ABSTRACT
Autophagy is a cell survival mechanism by which bulk cytoplasmic material, including soluble macromolecules and organelles, is targeted for lysosomal degradation. The role of autophagy in diverse cellular processes such as metabolic stress, neurodegeneration, cancer, aging, immunity, and inflammatory diseases is being increasingly recognized. Epithelial cell junctions play an integral role in the cell homeostasis via physical binding, regulating paracellular pathways, integrating extracellular cues into intracellular signaling, and cell-cell communication. Recent data indicates that cell junction composition is very dynamic. The junctional protein complexes are actively regulated in response to various intra- and extra-cellular clues by intracellular trafficking and degradation pathways. This review discusses the recent and emerging information on how autophagy regulates various epithelial cell junctions. The knowledge of autophagy regulation of epithelial junctions will provide further rationale for targeting autophagy in a wide variety of human disease conditions.
Epithelial junctions
Cell junctions occur at points of cell-cell and cell-matrix contact in all tissues, particularly in epithelia. In vertebrates, 3 major types of cell junctions occur in the epithelia; tight junctions (TJ), adherens junctions (AJ), and gap junctions (GJ).Citation1 The most apically located inter-cellular TJs polarize the epithelial cell membrane into apical and basolateral regions (fence function) and regulate passive diffusion of solutes and macromolecules in between adjacent cells (gate function).Citation2 TJs consist of an array of membrane-spanning proteins such as occludin and claudins, linked by cytoplasmic plaque protein zona occludin to the cytoskeleton. The TJs act as a paracellular barrier and serve as a first line of defense against paracellular permeation of noxious antigens into the epithelia and subepithelial host tissue.Citation3,4 The TJs consists of structural barrier forming proteins such as occludin, and claudin−1, −4, −8 etc. as well as pore forming claudins such as claudin−2 and −15. Disturbances in this TJ composition play an important role in variety of diseases including inflammatory bowel diseases, kidney diseases such as hypomagnesemia and hypercalciuria, autoimmune diseases, dermal or retinal disease etc. Just below the TJs, AJs encircle interacting adjoining cells. In AJs, special proteins called cadherins form homodimers in the plasma membrane of opposite cell.Citation5,6 The intracellular domain of the cadherins binds to intracellular actin filaments via catenin anchor family proteins. The cadherin–β-catenin–α-catenin complex has been shown to interact with actin filaments via several actin binding proteins such as vinculin,Citation7 formin,Citation8 and EPLIN (epithelial protein lost in neoplasm; also known as Lima-1).Citation9 Besides actin filaments, microtubulesCitation10,11 and nectins, a family of immunoglobulin-like moleculesCitation12 are known to regulate the assembly of AJs. In addition to being a mechanical linkage of neighboring cells to each other, AJ protein complexes are important regulators of morphogenesis, contact inhibition, proliferation, and are key sensors and transmitters of the extracellular cues converging into intracellular signaling.Citation13 Below the AJs, the adjoining cells communicate via GJs that are made up of intercellular channels formed by 6 subunits of connexins that are bundled together in homomeric or heteromeric fashion. GJs allow exchange of small metabolites, ions, and second messengers between adjacent cells that is essential for physiological functions such as cell synchronization, differentiation, cell growth, and metabolic coordination of avascular organs including epidermis and lens.Citation14,15
Junctional homeostasis through constant protein turnover
The dynamic nature of cell junctions necessitates continuous endocytosis and recycling of junctional proteins to-and-from the membrane. The endocytosed junctional proteins, under various physiological and pathological conditions, may be diverted to the degradation pathway. Emerging evidence shows that TJs are highly interactive and dynamic in nature and intracellular vesicular membrane transport is a key process in the formation of TJ domains.Citation16,17 The TJ complex undergoes constant remodeling and intracellular trafficking where TJ proteins are continuously inserted and internalized from the membrane.Citation18 AJs, too, are regulated by intracellular trafficking in addition to transcriptional and posttranscriptional regulation. Following disruption of AJs, such as after extra cellular calcium depletion, E-cadherins are rapidly internalized.Citation19,20 Interactions with β-catenin, p120-catenin, and nectins are important for stabilizing E-cadherins at the AJs.Citation21,22 In GJs, the connexin hemichannels called connexons are carried from ER to the cell surface via microtubule aided vesicles. The hemichannels fuse in homotypic or heterotypic fashion to form intercellular channels. From the GJ plaque of intercellular channels, old channels are removed from the center and new channels are added to the periphery in highly dynamic events. This rapid and constant synthesis and degradation of connexins at the GJs may offer quick adaptation of tissues to the changing environmental conditions.Citation23
Autophagy functions
Autophagy, an intracellular degradation pathway, refers to the engulfment and processing of cellular proteins, including damaged organelles and long-lived and misfolded proteins. Among the 3 main types of autophagy, ‘microautophagy’ involves the engulfment of cytoplasmic material that is in direct contact with the lysosomal membrane while in ‘chaperone-mediated autophagy’ cargo proteins must be recognized by the Hsc70 chaperone before being translocated into the lysosomal interior. “Macroautophagy” (commonly referred as autophagy) is characterized by sequestration of structures intended for destruction into double-membrane vesicles called autophagosomesCitation24 and is the subject of this review. The kinase mammalian target of rapamycin (mTOR) is a critical regulator of autophagy induction; activated mTOR (presence of growth factors and abundant nutrients, Akt, and MAPK signaling) suppresses autophagy while negative regulation of mTOR (nutrient starvation, stress, AMPK, and p53 signaling) induces autophagy. Downstream of mTOR, several autophagy related ATG proteins participate in ubiquitin-like conjugation reactions to control the autophagosome formation. The sequential events of vesicle nucleation (formation of the isolation membrane/phagophore), vesicle elongation and completion, and fusion of the double-membraned autophagosome with the lysosome to form an autolysosome ultimately culminate into the lysis of the autophagosomal inner membrane and breakdown of its contents inside the autolysosome.Citation25,26 An essential cell survival mechanism, autophagy plays an important role in diverse processes such as metabolic stress, neurodegeneration, cancer, aging, immunity, and inflammatory diseases.Citation25-30 The main cellular functions of autophagy includes energy and glucose metabolic adaptation to stress, elimination of excess or unneeded organelles, tumor suppressor function, and elimination of intracellular pathogens.Citation31 Besides these conventional functions, autophagy proteins have recently been shown to participate in extracellular secretion of biomolecules and cytokines,Citation32,33 osteoclastic bone resorption,Citation34 regulation of tumor microenvironment through metabolic coupling,Citation35,36 and other autophagy-independent functions such as innate viral immune response, mitochondrial homeostasis, and cell death.Citation24 In this review, we examine the potential role autophagy plays in the regulation of cell junctions.
