Abstract
Extracellular vesicles (ECV), like exosomes, gained recently a lot of attention as potentially playing a significant role in neurodegenerative diseases, particularly in Aβ pathology. While there are a lot of reports on ECV/exosomes derived from a variety of cell types, there is limited information on ECV/exosomes originated from brain microvascular endothelial cells forming the blood-brain barrier (BBB). In this review, we summarize the literature data on brain endothelial ECV/exosomes and present our own data on BBB-derived ECV and their possible involvement in the brain's Aβ pathology. We propose that ECV/exosome release from brain endothelial cells associated with Aβ affects different cells of the neurovascular unit and may be an important contributor to the Aβ deposition in the central nervous system.
Introduction
Brain microvascular endothelial cells joined by tight junctions constitute the anatomical basis of the blood-brain barrier (BBB). These cells line the brain microvessels and represent the major interface between the blood and the central nervous system. They maintain the brain homeostasis by regulating BBB permeability as well as molecular and cellular traffic across the barrier.Citation1
The BBB, together with the neighboring cells, including pericytes, perivascular astrocytes, microglia, and neurons, forms a functional unit called the neurovascular unit. In this structural and functional module, a capillary fragment together with the associated other cell types, support several neurons. Within the neurovascular unit, there is a complex physiological and pathological cross-talk between the different cell types.Citation2 Disturbances of the BBB and neurovascular unit dysfunction are important players in the pathogenesis of neurodegenerative diseases, including Alzheimer's disease (AD).Citation3 It is believed that the BBB is critical for amyloid β (Aβ) homeostasis and may have a role in Aβ accumulation in the brain.Citation4 According to the amyloid cascade hypothesis, Aβ deposition in the brain may contribute to neurodegeneration.Citation3
Extracellular Vesicles Represent A Complex Carrier System
Extracellular vesicles (ECV) have drawn attention as being important players in a variety of neurodegenerative diseases. ECV are heterogeneous and include exosomes Citation5 and other larger vesicles derived from plasma membrane shedding, e.g., microvesicles ranging in size from 100 to 1000 nm.Citation6,7,8
Exosomes are small ECV secreted by cells of approximately 30-100 nm in size. Exosomes carry diverse cellular cargo originating from the parent cell including mRNAs, miRNAs, lipids and proteins. After release, exosomes can enter the circulatory system, being carried to distant sites in the periphery, or they can act on cells in the immediate microenvironment. Exosomes reaching their target are internalized by recipient cells through endocytosis.Citation9 The exosome membrane contains various proteins; some of them may be involved in exosome targeting, like tetraspanins.Citation10 Even if they originate from the same cell, secreted extracellular vesicles may be of different sizes and may enclose various cargo-molecules,Citation11 which are considered important players in a special way of cell-cell communication. Because exosomes bud from endosomes, they contain a variety of proteins including membrane transport and fusion proteins (GTPases, annexins, flotillin) as well as tetraspanins (CD9, CD63, CD81, and CD82).Citation12 Marker proteins widely used to characterize exosomes include the tetraspanins CD63, CD9, or Alix.
