.
Figure 2. Temperature-dependent dielectric spectra of (a) DC-poled and (b) AC-poled PMN-PT single crystals with respect to measurement frequencies.
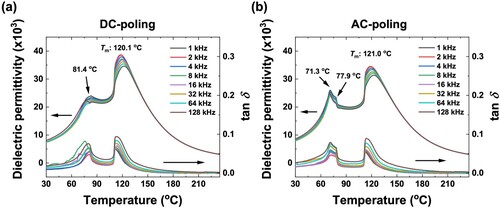
To verify the commonly-accepted belief above, actual thermal stabilities were studied by investigating how annealing temperatures indeed impact the properties of the crystals. We applied electric fields to the crystals (poling) and then annealed at specific temperatures (depoling) to evaluate degraded piezoelectric and dielectric properties, which is certainly indicative of how crystals are really depoled. Figure shows the piezoelectric coefficient and dielectric permittivity measured at 25°C of the annealed crystals. To assess how the properties vary as a function of the annealing temperatures, the piezoelectric coefficient and dielectric permittivity of the DC and AC poled crystals were optimized as a reference point before annealing crystals. The optimized properties of DC-poled crystals were determined when properties were saturated as poling electric field and time increase (10 kV/cm for 300 s). For AC-poled crystals, the optimized properties were achieved when enhancements by AC-poling were fully saturated. Given that property enhancements are proportional to cycle numbers [Citation28], optimized properties were realized with 20 cycles of peak-to-peak 20 kV/cm at 1 Hz. Compared to the DC-poled counterparts, the optimum piezoelectric coefficient and dielectric permittivity of the AC-poled crystals were enhanced to 1760 pC/N (+ 15.8%) and 7150 (+ 13.1%), respectively. The initial properties after the poling are denoted in the upper side of the figures for better comparisons. Though a slight drop in the properties of the AC-poled crystals was detected at 74.7°C near which the phase transformations happen as shown in Figure (b), their properties were not fully degraded up to 91.3°C, indicating that depolarizations did not arise significantly. However, it should be noted that substantial decreases in the properties of the AC-poled crystals were rapidly initiated at 99.6°C, while the properties of the DC-poled crystals began to be partially degraded at 83.0°C. Before annealing temperatures above Tm, the properties of the DC-poled crystals annealed at 99.6°C were already close to the completely depoled properties which indicate the properties obtained at annealing temperatures above Tm, whereas the averaged properties of the AC-poled crystals were not fully deteriorated up to 116.2°C. After annealing at temperatures above Tm, both crystals were completely depoled in that resonance and anti-resonance frequencies in the impedance spectra had totally vanished (Figure ).
Figure 3. Variations in the (a) piezoelectric coefficient and (b) dielectric permittivity of DC and AC-poled PMN-PT single crystals as a function of annealing (depoling) temperatures.
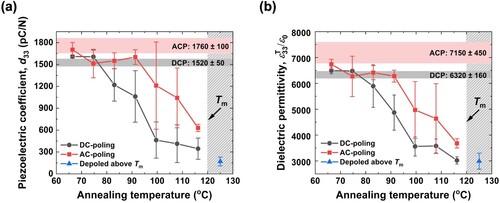
Figure 4. Impedance spectra of the (a) DC-poled and (b) AC-poled PMN-PT single crystals near their fundamental thickness mode with respect to the annealing temperatures.
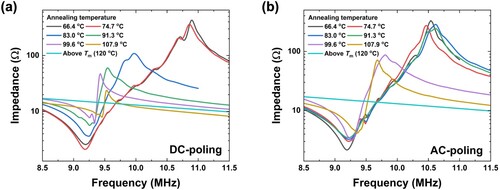
Consequently, the AC-poled crystals certainly possess better thermal stabilities in comparison with the DC-poled crystals. It draws us to the initial question of how actual operational temperatures are indeed deviated from phase transformation temperatures. It is interesting to note the actual degradations of the DC-poled crystals were initiated at 83.0°C, which is close to the phase transformation temperature (81.4°C) of the temperature-dependent permittivity (Figure (a)). It is well-consistent with the common belief in the case of the DC-poled crystals. However, a notable discrepancy was found for the AC-poled crystals: the temperature at which the substantial degradations in the properties occurred (91.3–99.6°C) is much higher than the phase transformation temperatures, i.e. 71.3°C or 77.9°C, though the partial deterioration was also detected at these temperatures. It implies other factors including domain configurations likely influence the thermal stabilities of relaxor-PT single crystals.