Autophagy regulation of tight junction
In a recent study, we have shown that autophagy profoundly enhances TJ barrier function.Citation37 The TJs are known to have 2 pathways, a small-size, cation-selective, high-capacity “pore” pathway and a large-size, non-charge-selective “leak” pathway. The pore pathway is largely regulated by the TJ membrane proteins claudins, particularly claudin-2, which is a cation-selective, pore-forming protein while leak pathway is regulated by barrier forming occludin.Citation38 Induction of autophagy in human intestinal Caco-2 cells by starvation or mTOR inhibitors rapamycin and PP242 treatment caused reduction in ion permeability as reflected by increased transepithelial resistance (TER) and reduced small-size urea permeability, indicating selective autophagy targeting of the pore pathway (). Furthermore, the levels of the pore forming claudin-2 were reduced markedly after starvation or rapamycin treatment and claudin-2 was increasingly targeted for the lysosomal degradation. Pharmacologic inhibition of autophagy with bafilomycin A (V-ATPase inhibitor that impedes autolysosome formation),Citation39chloroquine (inhibitor of lysosomal acidification),Citation40 and wortmannin (inhibitor of class III PI3K required to initiate autophagy)Citation41 as well as genetic inhibition of autophagy with ATG16L1 and ATG7 siRNA prevented the starvation or rapamycin induced increase in TER, reduction in urea flux, and degradation of claudin-2.Citation37 These findings demonstrated that autophagy induction led to an enhancement of TJ barrier function via claudin-2 degradation. Similarly, in primary porcine intestinal epithelial cells also, autophagy played a critical role in TJ barrier function. Non-essential amino acid (NEAA) deprivation induced TJ barrier dysfunction via reduction in expression of TJ protein claudin-1 and ZO-1. Inhibition of autophagy enhanced Non-essential amino acid (NEAA) deprivation-induced TJ barrier dysfunction whereas activation of autophagy by rapamycin was protective against NEAA deprivation-induced TJ barrier dysfunction.Citation42 In mice stomach, the oxidative stress induced by hydrogen peroxide (H2O2) disrupted gastric TJ barrier, reduced the levels of TJ protein occludin and ZO-1, and inhibited autophagy. However, treatment with reactive oxygen species scavenger PDTC (Ammonium pyrrolidinedithiocarbamate) restored the H2O2-induced disruption of gastric TJ barrier and also increased the conversion of LC3I into LC3II, suggesting synergistic association between autophagy and TJ barrier enhancement.Citation43 Autophagy also plays a role in the TJ function in blood-brain barrier. In an ischemia-reperfusion (I/R) model, oxygen-glucose deprivation/reoxygenation (OGD/R) injury in brain microvascular endothelial cell (BMVEC) caused apoptosis, high levels of reactive oxygen species, and reduction in the level and redistribution of ZO-1. Autophagy induction by rapamycin and lithium carbonate attenuated these adverse effects induced by OGD/R. Rapamycin and lithium carbonate pretreatments also prevented evans blue extravasation and brain water content induced by OGD/R in the ischemic hemisphere of the rat.Citation44 In contrast, Macrophage migration inhibitory factor (MIF)-induced autophagy caused a degradation and disorganization of endothelial tight junctions resulting into vascular leakage in human microvascular endothelial cell line (HMEC-1).Citation45 Also, under prolonged ischemic conditions (OGD/R injury) in brain endothelial cells, endothelial barrier was disrupted in part by nitric oxide-induced caveolin-1-mediated trafficking of claudin-5 to the autophagosome for autophagy-lysosome-dependent degradation.Citation46 However, these effects of autophagy on the endothelial TJ barrier appear to be influenced by activation of different intracellular signaling pathwaysCitation45 and dependent on the cell type and the extent of injury. Synergistic association between autophagy and improvement of epithelial TJ barrier has been demonstrated in a diabetic retinopathy model where hyperglycemia and hypoxia caused disruption of claudin-1 and ZO-1 localization in retinal pigment epithelial cells. Fenofibric acid treatment which is known to reduce the progression of diabetic retinopathy in clinical trials, downregulated stress-mediated signaling, induced autophagy, and prevented TJ barrier disruption induced by hyperglycemia and hypoxia.Citation47 Overall, autophagy appears to be critical for homeostasis of the epithelial TJ barrier.
Figure 1. Autophagy enhances the epithelial barrier function. Filter-grown intestinal Caco-2 monolayers were incubated in starvation medium (Earle's balanced salt solution) to induce autophagy. Starvation significantly increased transepithelial resistance (TER) and progressively reduced urea flux (#, *, p < 0.01 vs. time 0). Adapted from Nighot et. al., 2015.
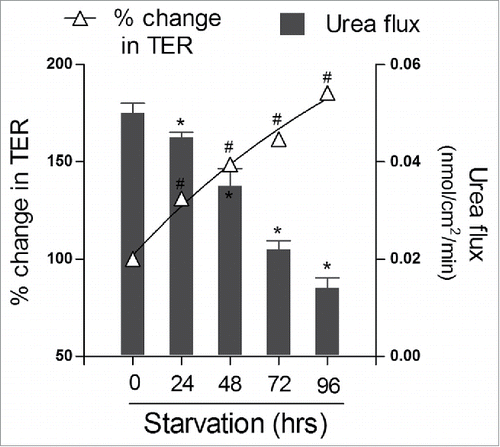
Autophagy regulation of adherens junction
The interplay between autophagy and AJs is exemplified by the classical Wnt-induced β-catenin signaling. The complex of β-catenin-E-cadherin present normally at the plasma membrane is destabilized during aberrant proliferative signaling events such as Wnt-induced β-catenin signaling in undifferentiated acute myeloid leukemia (AML) cells. The independent and constitutively active β-catenin blocked autophagy and promoted proliferation.Citation48 However, treatment with lectin LecB was found to degrade β-catenin, allowing induction of autophagy and suppress deregulated proliferation of leukemia THP-1 cells.Citation48 Autophagy is also known to contribute to therapy-induced degradation of the PML/RARA oncoprotein, allowing terminal differentiation of immature precursor cells and suppress deregulated proliferation of myeloid cells.Citation49 Moreover, the reduced levels of β-catenin also sensitized leukemia THP-1 cells to mTOR inhibitors, suggesting a potential solution for the low clinical benefit of mTOR inhibitors in AML patients.Citation50 The autophagy protein LC3 also directly interacts with β‐catenin via W/YXXI/L motif and LC3‐interacting region (LIR) in β‐catenin, and targets β‐catenin for autophagic degradation.Citation51 Autophagy is furthermore known to down-regulate SNAIL and SLUG, 2 key transcription repressor factors that regulate cadherin expression during epithelial-mesenchymal transition (EMT) process. This autophagy-mediated upregulation of N- and R-cadherins transcription and translation reduces migration and invasion abilities of glioblastoma cells by reversing EMT process.Citation52 Conversely, autophagy deficiency promotes cell migration, invasion, and proliferation via reduction in E-cadherin expression. Under autophagic deficiency, autophagosomal degradation of E-cadherin transcriptional repressor Twist1 was inhibited; further the accumulated autophagy substrate p62 (SQSTM1) stabilizes Twist1 via binding to its ubiquitin-associated domain and causes reduction in E-cadherin expression.Citation53 Thus while autophagy helps maintain E-cadherin expression and thus optimum cellular growth, β-catenin acts as a converging point between proliferative β-catenin/Wnt signaling and autophagy.Citation51
Autophagy regulation of gap junction
Autophagy plays an important role in the degradation of gap junctions during their rapid turnover. In a complex process, internalization of GJ occurs in form of a peculiar double membrane structure called annular gap junction (AGJ) which are constitutively degraded via autophagy.Citation54,55 Autophagy proteins associated with autophagosome formation and maturation, ATG5 and LC3, has been shown to be localized to AGJCitation56 and the autophagy receptor p62 has been found to be co-localized with connexin isotypes, Cx50 and Cx43.Citation57 Following traumatic brain injury (TBI), autophagy inhibition caused increased levels of phosphorylated connexin 43 (pCx43) in hippocampal astrocytes, indicating autophagy based degradation of GJs.Citation58 A single or several AGJs can be engulfed simultaneously within an individual autophagosome.Citation54 Furthermore, plasma-membrane bound connexin proteins have been shown to be involved in early biogenesis of autophagosome. Connexins form a constitutive complex with autophagy-related proteins involved in the initial steps of autophagosome formation, such as ATG16 and components of the PI(3)K autophagy initiation complex (Vps34, Beclin-1 and Vps15). Following autophagy stimulus, ATG14 joins the connexin–ATG complex leading to its internalization. The connexin containing pre-autophagosomal complex further matures into autophagosomes permitting sustained activation of autophagy.Citation59 Over all, this involvement of connexins in the early biogenesis of autophagosomes complements cell's efficiency to counteract the stressful conditions by modulation of cell communication via autophagy-mediated degradation of GJ and possibly the larger hazardous molecules entrapped in the internalized AGJ vesicle lumen.Citation60