Exosomes were shown to be released from brain cells, including neurons, glial cells, astrocytes and oligodendrocytes, and it is believed that they create a highly unique cell-cell communication system, which may play an important role in the physiology and pathology of the central nervous system. Exosomes may also have diagnostic and therapeutic potential in the brain.Citation13
Exosomes and the Aβ Pathology in the Central Nervous System
The contribution of exosomes to neurodegenerative disorders, like Alzheimer's disease (AD) involving Aβ pathology has been summarized in several reviews.Citation10,14,15 Aβ enclosed in exosomes was hypothesized to take part in a prion-like propagation of Aβ/Aβ deposits.Citation16 They were proposed to serve as an early diagnostic tool, as it was reported that Aβ 1-42 levels, together with phospho-tau, in blood exosomes of neural origin predicted AD up to 10 years before onset.Citation17
There are conflicting opinions on the role of exosomes in the Aβ pathology in AD. On one hand, serum exosome levels were correlated with increased amyloid plaque burden in an AD 5XFAD mouse model,Citation18 and inhibition of exosome secretion by the nSMase2 inhibitor GW4869 reduced amyloid plaque formation in vivo in the same mouse model.Citation19 Exosomes were nicknamed the ‘Trojan horse’ of neurodegenerative diseases carrying Aβ and transferring Aβ easily to other cells.Citation20 On the other hand neuroblastoma-derived exosomes injected into another AD-model mouse brain took up Aβ, leading to reduced Aβ levels, lower amyloid depositions, and decreased Aβ-mediated synaptotoxicity in the hippocampus. This suggested a role for neuroblastoma cell exosomes in Aβ clearance.Citation21 Similar findings were also described for neuron-derived exosomes.Citation22
ECV from Peripheral Endothelial Cells
ECV originating from peripheral endothelial cells were assigned diverse functions and were shown to have different cargo molecules. For instance, endothelial ECV secreted from activated or apoptotic endothelial cells may participate both in harmful or beneficial events of the vascular endothelial response. These responses may include coagulation/anticoagulation, inflammation/anti-inflammatory effects, angiogenesis, endothelial survival, and regeneration. Therefore, these microparticles may have a dual role in vascular homeostasis.Citation23 It was also demonstrated that human cytomegalovirus infected human umbilical vein endothelial cells (HUVEC) secreted exosomes that were immunogenic, possibly playing a role in immune surveillance.Citation24
Peripheral endothelial ECV cargo content can be very diverse. Among these cargo molecules, secretion of HSP70 via exosomes from rat aortic endothelial cells was demonstrated.Citation25 In another report, ECV released by HUVEC undergoing apoptosis and autophagy were enriched with autophagosomes and mitochondria, containing HSP60, HSP90, S100A8, and ATP. It was suggested that these ECV could be involved in the modulation of innate immunity.Citation26 ECV originating from HUVEC may contain angiopoietin-2, which is involved in the regulation of vascular integrity and inflammation.Citation27 When HUVEC were treated with chemotherapeutic agents, more exosomes were released containing miR-503, which exert antitumor activity by diminishing breast cancer cell proliferation and invasion.Citation28 This pointed to the therapeutic potential of endothelial exosomes. Neprilysin was also postulated to be released from endothelial cells via exosomes.Citation29 In addition, human dermal microvascular endothelial cells selectively sequestered cytoplasmic RNA-degrading machineries in exosomes, which may be involved in gene regulation.Citation30
It is interesting that the cargo type may change upon stimuli. For instance, when human dermal microvascular endothelial cells were exposed to different types of cellular stress, the secreted exosome protein and RNA content changed depending on the stress factor as evidenced by microarray analysis and a quantitative proteomics approach. This suggested that endothelial exosomes might transfer stress factors to the target cells by this unique communication system.Citation31
Although these studies do not specifically report on BBB relevance, we may assume that ECV originating from peripheral endothelial cells can cross the BBB and deliver their cargo into the central nervous system (CNS).
ECV as Drug Carriers into the CNS
The idea of using ECV from different cell types as carriers for various cargo molecules including drugs in the treatment of CNS diseases has been explored in the field. It was demonstrated that autologous exosomes (derived from dendritic cells) can be targeted to deliver siRNA to neurons, microglia, and oligodendrocytes of the mouse brain. The intravenously injected exosomes contained glyceraldehyde-3-phosphate dehydrogenase (GAPDH) siRNA and a specific knockdown of the GAPDH gene in the brain was achieved. Moreover, the therapeutic potential of the exosome-based delivery system was demonstrated in delivering the APP cleaving enzyme (BACE1) siRNA, resulting in downregulation of the BACE1 protein. In these experiments, exosomes represented a safe and efficient delivery tool by crossing the BBB noninvasively and without being immunogenic.Citation32 Exosomes were also used for treatment of brain inflammatory diseases. Specifically, intranasally injected curcumin-loaded exosomes from different cell types were capable of inhibiting brain inflammation and autoimmune responses in a model of experimental autoimmune encephalomyelitis. Treatment with exosomes loaded with the Stat3 inhibitor JSI-124 resulted in delayed tumor growth in a model of glioblastoma. In both cases, the drugs enclosed in exosomes and administered via the intranasal route reached the brain rapidly and showed effectiveness.Citation33 Recently, exosomes derived from endothelial cells were also demonstrated to be suitable for various cargo delivery in vitro. For example, exosomes from primary aortic endothelial cells were reported to successfully deliver siRNA against luciferase into endothelial cells in a gene-silencing study.Citation34 However, additional studies are needed to explore if endothelial ECV can successfully deliver different cargo molecules into the brain as well.