How poled crystals are truly depolarized can be estimated by thickness vibration modes (kt), which are certainly relevant to the degree of electromechanical coupling. Figure presents the impedance spectra of the DC and AC-poled crystals in the vicinity of their fundamental kt mode represented by resonance and anti-resonance frequencies. Consistent with the previous plots, the kt mode of the DC-poled crystals gradually diminished and almost disappeared when the annealing temperature passed through Tm. On the other hand, no distinctive variations in the impedance profiles were observed up to the annealing temperature of 91.3°C for the AC-poled crystals, indicating the preservation of their domain configurations to some extent. Subsequently, depoling was rapidly initiated between 91.3°C and 99.6°C. This phenomenon also indicates that the thermal stability of the AC-poled crystals is better than that of the DC-poled crystals.
On the basis of the results above, it is noted that the thermal stabilities of DC- and AC-poled crystals are dissimilar. Being supported by the depolarization current results of the following literature [Citation37], DC-poled crystals show thermal depolarization over a relatively wider range of temperatures than AC-poled crystals in that the gradual losses of the piezoelectric and dielectric properties emanated. Given that such step-like depolarization is believed to be relevant to the coexistence of multiple phases or domains [Citation18,Citation44,Citation45], the coexistence of 71° and 109° domains in DC-poled crystals well supports these claims. On the other hand, it is generally accepted that the domains of AC-poled samples are only composed of periodically-aligned 109° domains parallel to their electrode. This is why the sharper depolarization current and substantial loss of piezoelectric and dielectric properties emanated for the AC-poled crystals [Citation37]. To support the assumptions above, the PFM was used to observe the domain patterns of ferroelectric materials. The contrast in PFM images is associated with how domains are distributed in terms of their polarization direction. Figure provides optical microscopy images and their PFM results of fractured surfaces (010) for the DC- and AC-poled crystals. It is interesting to note that the stripe-like domains of the AC-poled crystals can be apparently discerned even in the optical microscopy image, whereas the non-uniform morphologies containing 71° and 109° domains were observed in the case of the DC-poled crystals. It is well consistent with our assumption, i.e. the single type of uniform domains is responsible for the rapider depolarization behaviors of the AC-poled crystals. On the other hand, the broader features of the DC-poled crystals are likely attributed to the non-uniformity of 71° and 109° domains. Furthermore, one can expect that non-uniform domain configurations have worse thermal stabilities due to their more randomly distributed depolarization fields coming from an incomplete compensation of bound charges at domain walls [Citation31]. Such argument is well supported by the fact that the thermal stability of a short-ranged relaxor state in non-ergodic relaxor are lower than that of a long-ranged field-induced ferroelectric state [Citation10]. Therefore, it should be noted that not only phase transformation temperatures but also domain configurations are highly associated with the thermal stability of relaxor-PT single crystals. We also investigated the thermal stabilities of crystals prepared by other growth techniques. Except for crystals grown by a continuous-feeding Bridgman method (CF-BM) which guarantees more compositional uniformity [Citation46], most crystals did not always show similar results obtained in this work. We carefully deduce that compositional uniformity should be at least assured to obtain dissimilar thermal stabilities depending on poling conditions. Further studies are required to verify our hypothesis.
4. Conclusions
To revisit how the actual operational temperature of relaxor-PT crystals is truly deviated from symmetry-changing transitions in temperature-dependent permittivity, we conducted the comparative experiments on the thermal stability of the DC- and AC-poled crystals grown by the SSCG method where compositional uniformity is more guaranteed. With the measured impedance spectra of the thickness mode samples, we found that the AC-poled crystals have better thermal stabilities than the DC-poled crystals, though the commonly accepted operational temperature of the AC-poled crystals is less than that of the DC-poled crystals. The dielectric permittivity of the AC-poled crystals (4,970) is 1.4 times higher than that of the DC-poled crystals (3,560) after annealing at 99.6°C. Given that the dielectric permittivity of unpoled crystals is 2,990, AC-poling has an advantage in improving the thermal stabilities of relaxor-PT single crystals. We believe that such dissimilar thermal stabilities are originated from not only phase transformation temperatures but also domain configurations.
Disclosure statement
No potential conflict of interest was reported by the author(s).
Additional information
Funding
References
- Zhang S, Li F, Jiang X, et al. Advantages and challenges of relaxor-PbTiO3 ferroelectric crystals for electroacoustic transducers – a review. Prog Mater Sci. 2015;68:1–66.
- Rödel J, Webber KG, Dittmer R, et al. Transferring lead-free piezoelectric ceramics into application. J Eur Ceram Soc. 2015;35:1659–1681.
- Park SE, Shrout TR. Ultrahigh strain and piezoelectric behavior in relaxor based ferroelectric single crystals. J Appl Phys. 1997;82:1804–1811.
- Shrout TR, Chang ZP, Kim N, et al. Dielectric behavior of single crystals near the (1−x) Pb(Mg1/3Nb2/3)O3-(x) PbTiO3 morphotropic phase boundary. Ferroelectr Lett Sect. 1990;12:63–69.