Importance of the context for autophagy regulation of cell junctions
Whether autophagy is beneficial or harmful for the host is a highly debated question. Critical evaluation of the role of autophagy in the organization and function of cell junctions is needed. This is warranted due to the fact that autophagy is very dynamic, multistep, cell specific process, the outcome of which is dependent on the substrate that is being degraded and the context of autophagic flux.Citation61 The effect of autophagy on cell junctions may have untoward outcomes. For instance, in hypoxic melanoma cells, autophagy induction destabilizes immune synapses by increasing degradation of gap-junctional connexin Cx43, thus impairing NK cell-mediated killing. Conversely, genetic or pharmacological autophagy inhibition stabilizes immune synapses by increasing the presence of Cx43 within the immune synapses and improves NK cell-mediated lysis of hypoxic melanoma cells.Citation62 In another instance, autophagy is rapidly up-regulated in necrotizing enterocolitis (NEC) mice model. Under this situation, erythropoietin, a breast milk component that downregulates autophagy via the Akt/mTOR signaling pathway has been shown to preserve the intestinal barrier function and decrease the incidence of NEC.Citation63 The extent of autophagy induction is also a deciding factor for the outcome of autophagy induction. Among the 2 mTOR complexes that regulate autophagy, mTORC1 and mTORC2, sustained AMP-activated protein kinase (AMPK) activation of ERK MAPK has been shown to cause disassembly of mTORC2 via marked increase in beclin 1 expression, leading to cytodestructive autophagy.Citation64 In this context, mTORC2 assembly is known to regulate actin cytoskeleton and localization of TJ proteins occludin and ZO-1 via PKC-α and small GTPase Rac1.Citation65,66 Further, the substrate specific outcome of autophagy induction is illustrated by our recent study in Madin-Darby Canine Kidney MDCK cells. MDCK cells have 2 distinct strains based in part on the claudin-2 expression. MDCK I cells are claudin-2 deficient and therefore have very high TER whereas MDCK II cells have high claudin-2 expression and have low TER.Citation67,68 Autophagy induction by starvation or mTOR inhibitors rapamycin and PP242 treatment enhanced TJ barrier in MDCK II cells by markedly increasing TER, decreasing urea flux, and reducing claudin-2 protein levels. This autophagy-induced enhancement of TJ barrier was however not evident in claudin-2 deficient MDCK I cells.Citation37 Autophagy regulators may also have autophagy-independent role in TJ barrier function. For instance, AMPK is involved in cytokine-induced TJ barrier modulation, independent of intracellular energy levels.Citation69,70
Mechanisms intersecting autophagy and junction protein trafficking
In the autophagy regulation of cell junctions, which are located at the plasma membrane, the crosstalk between autophagy machinery and the endocytic machinery is a crucial step. Autophagy is known to regulate the key modes of endocytosis of junctional proteins. In case of clathrin-mediated endocytosis, various clathrin adaptor protein (AP) complexes (AP-1, −2, and −3) connect cargo proteins to clathrin coat and accessory proteins that regulate coat assembly and disassembly. AP2 complex is involved in membrane protein endocytosis and has been recently shown to function as an LC3 receptor, which shuttles endocytic cargo to autophagosomes.Citation71 TJ protein claudin-2 has been shown to be endocytosed via clathrin pits that are positive for adaptin α, a key constituent of AP-2 complexCitation72 while AJ protein E-cadherin is endocytosed via clathrin in a SMAP1, a clathrin-interacting GTPase-activating protein (GAP) dependent manner.Citation73 Clathrin pits are also implicated in the autophagy mediated connexin Cx31.1 degradation.Citation74 Autophagy machinery also regulates caveolae. Under basal conditions, caveolin-1 (Cav-1) binds to ATG5 and ATG12 in the cytosol while formation of ATG5-ATG12 complex during starvation leads to rapid dissociation of Cav-1 from ATG5-ATG12 conjugates.Citation75 Moreover, sustained autophagy is known to reduce Cav-1 mRNA level.Citation76 Cav-1 deficiency also promotes both basal and inducible autophagy, particularly by increasing lysosomal function and autophagosome-lysosome fusion as a cell survival mechanism under starvation.Citation77 TJ protein occludin,Citation78-80 AJ protein β-catenin,Citation81,82 and GJ protein connexinsCitation83 are known to be associated with caveolins. Macropinocytosis is another mode of vesicular trafficking of junctional proteinsCitation84 that is regulated by autophagy. LC3 (Light chain 3), a component of autophagosomes, is recruited to macropinosomes via lipidation by ATG5 and ATG7, and the class III phosphatidylinositol-3-kinase VPS34, facilitating lysosomal degradation of internalized cargo.Citation85
The autophagy regulation of endocytic processes assumes greater relevance in the context of the larger question about the origin of the autophagosomal membrane. Based upon the location of enrichment of scaffolding ATG proteins with specific lipid-modifying enzymes that promotes autophagosomal membrane formation,Citation86 the origin of the autophagosomal membrane has been traced to the endoplasmic reticulum,Citation87 mitochondria,Citation88 and plasma membrane.Citation89 In case of plasma membrane-originated autophagosomal membrane, the contribution of endocytic clathrin machinery for the assembly of the autophagosome-limiting membranes has already been demonstrated.Citation89 The key questions that need to be answered include the following: what kind of autophagic stimulus dictates plasma membrane-derived autophagosome formation and how specific junctional proteins are targeted to the autophagosomes assuming they are not moved passively along with the plasma membrane fractions. One such clue has already been revealed regarding the autophagy-mediated degradation of gap junctions. E3 ubiquitin-protein ligase Nedd4-mediated ubiquitinylation of the connexin molecule and recruitment of the adaptor protein Eps15 to the GJ has been shown to be required for the initiation of autophagy-dependent internalization and degradation of connexin 43.Citation90
Autophagy, Tight junctions, and inflammatory bowel disease
Defects in intestinal TJ barrier,Citation4,72,73 AJ,Citation91 and GJCitation92 are associated with intestinal disorders and inflammatory bowel diseases (IBD) including Crohn disease and ulcerative colitis. The defects in intestinal TJ barrier allows increased antigenic penetration, resulting in amplified inflammatory response in intestinal tissue.Citation4,93 Genome wide association studies have identified mutations in autophagy related genes ATG16L1 and IRGM as substantiated risk factors for Crohn disease (CD).Citation94-96 Recent studies have also shown the role of autophagy in dendritic-epithelial cell interactions, NOD2 directed bacterial sensing, and immune mediated clearance which is considered to be important in pathogenesis of IBD.Citation27-30 In mice, Atg4b-deficiency caused increased susceptibility to dextran sulfate sodium (DSS)-induced colitis with alterations in pro-inflammatory cytokine profiles and Paneth cell abnormalities.Citation97 On the other hand, autophagy enhancement ameliorated intestinal inflammation in IL-10 knockout mouse model of spontaneous enterocolitis.Citation98,99 Clinical data and animal studies show a direct link between defective intestinal TJ barrier and intestinal inflammation in IBD patients and animal models of IBD.Citation100-102 Previous study from our laboratory showed that starvation-induced autophagy reduced TJ permeability of the intestinal epithelial cells, indicating a role for autophagy in the maintenance of intestinal barrier function.Citation37 Moreover, we have observed that acute deletion of Atg7, which is critical for the autophagy conjugation system and formation of autophagosomes,Citation103 in adult mice exacerbate DSS-induced increase in colonic TJ permeability, disease activity, and colonic inflammation (unpublished data). Thus the role of autophagy in the maintenance of intestinal TJ barrier appears to be a critical mechanism in prevention of intestinal inflammation.