Brain Endothelial Cell-Derived ECV
While a lot of information is available on ECV from different cell types, including peripheral endothelial cells, only few reports are found in the literature regarding ECV derived from brain microvascular endothelial cells forming the BBB.
The first study mentioning brain endothelial ECV was published by Virgintino et al.Citation35 The authors observed a fascinating phenomenon in the developing human brain's vascular system. Specifically, the tip endothelial cells of the sprouting microvessels emitted several long and spider-web like filopodia as revealed by immunofluorescence and confocal microscopy. These filopodia were “decorated” with endothelial microvesicles, which were tightly associated with these elongations and appeared to follow them during the vessel sprouting. The authors speculated that these vesicles may contain signaling molecules and may be involved in cell-cell communication, and tip cell guidance to find the target cells during angiogenesis in the developing brain. They concluded that further studies would shed light on the content and role of these endothelial microvesicles. To the best of our knowledge, this paper was the first to describe microvesicles derived from human brain microvascular endothelial cells.
Soon after that, in 2013, a method for isolation and characterization of brain endothelial ECV gave more insight into their features.Citation36 The in vitro BBB model used in this case was represented by the immortalized human brain microvascular endothelial cells.Citation37 The authors proposed that BBB-derived ECV can potentially have different functions: they may contain BBB-specific biomarkers, may be communication tools among the different cell types of the neurovascular unit, and may cross the cells through receptor mediated transcytosis mechanism. In this work, two ultracentrifugation steps at 100,000 x g for 60 min at 4°C were used to isolate ECV and ECV signature profiles were characterized using mass spectrometry-based proteomics. A total of 1179 proteins were identified. More than 60 known markers of ECV have been described.Citation38 The majority of these markers (58 out of 65) including Alix, TSG101 and the tetraspanin proteins CD81 and CD9 and many other ExoCarta proteins (a large proteomics database of ECV from different human cells) were detected. 524 proteins were not found in ExoCarta suggesting that they may be specific for brain endothelial cells. 35% of these were cell-surface and 65% were intracellular proteins. The 1179 identified proteins were involved in biological processes like intracellular trafficking, signal transduction, cell adhesion, and cell motility. They included RNA/DNA-binding proteins, receptors, structural proteins and enzymes. The authors concluded that these ECVs can take part in cell-cell communication within or outside of the neurovascular unit.
Later, another paper described research results from mouse brain endothelial exosomes which when preincubated with curcumin, demonstrated beneficial effects on endothelial dysfunction, like oxidative stress and increased permeability.Citation39 In these experiments, mouse brain endothelial cells were primed with curcumin for 72 h and exosomes were isolated from the cell culture media. Isolation ultracentrifugation was used at 100,000 x g for 1 h, followed by immunoprecipitation with exo-specific beads. The curcumin-primed exosomes alleviated hyperhomocysteinemia-induced brain endothelial dysfunction by diminishing oxidative stress, tight junction protein alterations, and endothelial permeability increase. These results suggested that BBB-derived exosomes may be used as therapeutic tools in BBB disturbances. Mouse brain endothelial cell line (bEND3)-derived exosomes were also shown to effectively deliver the anticancer drugs doxorubicin and paclitaxel across the BBB for the treatment of brain cancer in a zebrafish model, demonstrating the therapeutic potential in the treatment of CNS diseases.Citation40
Because ECV are heterogeneous, several methods were proposed for their isolation. The methods have not been standardized and controversy exists about which isolation approach is most suitable.Citation41 Therefore, it is important to take into account the isolation method when interpreting the results of individual research reports. In our laboratory, we use ExoQuickTC to isolate ECV/exosomes from the culture media of human brain microvascular endothelial cells (HBMEC).Citation37 Exosomes are traced with CD63-GFP and/or CD9-RFP fusion Cyto-Tracers from SBI Biosciences. The tetraspanin CD63 and CD9 proteins are common biomarkers for exosomes. Using the CD63 and CD9 Cyto-Tracer constructs, the transiently transfected HBMEC secrete green or red fluorescent vesicles. For better visualization of individual cells secreting glowing exosomes, a double transfection is used. By cotransfecting HBMEC with pT-CYTO RFP (which gives the cytoplasm red fluorescence) and pT CD63 GFP (exosome tracer resulting in CD63 specific green fluorescence) we can follow green fluorescent ECV/exosome secretion by live cell imaging. Using this procedure, green fluorescent CD63 positive vesicles with different sizes budding from the cells can be observed (). Secreted CD63-positive (green) or CD9-positive (red) ECV/exosomes can also be observed in the cell culture media following isolation using ExoQuickTC. Isolated vesicles are fixed, mounted and imaged by fluorescence microscopy (). Visualization of ECV by differential interference contrast (DIC) imaging is presented in .