- Li F, Cabral MJ, Xu B, et al. Giant piezoelectricity of Sm-doped Pb(Mg1/3Nb2/3)O3-PbTiO3 single crystals. Science. 2019;364:264–268.
- Zhang S, Li F, Yu F, et al. Recent developments in piezoelectric crystals. J Korean Ceram Soc. 2018;55:419–439.
- Yamashita Y, Karaki T, Lee H-Y, et al. A review of lead perovskite piezoelectric single crystals and their medical transducers application. IEEE Trans Ultrason Ferroelectr Freq Control. 2022;69:3048–3056.
- Zhang S, Shrout TR. Relaxor-PT single crystals: observations and developments. IEEE Trans Ultrason Ferroelectr Freq Control. 2010;57:2138–2146.
- Zhang S, Li F. High performance ferroelectric relaxor-PbTiO3 single crystals: status and perspective. J Appl Phys. 2012;111:031301.
- Kim H-P, Ahn CW, Hwang Y, et al. Strategies of a potential importance, making lead-free piezoceramics truly alternative to PZTs. J Korean Ceram Soc. 2017;54:86–95.
- Damjanovic D. Ferroelectric, dielectric and piezoelectric properties of ferroelectric thin films and ceramics. Rep Prog Phys. 1998;61:1267.
- Su S, Zuo R, Lu S, et al. Poling dependence and stability of piezoelectric properties of Ba(Zr0.2Ti0.8)O3-(Ba0.7Ca0.3)TiO3 ceramics with huge piezoelectric coefficients. Curr Appl Phys. 2011;11:S120–S123.
- Chang WS, Lim LC, Yang P, et al. Rhombohedral-to-tetragonal phase transformation and thermal depolarization in relaxor-based ferroelectric single crystal. Appl Phys Lett. 2008;93:082903.
- Hosono Y, Harada K, Kobayashi T, et al. Temperature dependence of dielectric and piezoelectric properties of Pb(Zn1/3Nb2/3)O3–PbTiO3 piezoelectric single crystals. Jpn J Appl Phys. 2002;41:3808.
- Senousy MS, Rajapakse RKND, Mumford D, et al. Self-heat generation in piezoelectric stack actuators used in fuel injectors. Smart Mater Struct. 2009;18:045008.
- Anton E-M, Jo W, Damjanovic D, et al. Determination of depolarization temperature of (Bi1/2Na1/2)TiO3-based lead-free piezoceramics. J Appl Phys. 2011;110:094108.
- Lee G-J, Kim MC, Lee S-G, et al. Less than mm2 macrosymmetry in polarized (011)-oriented relaxor-PbTiO3 single crystals reflected in the face shear properties. Appl Phys Lett. 2022;120:042903.
- Sapper E, Schaab S, Jo W, et al. Influence of electric fields on the depolarization temperature of Mn-doped(1-x)Bi1/2Na1/2TiO3-xBaTiO3. J Appl Phys. 2012;111:014105.
- Li C-B-W, Thong H-C, Liu Y-X, et al. Thermally induced domain reconfiguration in ferroelectric alkaline niobate. Adv Funct Mater. 2022;32:2204421.
- Yamashita Y, Yamamoto N, Hosono Y, et al. Piezoelectric transducer, ultrasonic probe, and piezoelectric transducer manufacturing method. US Patent. 2015/0372219 A1. 2015.
- Yamamoto N, Yamashita Y, Hosono Y, et al. Ultrasonic probe, piezoelectric transducer, method of manufacturing ultrasonic probe, and method of manufacturing piezoelectric transducer. US Patent. 2014/0062261 A1. 2014.
- Sun Y, Karaki T, Yamashita Y. Recent progress on AC poling of relaxor-PbTiO3 ferroelectric single crystals: a review. Jpn J Appl Phys. 2022;61:SB0802.
- Wan H, Luo C, Chang W-Y, et al. Effect of poling temperature on piezoelectric and dielectric properties of 0.7Pb(Mg1/3Nb2/3)O3-0.3PbTiO3 single crystals under alternating current poling. Appl Phys Lett. 2019;114:172901.
- Kim H-P, Wan H, Lu X, et al. Scaling effects in the alternating-current poling of thin PIN-PMN-PT single crystals. Appl Phys Lett. 2022;120:142901.
- Luo C, Wan H, Chang WY, et al. Effect of low-frequency alternating current poling on 5-mm-thick 0.7Pb(Mg1/3Nb2/3)O3-0.3PbTiO3 single crystals. Appl Phys Lett. 2019;115:192904.