Perspective
In summary, various autophagic responses regulate epithelial junctions to maintain cell homeostasis (). Autophagy enhances TJ barrier function and reduces paracellular permeability by modulating TJ protein composition. At AJ, autophagy promotes E-cadherin expression and regulates cell proliferation and migration. Sustained autophagy also regulates cell growth and proliferation via degradation of AJ protein β-catenin. Autophagy-mediated connexin (GJ) degradation helps cell adaptation in the event of stress. These autophagic responses however may have context dependent outcomes and not globally uniform consequences. The role of autophagy in epithelial cell junction regulation has been deduced using pharmacological or genetic modulation of ATG proteins in stress or injury models. Further studies are warranted to identify specific autophagy regulators of epithelial cell junctions. The advances in the understanding of the intricate role of autophagy in the regulation of epithelial junctions will help rationalize and improve therapeutic efforts toward the clinical problems ranging from diabetic retinopathy, edema, jaundice, and diarrhea to autoimmune diseases, cancer metastasis, and infectious diseases.
Figure 2. Summary of the cell homeostatic effects of autophagy on the epithelial cell junctions. Various positive or inhibitory regulators of authophagic effects on cell junctions are listed. The backdrop shows transmission electron micrograph of mouse small intestinal tight junction and adherens junction.
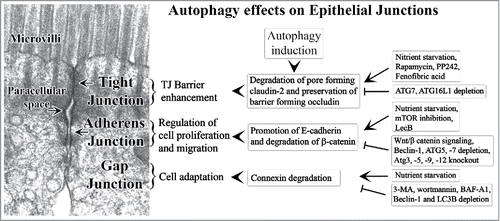
Abbreviations
AGJ | = | annular gap junction |
AJ | = | adherens junction |
Akt | = | Protein kinase B |
AML | = | acute myeloid leukemia |
AMPK | = | 5′ adenosine monophosphate-activated protein kinase |
BMVEC | = | brain microvascular endothelial cell |
Cav-1 | = | caveolin1 |
CD | = | Crohn disease |
Eps15 | = | Epidermal growth factor receptor substrate 15 |
GJ | = | gap junction |
HMEC | = | human microvascular endothelial cell line |
IBD | = | inflammatory bowel disease |
LC3 | = | light chain 3 |
MAPK | = | Mitogen-activated protein kinases |
MIF | = | macrophage migration inhibitory factor |
Mtor | = | mammalian target of rapamycin |
NEAA | = | non-essential amino acids |
NEC | = | necrotizing enterocolitis |
OGD/R | = | oxygen-glucose deprivation/reoxygenation |
SMAP1 | = | Stromal Membrane-Associated Protein 1 |
SQSTM1 | = | Sequestosome 1 |
TJ | = | tight junction |
TER | = | transepithelial resistance |
Disclosure of potential conflicts of interest
No potential conflicts of interest were disclosed.
Funding
This research work has been supported in part by National Institute of Diabetes and Digestive and Kidney Diseases Grants (to T. Ma) and K01-DK-100562-02 (to P. Nighot) and a Veterans Affairs (VA) Merit Review grant from the VA Research Service (to T. Ma).
References
- Giepmans BN, van Ijzendoorn SC. Epithelial cell-cell junctions and plasma membrane domains. Biochim Biophys Acta 2009; 1788:820-31; PMID:18706883; http://dx.doi.org/10.1016/j.bbamem.2008.07.015
- Mandel LJ, Bacallao R, Zampighi G. Uncoupling of the molecular [fence] and paracellular [gate] functions in epithelial tight junctions. Nature 1993; 361:552-5; PMID:8429911; http://dx.doi.org/10.1038/361552a0
- Podolsky DK. Healing the epithelium: solving the problem from two sides. J Gastroenterol 1997; 32:122-6; PMID:9058308; http://dx.doi.org/10.1007/BF01213309
- Ma TY, Anderson JM. Tight junction and intestinal barrier. In: Johnson LR, ed. Textbook of gastrointestinal physiology: Elsevier Health Sciences, Philadelphia, PA, 2006:1559-94.