Figure 1. Extracellular vesicles from human brain microvascular endothelial cells (HBMEC). (A) Live fluorescence imaging of HBMEC transiently transfected with pT-CYTO-RFP and pT-CD63-GFP to visualize the brain endothelial cell (red) secreting green fluorescent CD63 positive extracellular vesicles (ECV). Scale bar: 2 μm. (B) HBMEC were transiently transfected with pT-CD63 GFP or pT-CD9 RFP. ECV were isolated from the cell culture media. Fluorescence microscopy images of isolated fluorescent ECV (CD63 GFP-green, upper panel; CD9 RFP-red, lower panel). Scale bar: 20 μm. (C) Differential interference contrast (DIC) image of isolated ECV. Scale bar: 2 μm.
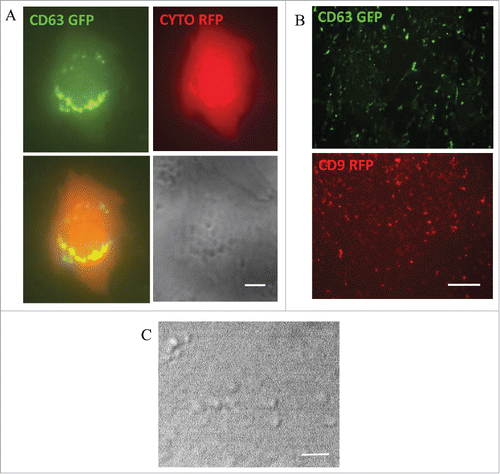
The size and volume distribution of ECV/exosome isolates is determined by dynamic light scattering (DLS) technique. Using the described isolation techniques, the DLS measurements indicate that ECV/exosome pool is highly polydisperse and prone to aggregation. Here we present one example of such measurements. The first order result from a DLS experiment is an intensity distribution of particle sizes. Intensity distribution in this sample indicates several peaks with the sizes of 68.41 nm, 609.1 nm and 5213 nm (). The intensity distribution can be converted by the software, using Mie theory, to a volume distribution or a distribution describing the relative proportion of multiple components in the sample based on their mass or volume. The conversion assumes that all particles are spherical, homogenous, with known optical properties and that there is no error in the intensity distribution. According to the relative volume percentages, the volume distribution in sample from indicates 3 peaks: 47.4% of the volume for particles with ~600 nm, 2.4% of the volume for particles with ~5000 nm. Interestingly, 50.1% of the total volume is made up by particles with an average diameter of ~60 nm, which is consistent with the size range of exosomes ().
Figure 2. Dynamic light scattering analysis of isolated ECV from HBMEC. HBMEC-derived ECV were isolated from the cell culture media. (A) Size distribution indicates several peaks with the sizes of 68.41 nm, 609.1 nm and 5213 nm. (B) Volume distribution: 50.1% of the volume is for particles of ~60 nm, 2.4% for particles of ~5000 nm, and 47.4% for particles with an average diameter of ~600 nm.