- Guo L, Su B, Wang C, et al. Orientation dependence of dielectric and piezoelectric properties of tetragonal relaxor ferroelectric single crystals by alternate current poling. J Appl Phys. 2020;127:184104.
- Qiu C, Xu Z, An Z, et al. In-situ domain structure characterization of Pb(Mg1/3Nb2/3)O3-PbTiO3 crystals under alternating current electric field poling. Acta Mater. 2021;210:116853.
- Chang W-Y, Chung C-C, Luo C, et al. Dielectric and piezoelectric properties of 0.7 Pb(Mg1/3Nb2/3)O3-0.3 PbTiO3 single crystal poled using alternating current. Mater Res Lett. 2018;6:537–544.
- Liu J, Qiu C, Qiao L, et al. Impact of alternating current electric field poling on piezoelectric and dielectric properties of Pb(In1/2Nb1/2)O3–Pb(Mg1/3Nb2/3)O3–PbTiO3 ferroelectric crystals. J Appl Phys. 2020;128:094104.
- Wan H, Luo C, Chung C-C, et al. Enhanced dielectric and piezoelectric properties of manganese-doped Pb(In1/2Nb1/2)O3-Pb(Mg1/3Nb2/3)O3-PbTiO3 singlecrystals by alternating current poling. Appl Phys Lett. 2021;118:102904.
- Wan H, Luo C, Liu C, et al. Alternating current poling on sliver-mode rhombohedral Pb(Mg1/3Nb2/3)O3-PbTiO3 single crystals. Acta Mater. 2021;208:116759.
- Ma M, Xia S, Gao X, et al. Enhanced energy harvesting performance of PIN-PMN-PT single crystal unimorph using alternating current poling. Appl Phys Lett. 2022;120:042902.
- Xu J, Zhang Z, Liu S, et al. Optimizing the piezoelectric vibration of Pb(Mg1/3Nb2/3)O3-0.25PbTiO3 single crystal by alternating current polarization for ultrasonic transducer. Appl Phys Lett. 2020;116:202903.
- Kim H-P, Wan H, Luo C, et al. A review on alternating current poling for perovskite relaxor-PbTiO3 single crystals. IEEE Trans Ultrason Ferroelectr Freq Control. 2022;69:3037–3047.
- Wang B, Li F, Chen L-Q. Inverse domain-size dependence of piezoelectricity in ferroelectric crystals. Adv Mater. 2021;33:2105071.
- Qiu C, Wang B, Zhang N, et al. Transparent ferroelectric crystals with ultrahigh piezoelectricity. Nature. 2020;577:350–354.
- Lee G-J, Kim H-P, Lee S-G, et al. Depolarization mechanism of alternating-current-poled Pb(Mg1/3Nb2/3)O3-PbTiO3 single crystals measured using in-situ thermally stimulated depolarization current. J Sens Sci Technol. 2020;29:59–62.
- Zhao X, Fang B, Cao H, et al. Dielectric and piezoelectric performance of PMN–PT single crystals with compositions around the MPB: influence of composition, poling field and crystal orientation. Mater Sci Eng B. 2002;96:254–262.
- Chang W-Y, Huang W, Bagal A, et al. Study on dielectric and piezoelectric properties of 0.7Pb(Mg1/3Nb2/3)O3-0.3PbTiO3 single crystal with nano-patterned composite electrode. J Appl Phys. 2013;114:114103.
- Sluka T, Tagantsev AK, Damjanovic D, et al. Enhanced electromechanical response of ferroelectrics due to charged domain walls. Nat Commun. 2012;3:748.
- Kim H-P, Lee G-J, Cho J-H, et al. In situ total strain measurements revealing the strain mechanism of Pb(Mg1/3Nb2/3)O3PbTiO3 single crystals. J Materiomics. 2021;7:693–698.
- Noheda B, Cox DE, Shirane G, et al. Phase diagram of the ferroelectric relaxor (1− x) PbMg1/3Nb2/3O3−x PbTiO3. Phys Rev B. 2002;66:054104.
- Sun Y, Karaki T, Fujii T, et al. Alternate current poling and direct current poling for Pb(Mg1/3Nb2/3)O3–PbTiO3 single crystals. Jpn J Appl Phys. 2019;58:SLLC06.
- Colla EV, Weissman MB. Two-step phase changes in cubic relaxor ferroelectrics. Phys Rev B. 2005;72:104106.
- Gehring PM, Ohwada K, Shirane G. Electric-field effects on the diffuse scattering in Pb Zn1/3Nb2/3O3 doped with 8% PbTiO3. Phys Rev B. 2004;70:014110.
- Echizenya K, Nakamura K, Mizuno K. PMN-PT and PIN-PMN-PT single crystals grown by continuous-feeding Bridgman method. J Cryst Growth. 2020;531:125364.