- Zhang Y, Sivasankar S, Nelson WJ, Chu S. Resolving cadherin interactions and binding cooperativity at the single-molecule level. Proc Natl Acad Sci U S A 2009; 106:109-14; PMID:19114658; http://dx.doi.org/10.1073/pnas.0811350106
- Troyanovsky S. Cadherin dimers in cell-cell adhesion. Eur J Cell Biol 2005; 84:225-33; PMID:15819403; http://dx.doi.org/10.1016/j.ejcb.2004.12.009
- Watabe-Uchida M, Uchida N, Imamura Y, Nagafuchi A, Fujimoto K, Uemura T, Vermeulen S, van Roy F, Adamson ED, Takeichi M. alpha-Catenin-vinculin interaction functions to organize the apical junctional complex in epithelial cells. J Cell Biol 1998; 142:847-57; PMID:9700171; http://dx.doi.org/10.1083/jcb.142.3.847
- Kobielak A, Pasolli HA, Fuchs E. Mammalian formin-1 participates in adherens junctions and polymerization of linear actin cables. Nat Cell Biol 2004; 6:21-30; PMID:14647292; http://dx.doi.org/10.1038/ncb1075
- Abe K, Takeichi M. EPLIN mediates linkage of the cadherin catenin complex to F-actin and stabilizes the circumferential actin belt. Proc Natl Acad Sci U S A 2008; 105:13-9; PMID:18093941; http://dx.doi.org/10.1073/pnas.0710504105
- Ivanov AI, McCall IC, Babbin B, Samarin SN, Nusrat A, Parkos CA. Microtubules regulate disassembly of epithelial apical junctions. BMC Cell Biol 2006; 7:12; PMID:16509970; http://dx.doi.org/10.1186/1471-2121-7-12
- Meng W, Mushika Y, Ichii T, Takeichi M. Anchorage of microtubule minus ends to adherens junctions regulates epithelial cell-cell contacts. Cell 2008; 135:948-59; PMID:19041755; http://dx.doi.org/10.1016/j.cell.2008.09.040
- Takai Y, Irie K, Shimizu K, Sakisaka T, Ikeda W. Nectins and nectin-like molecules: roles in cell adhesion, migration, and polarization. Cancer Sci 2003; 94:655-67; PMID:12901789; http://dx.doi.org/10.1111/j.1349-7006.2003.tb01499.x
- Klezovitch O, Vasioukhin V. Cadherin signaling: keeping cells in touch. F1000Res 2015; 4:550; PMID:26339481
- White TW, Paul DL. Genetic diseases and gene knockouts reveal diverse connexin functions. Annu Rev Physiol 1999; 61:283-310; PMID:10099690; http://dx.doi.org/10.1146/annurev.physiol.61.1.283
- Vinken M, Vanhaecke T, Papeleu P, Snykers S, Henkens T, Rogiers V. Connexins and their channels in cell growth and cell death. Cell Signal 2006; 18:592-600; PMID:16183253; http://dx.doi.org/10.1016/j.cellsig.2005.08.012
- Mruk DD, Lau AS, Conway AM. Crosstalk between Rab GTPases and cell junctions. Contraception 2005; 72:280-90; PMID:16181972; http://dx.doi.org/10.1016/j.contraception.2005.03.013
- Yu D, Turner JR. Stimulus-induced reorganization of tight junction structure: the role of membrane traffic. Biochimica et biophysica acta 2008; 1778:709-16; PMID:17915190; http://dx.doi.org/10.1016/j.bbamem.2007.07.027
- Shen L, Weber CR, Turner JR. The tight junction protein complex undergoes rapid and continuous molecular remodeling at steady state. J Cell Biol 2008; 181:683-95; PMID:18474622; http://dx.doi.org/10.1083/jcb.200711165
- Le TL, Yap AS, Stow JL. Recycling of E-cadherin: a potential mechanism for regulating cadherin dynamics. J Cell Biol 1999; 146:219-32; PMID:10402472
- Ivanov AI, Nusrat A, Parkos CA. Endocytosis of epithelial apical junctional proteins by a clathrin-mediated pathway into a unique storage compartment. Mol Biol Cell 2004; 15:176-88; PMID:14528017; http://dx.doi.org/10.1091/mbc.E03-05-0319
- Meng W, Takeichi M. Adherens junction: molecular architecture and regulation. Cold Spring Harb Perspect Biol 2009; 1:a002899; http://dx.doi.org/10.1101/cshperspect.a002899
- Ivanov AI, Naydenov NG. Dynamics and regulation of epithelial adherens junctions: recent discoveries and controversies. Int Rev Cell Mol Biol 2013; 303:27-99; PMID:23445808; http://dx.doi.org/10.1016/B978-0-12-407697-6.00002-7
- Mese G, Richard G, White TW. Gap junctions: basic structure and function. J Invest Dermatol 2007; 127:2516-24; PMID:17934503; http://dx.doi.org/10.1038/sj.jid.5700770
- Boya P, Reggiori F, Codogno P. Emerging regulation and functions of autophagy. Nat Cell Biol 2013; 15:713-20; PMID:23817233; http://dx.doi.org/10.1038/ncb2788
- Deretic V, Saitoh T, Akira S. Autophagy in infection, inflammation and immunity. Nat Rev Immunol 2013; 13:722-37; PMID:24064518; http://dx.doi.org/10.1038/nri3532
- Levine B, Kroemer G. Autophagy in the pathogenesis of disease. Cell 2008; 132:27-42; PMID:18191218; http://dx.doi.org/10.1016/j.cell.2007.12.018
- Strisciuglio C, Duijvestein M, Verhaar AP, Vos AC, van den Brink GR, Hommes DW, Wildenberg ME. Impaired autophagy leads to abnormal dendritic cell-epithelial cell interactions. J Crohns Colitis 2013; 7:534-41; PMID:22981596; http://dx.doi.org/10.1016/j.crohns.2012.08.009
- Saitoh T, Fujita N, Jang MH, Uematsu S, Yang BG, Satoh T, Omori H, Noda T, Yamamoto N, Komatsu M, et al. Loss of the autophagy protein Atg16L1 enhances endotoxin-induced IL-1beta production. Nature 2008; 456:264-8; PMID:18849965; http://dx.doi.org/10.1038/nature07383
- Wildenberg ME, Vos AC, Wolfkamp SC, Duijvestein M, Verhaar AP, Te Velde AA, van den Brink GR, Hommes DW. Autophagy attenuates the adaptive immune response by destabilizing the immunologic synapse. Gastroenterology 2012; 142:1493-503.e6; PMID:22370477; http://dx.doi.org/10.1053/j.gastro.2012.02.034
- Cooney R, Baker J, Brain O, Danis B, Pichulik T, Allan P, Ferguson DJ, Campbell BJ, Jewell D, Simmons A. NOD2 stimulation induces autophagy in dendritic cells influencing bacterial handling and antigen presentation. Nat Med 2010; 16:90-7; PMID:19966812; http://dx.doi.org/10.1038/nm.2069
- Mizushima N. Autophagy: process and function. Genes Dev 2007; 21:2861-73; PMID:18006683; http://dx.doi.org/10.1101/gad.1599207
- Duran JM, Anjard C, Stefan C, Loomis WF, Malhotra V. Unconventional secretion of Acb1 is mediated by autophagosomes. J Cell Biol 2010; 188:527-36; PMID:20156967; http://dx.doi.org/10.1083/jcb.200911154
- Dupont N, Jiang S, Pilli M, Ornatowski W, Bhattacharya D, Deretic V. Autophagy-based unconventional secretory pathway for extracellular delivery of IL-1beta. EMBO J 2011; 30:4701-11; PMID:22068051; http://dx.doi.org/10.1038/emboj.2011.398