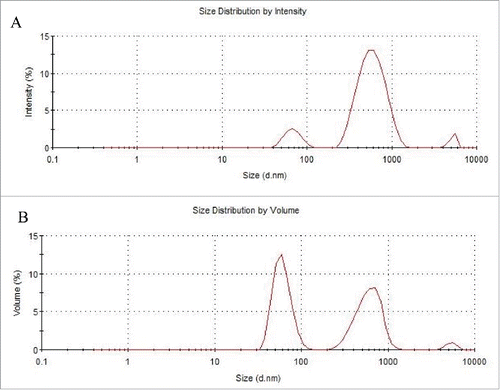
Because ECV/exosomes from other cell types were reported to be important players in the brain's Aβ pathology,Citation18,19,21,22 we hypothesized that BBB-derived ECV could also contribute to this pathology. HBMEC were exposed to fluorescently labeled Aβ, specifically to 100 nM Aβ (1-40) HiLyte, for 48 h. ECV from the cell culture media were isolated using ExoQuickTC. An interesting phenomenon was observed as Aβ (1-40) HiLyte was associated with ECV of very different sizes. Surprisingly, not only smaller but also larger vesicles contained Aβ (1-40) HiLyte cargo as indicated by green fluorescence under fluorescence microscopy (). This suggests that BBB-derived different ECV, and not only exosomes, may have a role in distributing Aβ within the central nervous system.
Figure 3. Exogenous Aβ (1-40) HiLyte in HBMEC-derived ECV. HBMEC were exposed to 100 nM Aβ (1-40) HiLyte for 48 h, followed by ECV isolation from cell culture media. Aβ (1-40) HiLyte (green fluorescence) in the isolated ECV was visualized by fluorescence microscopy. The images show the green fluorescent Aβ (1-40) HiLyte associated with ECV of different sizes. Scale bar: 20 μm.
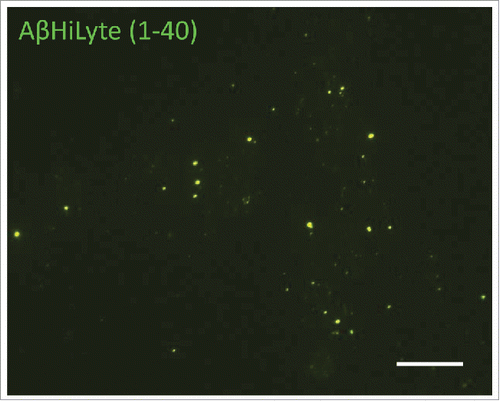
Concluding Remarks
While limited literature data exist regarding ECV from the BBB, there is a critical need to understand this area better. BBB-derived ECV may have a crucial role in the brain's physiological and pathological processes. For example, BBB-derived ECV of different sizes may carry and transfer Aβ among the neighboring cells of the neurovascular units, possibly leading to neurovascular dysfunction in the course of AD.
Disclosure of Potential Conflicts of Interest
No potential conflicts of interest were disclosed.
Acknowledgments
We would like to thank Dr. Emre Dikici from Dr. Sylvia Daunert's laboratory for his help with the DLS measurements.
Funding
Supported by NIH/NHLBI (HL126559), NIH/NIDA (DA039576 and DA027569), and NIH/NIMH (MH063022, MH098891). We acknowledge support from the Miami Center for AIDS Research (CFAR) at the University of Miami Miller School of Medicine funded by a grant (P30AI073961) from the National Institutes of Health (NIH).