- DeSelm CJ, Miller BC, Zou W, Beatty WL, van Meel E, Takahata Y, Klumperman J, Tooze SA, Teitelbaum SL, Virgin HW. Autophagy proteins regulate the secretory component of osteoclastic bone resorption. Dev Cell 2011; 21:966-74; PMID:22055344; http://dx.doi.org/10.1016/j.devcel.2011.08.016
- Pavlides S, Vera I, Gandara R, Sneddon S, Pestell RG, Mercier I, Martinez-Outschoorn UE, Whitaker-Menezes D, Howell A, Sotgia F, et al. Warburg meets autophagy: cancer-associated fibroblasts accelerate tumor growth and metastasis via oxidative stress, mitophagy, and aerobic glycolysis. Antioxid Redox Signal 2012; 16:1264-84; PMID:21883043; http://dx.doi.org/10.1089/ars.2011.4243
- Capparelli C, Guido C, Whitaker-Menezes D, Bonuccelli G, Balliet R, Pestell TG, Goldberg AF, Pestell RG, Howell A, Sneddon S, et al. Autophagy and senescence in cancer-associated fibroblasts metabolically supports tumor growth and metastasis via glycolysis and ketone production. Cell Cycle 2012; 11:2285-302; PMID:22684298; http://dx.doi.org/10.4161/cc.20718
- Nighot PK, Hu CA, Ma TY. Autophagy enhances intestinal epithelial tight junction barrier function by targeting claudin-2 protein degradation. J Biol Chem 2015; 290:7234-46; PMID:25616664; http://dx.doi.org/10.1074/jbc.M114.597492
- Shen L, Weber CR, Raleigh DR, Yu D, Turner JR. Tight junction pore and leak pathways: a dynamic duo. Annu Rev Physiol 2011; 73:283-309; PMID:20936941; http://dx.doi.org/10.1146/annurev-physiol-012110-142150
- Yamamoto A, Tagawa Y, Yoshimori T, Moriyama Y, Masaki R, Tashiro Y. Bafilomycin A1 prevents maturation of autophagic vacuoles by inhibiting fusion between autophagosomes and lysosomes in rat hepatoma cell line, H-4-II-E cells. Cell structure and function 1998; 23:33-42; PMID:9639028; http://dx.doi.org/10.1247/csf.23.33
- Rubinsztein DC, Gestwicki JE, Murphy LO, Klionsky DJ. Potential therapeutic applications of autophagy. Nat Rev Drug Discov 2007; 6:304-12; http://dx.doi.org/10.1038/nrd2272
- Blommaart EF, Krause U, Schellens JP, Vreeling-Sindelarova H, Meijer AJ. The phosphatidylinositol 3-kinase inhibitors wortmannin and LY294002 inhibit autophagy in isolated rat hepatocytes. Eur J Biochem 1997; 243:240-6; PMID:9030745; http://dx.doi.org/10.1111/j.1432-1033.1997.0240a.x
- Yang Y, Li W, Sun Y, Han F, Hu CA, Wu Z. Amino acid deprivation disrupts barrier function and induces protective autophagy in intestinal porcine epithelial cells. Amino acids 2015; 47:2177-84; PMID:25287255; http://dx.doi.org/10.1007/s00726-014-1844-6
- Duan JL, Yin J, Ren WK, Wu MM, Chen S, Cui ZJ, Wu X, Huang RL, Li TJ, Yin YL. Pyrrolidine dithiocarbamate restores gastric damages and suppressive autophagy induced by hydrogen peroxide. Free Radic Res 2015; 49:210-8; PMID:25471085; http://dx.doi.org/10.3109/10715762.2014.993627
- Li H, Gao A, Feng D, Wang Y, Zhang L, Cui Y, Li B, Wang Z, Chen G. Evaluation of the protective potential of brain microvascular endothelial cell autophagy on blood-brain barrier integrity during experimental cerebral ischemia-reperfusion injury. Transl Stroke Res 2014; 5:618-26; PMID:25070048; http://dx.doi.org/10.1007/s12975-014-0354-x
- Chen HR, Chuang YC, Chao CH, Yeh TM. Macrophage migration inhibitory factor induces vascular leakage via autophagy. Biology open 2015; 4:244-52; PMID:25617421; http://dx.doi.org/10.1242/bio.201410322
- Liu J, Weaver J, Jin X, Zhang Y, Xu J, Liu KJ, Li W, Liu W. Nitric Oxide Interacts with Caveolin-1 to Facilitate Autophagy-Lysosome-Mediated Claudin-5 Degradation in Oxygen-Glucose Deprivation-Treated Endothelial Cells. Mol Neurobiol 2015; 29 Oct; http://dx.doi.org/10.1007/s12035-015-9504-8.
- Miranda S, Gonzalez-Rodriguez A, Garcia-Ramirez M, Revuelta-Cervantes J, Hernandez C, Simo R, Valverde AM. Beneficial effects of fenofibrate in retinal pigment epithelium by the modulation of stress and survival signaling under diabetic conditions. J Cell Physiol 2012; 227:2352-62; PMID:21826649; http://dx.doi.org/10.1002/jcp.22970
- Kühn K, Cott C, Bohler S, Aigal S, Zheng S, Villringer S, Imberty A, Claudinon J, Römer W. The interplay of autophagy and β-Catenin signaling regulates differentiation in acute myeloid leukemia. Cell Death Discovery 2015; 1:15031; http://dx.doi.org/10.1038/cddiscovery.2015.31
- Isakson P, Bjoras M, Boe SO, Simonsen A. Autophagy contributes to therapy-induced degradation of the PML/RARA oncoprotein. Blood 2010; 116:2324-31; PMID:20574048; http://dx.doi.org/10.1182/blood-2010-01-261040
- Kuhn K, Romer W. Considering autophagy, beta-Catenin and E-Cadherin as innovative therapy aspects in AML. Cell Death Dis 2015; 6:e1950; PMID:26512960; http://dx.doi.org/10.1038/cddis.2015.314
- Petherick KJ, Williams AC, Lane JD, Ordonez-Moran P, Huelsken J, Collard TJ, Smartt HJ, Batson J, Malik K, Paraskeva C, et al. Autolysosomal beta-catenin degradation regulates Wnt-autophagy-p62 crosstalk. EMBO J 2013; 32:1903-16; PMID:23736261; http://dx.doi.org/10.1038/emboj.2013.123
- Catalano M, D'Alessandro G, Lepore F, Corazzari M, Caldarola S, Valacca C, Faienza F, Esposito V, Limatola C, Cecconi F, et al. Autophagy induction impairs migration and invasion by reversing EMT in glioblastoma cells. Mol Oncol 2015; 9:1612-25; PMID:26022108; http://dx.doi.org/10.1016/j.molonc.2015.04.016
- Qiang L, Zhao B, Ming M, Wang N, He TC, Hwang S, Thorburn A, He YY. Regulation of cell proliferation and migration by p62 through stabilization of Twist1. Proc Natl Acad Sci U S A 2014; 111:9241-6; PMID:24927592; http://dx.doi.org/10.1073/pnas.1322913111
- Carette D, Gilleron J, Denizot JP, Grant K, Pointis G, Segretain D. New cellular mechanisms of gap junction degradation and recycling. Biol Cell 2015; 107:218-31; PMID:25818265; http://dx.doi.org/10.1111/boc.201400048
- Falk MM, Fong JT, Kells RM, O'Laughlin MC, Kowal TJ, Thévenin AF. Degradation of endocytosed gap junctions by autophagosomal and endo-/lysosomal pathways: a perspective. J Membr Biol 2012; 245:465-76; PMID:22825714; http://dx.doi.org/10.1007/s00232-012-9464-0
- Hesketh GG, Shah MH, Halperin VL, Cooke CA, Akar FG, Yen TE, Kass DA, Machamer CE, Van Eyk JE, Tomaselli GF. Ultrastructure and regulation of lateralized connexin43 in the failing heart. Circ Res 2010; 106:1153-63; PMID:20167932; http://dx.doi.org/10.1161/CIRCRESAHA.108.182147
- Lichtenstein A, Minogue PJ, Beyer EC, Berthoud VM. Autophagy: a pathway that contributes to connexin degradation. J Cell Sci 2011; 124:910-20; PMID:21378309; http://dx.doi.org/10.1242/jcs.073072
- Sun LQ, Gao JL, Cui Y, Zhao MM, Jing XB, Li R, Tian YX, Cui JZ, Wu ZX. Neuronic autophagy contributes to p-connexin 43 degradation in hippocampal astrocytes following traumatic brain injury in rats. Mol Med Rep 2015; 11:4419-23; PMID:25626126
- Bejarano E, Yuste A, Patel B, Stout RF, Jr., Spray DC, Cuervo AM. Connexins modulate autophagosome biogenesis. Nat Cell Biol 2014; 16:401-14; PMID:24705551; http://dx.doi.org/10.1038/ncb2934
- Fong JT, Kells RM, Gumpert AM, Marzillier JY, Davidson MW, Falk MM. Internalized gap junctions are degraded by autophagy. Autophagy 2012; 8:794-811; PMID:22635056; http://dx.doi.org/10.4161/auto.19390
- Thorburn A. Autophagy and its effects: making sense of double-edged swords. PLoS Biol 2014; 12:e1001967; PMID:25313680; http://dx.doi.org/10.1371/journal.pbio.1001967
- Tittarelli A, Janji B, Van Moer K, Noman MZ, Chouaib S. The Selective Degradation of Synaptic Connexin 43 Protein by Hypoxia-induced Autophagy Impairs Natural Killer Cell-mediated Tumor Cell Killing. J Biol Chem 2015; 290:23670-9; PMID:26221040; http://dx.doi.org/10.1074/jbc.M115.651547
- Yu Y, Shiou SR, Guo Y, Lu L, Westerhoff M, Sun J, Petrof EO, Claud EC. Erythropoietin protects epithelial cells from excessive autophagy and apoptosis in experimental neonatal necrotizing enterocolitis. PLoS One 2013; 8:e69620; PMID:23936061; http://dx.doi.org/10.1371/journal.pone.0069620
- Wang J, Whiteman MW, Lian H, Wang G, Singh A, Huang D, Denmark T. A non-canonical MEK/ERK signaling pathway regulates autophagy via regulating Beclin 1. J Biol Chem 2009; 284:21412-24; PMID:19520853; http://dx.doi.org/10.1074/jbc.M109.026013
- Mok KW, Mruk DD, Lee WM, Cheng CY. Rictor/mTORC2 regulates blood-testis barrier dynamics via its effects on gap junction communications and actin filament network. FASEB J 2013; 27:1137-52; PMID:23288930; http://dx.doi.org/10.1096/fj.12-212977
- Mok KW, Mruk DD, Cheng CY. Regulation of blood-testis barrier (BTB) dynamics during spermatogenesis via the “Yin” and “Yang” effects of mammalian target of rapamycin complex 1 (mTORC1) and mTORC2. Int Rev Cell Mol Biol 2013; 301:291-358; PMID:23317821; http://dx.doi.org/10.1016/B978-0-12-407704-1.00006-3
- Barker G, Simmons NL. Identification of two strains of cultured canine renal epithelial cells (MDCK cells) which display entirely different physiological properties. Quarterly journal of experimental physiology (Cambridge, England) 1981; 66:61-72; PMID:6911762; http://dx.doi.org/10.1113/expphysiol.1981.sp002529
- Furuse M, Furuse K, Sasaki H, Tsukita S. Conversion of zonulae occludentes from tight to leaky strand type by introducing claudin-2 into Madin-Darby canine kidney I cells. J Cell Biol 2001; 153:263-72; PMID:11309408; http://dx.doi.org/10.1083/jcb.153.2.263
- Scharl M, Paul G, Barrett KE, McCole DF. AMP-activated protein kinase mediates the interferon-gamma-induced decrease in intestinal epithelial barrier function. J Biol Chem 2009; 284:27952-63; PMID:19654324; http://dx.doi.org/10.1074/jbc.M109.046292
- Tang XX, Chen H, Yu S, Zhang L, Caplan MJ, Chan HC. Lymphocytes accelerate epithelial tight junction assembly: role of AMP-activated protein kinase (AMPK). PLoS One 2010; 5:e12343; PMID:20808811; http://dx.doi.org/10.1371/journal.pone.0012343
- Tian Y, Chang JC, Fan EY, Flajolet M, Greengard P. Adaptor complex AP2/PICALM, through interaction with LC3, targets Alzheimer's APP-CTF for terminal degradation via autophagy. Proc Natl Acad Sci U S A 2013; 110:17071-6; PMID:24067654; http://dx.doi.org/10.1073/pnas.1315110110
- Ikari A, Takiguchi A, Atomi K, Sugatani J. Epidermal growth factor increases clathrin-dependent endocytosis and degradation of claudin-2 protein in MDCK II cells. J Cell Physiol 2011; 226:2448-56; PMID:21660968; http://dx.doi.org/10.1002/jcp.22590
- Kon S, Tanabe K, Watanabe T, Sabe H, Satake M. Clathrin dependent endocytosis of E-cadherin is regulated by the Arf6GAP isoform SMAP1. Exp Cell Res 2008; 314:1415-28; PMID:18331728; http://dx.doi.org/10.1016/j.yexcr.2007.11.006
- Zhu X, Ruan Z, Yang X, Chu K, Wu H, Li Y, Huang Y. Connexin 31.1 degradation requires the Clathrin-mediated autophagy in NSCLC cell H1299. J Cell Mol Med 2015; 19:257-64; PMID:25388970; http://dx.doi.org/10.1111/jcmm.12470
- Chen ZH, Cao JF, Zhou JS, Liu H, Che LQ, Mizumura K, Li W, Choi AM, Shen HH. Interaction of caveolin-1 with ATG12-ATG5 system suppresses autophagy in lung epithelial cells. Am J Physiol Lung Cell Mol Physiol 2014; 306:L1016-25; http://dx.doi.org/10.1152/ajplung.00268.2013
- Engelman JA, Chu C, Lin A, Jo H, Ikezu T, Okamoto T, Kohtz DS, Lisanti MP. Caveolin-mediated regulation of signaling along the p42/44 MAP kinase cascade in vivo. A role for the caveolin-scaffolding domain. FEBS Lett 1998; 428:205-11; PMID:9654135; http://dx.doi.org/10.1016/S0014-5793(98)00470-0
- Shi Y, Tan SH, Ng S, Zhou J, Yang ND, Koo GB, McMahon KA, Parton RG, Hill MM, Del Pozo MA, et al. Critical role of CAV1/caveolin-1 in cell stress responses in human breast cancer cells via modulation of lysosomal function and autophagy. Autophagy 2015; 11:769-84; PMID:25945613; http://dx.doi.org/10.1080/15548627.2015.1034411
- Marchiando AM, Shen L, Graham WV, Weber CR, Schwarz BT, Austin JR, 2nd, Raleigh DR, Guan Y, Watson AJ, Montrose MH, et al. Caveolin-1-dependent occludin endocytosis is required for TNF-induced tight junction regulation in vivo. J Cell Biol 2010; 189:111-26; PMID:20351069; http://dx.doi.org/10.1083/jcb.200902153
- Nighot PK, Blikslager AT. Chloride channel ClC-2 modulates tight junction barrier function via intracellular trafficking of occludin. Am J Physiol Cell Physiol 2012; 302:C178-87; http://dx.doi.org/10.1152/ajpcell.00072.2011