References
- Abbott NJ. Blood-brain barrier structure and function and the challenges for CNS drug delivery. J Inherit Metab Dis 2013; 36:437-49; PMID:23609350; http://dx.doi.org/10.1007/s10545-013-9608-0
- Abbott NJ, Friedman A. Overview and introduction: the blood-brain barrier in health and disease. Epilepsia 2012; 53 Suppl 6:1-6; PMID:23134489; http://dx.doi.org/10.1111/j.1528-1167.2012.03696.x
- Erickson MA, Banks WA. Blood-brain barrier dysfunction as a cause and consequence of Alzheimer's disease. J Cereb Blood Flow Metab 2013; 33:1500-13; PMID:23921899; http://dx.doi.org/10.1038/jcbfm.2013.135
- Deane R, Zlokovic BV. Role of the blood-brain barrier in the pathogenesis of Alzheimer's disease. Curr Alzheimer Res 2007; 4:191-7; PMID:17430246; http://dx.doi.org/10.2174/156720507780362245
- Mathivanan S, Ji H, Simpson RJ. Exosomes: extracellular organelles important in intercellular communication. J Proteomics 2010; 73:1907-20; PMID:20601276; http://dx.doi.org/10.1016/j.jprot.2010.06.006
- Thery C, Ostrowski M, Segura E. Membrane vesicles as conveyors of immune responses. Nat Rev Immunol 2009; 9:581-93; PMID:19498381; http://dx.doi.org/10.1038/nri2567
- Meckes DG, Jr, Raab-Traub N. Microvesicles and viral infection. J Virol 2011; 85:12844-54; PMID:21976651; http://dx.doi.org/10.1128/JVI.05853-11
- Cocucci E, Racchetti G, Meldolesi J. Shedding microvesicles: artefacts no more. Trends Cell Biol 2009; 19:43-51; PMID:19144520; http://dx.doi.org/10.1016/j.tcb.2008.11.003
- Tian T, Wang Y, Wang H, Zhu Z, Xiao Z. Visualizing of the cellular uptake and intracellular trafficking of exosomes by live-cell microscopy. J Cell Biochem 2010; 111:488-96; PMID:20533300; http://dx.doi.org/10.1002/jcb.22733
- Gupta A, Pulliam L. Exosomes as mediators of neuroinflammation. J Neuroinflammation 2014; 11:68; PMID:24694258; http://dx.doi.org/10.1186/1742-2094-11-68
- Colombo M, Moita C, van Niel G, Kowal J, Vigneron J, Benaroch P, Manel N, Moita LF, Thery C, Raposo G. Analysis of ESCRT functions in exosome biogenesis, composition and secretion highlights the heterogeneity of extracellular vesicles. J Cell Sci 2013; 126:5553-65; PMID:24105262; http://dx.doi.org/10.1242/jcs.128868
- Vlassov AV, Magdaleno S, Setterquist R, Conrad R. Exosomes: current knowledge of their composition, biological functions, and diagnostic and therapeutic potentials. Biochim Biophys Acta 2012; 1820:940-8; PMID:22503788; http://dx.doi.org/10.1016/j.bbagen.2012.03.017
- Kawikova I, Askenase PW. Diagnostic and therapeutic potentials of exosomes in CNS diseases. Brain Res 2015; 1617:63-71; PMID:25304360; http://dx.doi.org/10.1016/j.brainres.2014.09.070
- Vella LJ, Sharples RA, Nisbet RM, Cappai R, Hill AF. The role of exosomes in the processing of proteins associated with neurodegenerative diseases. Eur Biophys J 2008; 37:323-32; PMID:18064447; http://dx.doi.org/10.1007/s00249-007-0246-z
- Kalani A, Tyagi A, Tyagi N. Exosomes: mediators of neurodegeneration, neuroprotection and therapeutics. Mol Neurobiol 2014; 49:590-600; PMID:23999871; http://dx.doi.org/10.1007/s12035-013-8544-1
- Vingtdeux V, Sergeant N, Buee L. Potential contribution of exosomes to the prion-like propagation of lesions in Alzheimer's disease. Front Physiol 2012; 3:229; PMID:22783199; http://dx.doi.org/10.3389/fphys.2012.00229
- Fiandaca MS, Kapogiannis D, Mapstone M, Boxer A, Eitan E, Schwartz JB, Abner EL, Petersen RC, Federoff HJ, Miller BL, et al. Identification of preclinical Alzheimer's disease by a profile of pathogenic proteins in neurally derived blood exosomes: A case-control study. Alzheimers Dement 2015; 11:600-7 e1; PMID:25130657; http://dx.doi.org/10.1016/j.jalz.2014.06.008
- Dinkins MB, Dasgupta S, Wang G, Zhu G, He Q, Kong JN, Bieberich E. The 5XFAD Mouse Model of Alzheimer's Disease Exhibits an Age-Dependent Increase in Anti-Ceramide IgG and Exogenous Administration of Ceramide Further Increases Anti-Ceramide Titers and Amyloid Plaque Burden. J Alzheimers Dis 2015; 46:55-61; PMID:25720409; http://dx.doi.org/10.3233/JAD-150088
- Dinkins MB, Dasgupta S, Wang G, Zhu G, Bieberich E. Exosome reduction in vivo is associated with lower amyloid plaque load in the 5XFAD mouse model of Alzheimer's disease. Neurobiol Aging 2014; 35:1792-800; PMID:24650793; http://dx.doi.org/10.1016/j.neurobiolaging.2014.02.012
- Bellingham SA, Guo BB, Coleman BM, Hill AF. Exosomes: vehicles for the transfer of toxic proteins associated with neurodegenerative diseases? Front Physiol 2012; 3:124; PMID:22563321; http://dx.doi.org/10.3389/fphys.2012.00124
- Yuyama K, Sun H, Sakai S, Mitsutake S, Okada M, Tahara H, Furukawa J, Fujitani N, Shinohara Y, Igarashi Y. Decreased amyloid-β pathologies by intracerebral loading of glycosphingolipid-enriched exosomes in Alzheimer model mice. J Biol Chem 2014; 289:24488-98; PMID:25037226; http://dx.doi.org/10.1074/jbc.M114.577213
- Yuyama K, Sun H, Usuki S, Sakai S, Hanamatsu H, Mioka T, Kimura N, Okada M, Tahara H, Furukawa J, et al. A potential function for neuronal exosomes: sequestering intracerebral amyloid-β peptide. FEBS Lett 2015; 589:84-8; PMID:25436414; http://dx.doi.org/10.1016/j.febslet.2014.11.027
- Dignat-George F, Boulanger CM. The many faces of endothelial microparticles. HUVEC ECV may also participate in immune surveillance. Arterioscler Thromb Vasc Biol 2011; 31(1):27-33; PMID:21160065; http://dx.doi.org/10.1161/ATVBAHA.110.218123
- Walker JD, Maier CL, Pober JS. Cytomegalovirus-infected human endothelial cells can stimulate allogeneic CD4+ memory T cells by releasing antigenic exosomes. J Immunol 2009; 182(3):1548-59; PMID:19155503; http://dx.doi.org/10.4049/jimmunol.182.3.1548
- Zhan R, Leng X, Liu X, Wang X, Gong J, Yan L, Wang L, Wang Y, Wang X, Qian LJ. Heat shock protein 70 is secreted from endothelial cells by a non-classical pathway involving exosomes. Biochem Biophys Res Commun 2009; 387(2):229-33; PMID:19555663; http://dx.doi.org/10.1016/j.bbrc.2009.06.095
- Pallet N, Sirois I, Bell C, Hanafi LA, Hamelin K, Dieudé M, Rondeau C, Thibault P, Desjardins M, Hebert MJ. A comprehensive characterization of membrane vesicles released by autophagic human endothelial cells. Proteomics 2013; 13(7):1108-20; PMID:23436686; http://dx.doi.org/10.1002/pmic.201200531
- Ju R, Zhuang ZW, Zhang J, Lanahan AA, Kyriakides T, Sessa WC, Simons M. Angiopoietin-2 secretion by endothelial cell exosomes: regulation by the phosphatidylinositol 3-kinase (PI3K)/Akt/endothelial nitric oxide synthase (eNOS) and syndecan-4/syntenin pathways. J Biol Chem 2014; 289(1):510-9; http://dx.doi.org/10.1074/jbc.M113.506899
- Bovy N, Blomme B, Frères P, Dederen S, Nivelles O, Lion M, Carnet O, Martial JA, Noël A, Thiry M, et al. Endothelial exosomes contribute to the antitumor response during breast cancer neoadjuvant chemotherapy via microRNA transfer. Oncotarget. 2015; 6(12):10253-66; PMID:25860935; http://dx.doi.org/10.18632/oncotarget.3520