- Itallie CM, Anderson JM. Caveolin binds independently to claudin-2 and occludin. Ann N Y Acad Sci 2012; 1257:103-7; PMID:22671595; http://dx.doi.org/10.1111/j.1749-6632.2012.06535.x
- Pellon-Cardenas O, Schweitzer J, D'Souza-Schorey C. Endocytic trafficking and Wnt/beta-catenin signaling. Curr Drug Targets 2011; 12:1216-22; PMID:21561414; http://dx.doi.org/10.2174/138945011795906552
- Galbiati F, Volonte D, Brown AM, Weinstein DE, Ben-Ze'ev A, Pestell RG, Lisanti MP. Caveolin-1 expression inhibits Wnt/beta-catenin/Lef-1 signaling by recruiting beta-catenin to caveolae membrane domains. J Biol Chem 2000; 275:23368-77; PMID:10816572; http://dx.doi.org/10.1074/jbc.M002020200
- Schubert AL, Schubert W, Spray DC, Lisanti MP. Connexin family members target to lipid raft domains and interact with caveolin-1. Biochemistry 2002; 41:5754-64; PMID:11980479; http://dx.doi.org/10.1021/bi0121656
- Bruewer M, Utech M, Ivanov AI, Hopkins AM, Parkos CA, Nusrat A. Interferon-gamma induces internalization of epithelial tight junction proteins via a macropinocytosis-like process. FASEB J 2005; 19:923-33; http://dx.doi.org/10.1096/fj.04-3260com
- Florey O, Kim SE, Sandoval CP, Haynes CM, Overholtzer M. Autophagy machinery mediates macroendocytic processing and entotic cell death by targeting single membranes. Nat Cell Biol 2011; 13:1335-43; PMID:22002674; http://dx.doi.org/10.1038/ncb2363
- Cuervo AM. The plasma membrane brings autophagosomes to life. Nat Cell Biol 2010; 12:735-7; PMID:20680002; http://dx.doi.org/10.1038/ncb0810-735
- Axe EL, Walker SA, Manifava M, Chandra P, Roderick HL, Habermann A, Griffiths G, Ktistakis NT. Autophagosome formation from membrane compartments enriched in phosphatidylinositol 3-phosphate and dynamically connected to the endoplasmic reticulum. J Cell Biol 2008; 182:685-701; PMID:18725538; http://dx.doi.org/10.1083/jcb.200803137
- Hailey DW, Rambold AS, Satpute-Krishnan P, Mitra K, Sougrat R, Kim PK, Lippincott-Schwartz J. Mitochondria supply membranes for autophagosome biogenesis during starvation. Cell 2010; 141:656-67; PMID:20478256; http://dx.doi.org/10.1016/j.cell.2010.04.009
- Ravikumar B, Moreau K, Jahreiss L, Puri C, Rubinsztein DC. Plasma membrane contributes to the formation of pre-autophagosomal structures. Nat Cell Biol 2010; 12:747-57; PMID:20639872; http://dx.doi.org/10.1038/ncb2078
- Bejarano E, Girao H, Yuste A, Patel B, Marques C, Spray DC, Pereira P, Cuervo AM. Autophagy modulates dynamics of connexins at the plasma membrane in a ubiquitin-dependent manner. Mol Biol Cell 2012; 23:2156-69; PMID:22496425; http://dx.doi.org/10.1091/mbc.E11-10-0844
- Mehta S, Nijhuis A, Kumagai T, Lindsay J, Silver A. Defects in the adherens junction complex (E-cadherin/ beta-catenin) in inflammatory bowel disease. Cell Tissue Res 2015; 360:749-60; PMID:25238996; http://dx.doi.org/10.1007/s00441-014-1994-6
- Perez White BE, Getsios S. Eph receptor and ephrin function in breast, gut, and skin epithelia. Cell Adh Migr 2014; 8:327-38; PMID:25482622; http://dx.doi.org/10.4161/19336918.2014.970012
- Turner JR. Intestinal mucosal barrier function in health and disease. Nat Rev Immunol 2009; 9:799-809.
- Hampe J, Franke A, Rosenstiel P, Till A, Teuber M, Huse K, Albrecht M, Mayr G, De La Vega FM, Briggs J, et al. A genome-wide association scan of nonsynonymous SNPs identifies a susceptibility variant for Crohn disease in ATG16L1. Nat Genet 2007; 39:207-11; PMID:17200669; http://dx.doi.org/10.1038/ng1954
- Rioux JD, Xavier RJ, Taylor KD, Silverberg MS, Goyette P, Huett A, Green T, Kuballa P, Barmada MM, Datta LW, et al. Genome-wide association study identifies new susceptibility loci for Crohn disease and implicates autophagy in disease pathogenesis. Nat Genet 2007; 39:596-604; PMID:17435756; http://dx.doi.org/10.1038/ng2032
- Parkes M, Barrett JC, Prescott NJ, Tremelling M, Anderson CA, Fisher SA, Roberts RG, Nimmo ER, Cummings FR, Soars D, et al. Sequence variants in the autophagy gene IRGM and multiple other replicating loci contribute to Crohn's disease susceptibility. Nat Genet 2007; 39:830-2; PMID:17554261; http://dx.doi.org/10.1038/ng2061
- Cabrera S, Fernandez AF, Marino G, Aguirre A, Suarez MF, Espanol Y, Vega JA, Laura R, Fueyo A, Fernandez-Garcia MS, et al. ATG4B/autophagin-1 regulates intestinal homeostasis and protects mice from experimental colitis. Autophagy 2013; 9:1188-200; PMID:23782979; http://dx.doi.org/10.4161/auto.24797
- Zhao J, Dong JN, Wang HG, Zhao M, Sun J, Zhu WM, Zuo LG, Gong JF, Li Y, Gu LL, et al. Docosahexaenoic Acid Attenuated Experimental Chronic Colitis in Interleukin 10-Deficient Mice by Enhancing Autophagy Through Inhibition of the mTOR Pathway. JPEN J Parenter Enteral Nutr 2015; 25 Sept; http://dx.doi.org/10.1177/0148607115609308
- Zhao J, Sun Y, Shi P, Dong JN, Zuo LG, Wang HG, Gong JF, Li Y, Gu LL, Li N, et al. Celastrol ameliorates experimental colitis in IL-10 deficient mice via the up-regulation of autophagy. Int Immunopharmacol 2015; 26:221-8; PMID:25858875; http://dx.doi.org/10.1016/j.intimp.2015.03.033
- Wyatt J, Vogelsang H, Hubl W, Waldhoer T, Lochs H. Intestinal permeability and the prediction of relapse in Crohn's disease. Lancet 1993; 341:1437-9; PMID:8099141; http://dx.doi.org/10.1016/0140-6736(93)90882-H
- Hollander D, Vadheim CM, Brettholz E, Petersen GM, Delahunty T, Rotter JI. Increased intestinal permeability in patients with Crohn's disease and their relatives. A possible etiologic factor. Ann Internal Med 1986; 105:883-5; PMID:3777713; http://dx.doi.org/10.7326/0003-4819-105-6-883
- Buhner S, Buning C, Genschel J, Kling K, Herrmann D, Dignass A, Kuechler I, Krueger S, Schmidt HH, Lochs H. Genetic basis for increased intestinal permeability in families with Crohn's disease: role of CARD15 3020insC mutation? Gut 2006; 55:342-7; PMID:16000642; http://dx.doi.org/10.1136/gut.2005.065557
- Komatsu M, Waguri S, Ueno T, Iwata J, Murata S, Tanida I, Ezaki J, Mizushima N, Ohsumi Y, Uchiyama Y, et al. Impairment of starvation-induced and constitutive autophagy in Atg7-deficient mice. J Cell Biol 2005; 169:425-34; PMID:15866887; http://dx.doi.org/10.1083/jcb.200412022