- Kuruppu S, Rajapakse NW, Minond D, Smith AI. Production of soluble neprilysin by endothelial cells. Biochem Biophys Res Commun 2014; 446(2):423-7; PMID:24495806; http://dx.doi.org/10.1016/j.bbrc.2014.01.158
- van Balkom BW, Eisele AS, Pegtel DM, Bervoets S, Verhaar MC. Quantitative and qualitative analysis of small RNAs in human endothelial cells and exosomes provides insights into localized RNA processing, degradation and sorting. J Extracell Vesicles 2015; 4:26760; PMID:26027894
- de Jong OG, Verhaar MC, Chen Y, Vader P, Gremmels H, Posthuma G, Schiffelers RM, Gucek M, van Balkom BW. Cellular stress conditions are reflected in the protein and RNA content of endothelial cell-derived exosomes. J Extracell Vesicles 2012; 1: 18396; PMID:24009886
- Alvarez-Erviti L, Seow Y, Yin H, Betts C, Lakhal S, Wood MJ. Delivery of siRNA to the mouse brain by systemic injection of targeted exosomes. Nat Biotechnol 2011; 29:341-5: 24858; PMID:21423189; http://dx.doi.org/10.1038/nbt.1807
- Zhuang X, Xiang X, Grizzle W, Sun D, Zhang S, Axtell RC, Ju S, Mu J, Zhang L, Steinman L, et al. Treatment of brain inflammatory diseases by delivering exosome encapsulated anti-inflammatory drugs from the nasal region to the brain. Mol Ther 2011; 19:1769-79; PMID:21915101; http://dx.doi.org/10.1038/mt.2011.164
- Banizs AB, Huang T, Dryden K, Berr SS, Stone JR, Nakamoto RK, Shi W, He J. In vitro evaluation of endothelial exosomes as carriers for small interfering ribonucleic acid delivery. Int J Nanomedicine 2014; 9:4223-30; PMID:25214786
- Virgintino D, Rizzi M, Errede M, Strippoli M, Girolamo F, Bertossi M, Roncali L. Plasma membrane-derived microvesicles released from tip endothelial cells during vascular sprouting. Angiogenesis 2012; 15:761-9; PMID:22886085; http://dx.doi.org/10.1007/s10456-012-9292-y
- Haqqani AS, Delaney CE, Tremblay TL, Sodja C, Sandhu JK, Stanimirovic DB. Method for isolation and molecular characterization of extracellular microvesicles released from brain endothelial cells. Fluids Barriers CNS 2013; 10:4; PMID:23305214; http://dx.doi.org/10.1186/2045-8118-10-4
- Weksler BB, Subileau EA, Perriere N, Charneau P, Holloway K, Leveque M, Tricoire-Leignel H, Nicotra A, Bourdoulous S, Turowski P, et al. Blood-brain barrier-specific properties of a human adult brain endothelial cell line. FASEB J 2005; 19:1872-4; PMID:16141364
- Simpson RJ, Jensen SS, Lim JW. Proteomic profiling of exosomes: current perspectives. Proteomics 2008; 8:4083-99; PMID:18780348; http://dx.doi.org/10.1002/pmic.200800109
- Kalani A, Kamat PK, Chaturvedi P, Tyagi SC, Tyagi N. Curcumin-primed exosomes mitigate endothelial cell dysfunction during hyperhomocysteinemia. Life Sci 2014; 107:1-7; PMID:24780320; http://dx.doi.org/10.1016/j.lfs.2014.04.018
- Yang T, Martin P, Fogarty B, Brown A, Schurman K, Phipps R, Yin VP, Lockman P, Bai S. Exosome delivered anticancer drugs across the blood-brain barrier for brain cancer therapy in Danio rerio. Pharm Res 2015; 32(6):2003-14; http://dx.doi.org/10.1007/s11095-014-1593-y
- Van Deun J, Mestdagh P, Sormunen R, Cocquyt V, Vermaelen K, Vandesompele J, Bracke M, De Wever O, Hendrix A. The impact of disparate isolation methods for extracellular vesicles on downstream RNA profiling. J Extracell Vesicles 2014; 3: 24858; PMID:25317274