ABSTRACT
Lipid mediators play a critical role in the development and resolution of vascular endothelial barrier dysfunction caused by various pathologic interventions. The accumulation of excess lipids directly impairs endothelial cell (EC) barrier function that is known to contribute to the development of atherosclerosis and metabolic disorders such as obesity and diabetes as well as chronic inflammation in the vascular endothelium. Certain products of phospholipid oxidation (OxPL) such as fragmented phospholipids generated during oxidative and nitrosative stress show pro-inflammatory potential and cause endothelial barrier dysfunction. In turn, other OxPL products enhance basal EC barrier and exhibit potent barrier-protective effects in pathologic settings of acute vascular leak caused by pro-inflammatory mediators, barrier disruptive agonists and pathologic mechanical stimulation. These beneficial effects were further confirmed in rodent models of lung injury and inflammation. The bioactive oxidized lipid molecules may serve as important therapeutic prototype molecules for future treatment of acute lung injury syndromes associated with endothelial barrier dysfunction and inflammation. This review will summarize recent studies of biological effects exhibited by various groups of lipid mediators with a focus on the role of oxidized phospholipids in control of vascular endothelial barrier, agonist induced EC permeability, inflammation, and barrier recovery related to clinical settings of acute lung injury and inflammatory vascular leak.
Introduction
The endothelial barrier provides a selective permeability to fluids and solutes between the blood and tissues and any alteration in this precisely regulated barrier integrity leads to many pathological conditions including lung edema, pulmonary hypertension, acute lung injury (ALI) and its most severe form acute respiratory distress syndrome (ARDS). A role of lipids in endothelial dysfunction is supported by the findings that accumulation of high levels of lipids in the circulation damage vascular tissues and their function.Citation1 Lipid-induced endothelial barrier dysfunction and its role in pathology of atherosclerosis and exacerbation of metabolic disorders such as obesity, diabetes has been described elsewhere and is not the scope of this review.Citation2–7 Rather, we will focus here on the endothelial barrier enhancing effects of bioactive phospholipids that have demonstrated protective effects in septic and aseptic models of ALI and inflammation in vitro as well as in vivo.
The endothelial barrier is a dynamic structure that constantly undergoes remodeling in response to mechanical forces or edemagenic and inflammatory agonists. The barrier disruptive and protective agents regulate endothelial permeability by altering the expression of cell-cell junction proteins or cell surface adhesion molecules, and reorganization of adherens junction (AJ), tight junction (TJ) and focal adhesion (FA) complexes.Citation8 The endothelial barrier function is regulated by a wide variety of signaling pathways, second messengers, kinases, phosphatases and small GTPases that determines cytoskeleton arrangements, cell contractility, and organization of cell junctions and focal adhesion (see [Citation9–12] for review). Among these, small GTPases, namely Rho and Rac, take the central stage in regulating endothelial barrier function which have opposing effects in controlling endothelial permeability. Many agonists including thrombin induce Rho activation that triggers actomyosin contraction and actin stress fiber formation leading to increased endothelial permeability.Citation10,13,14 In turn, barrier protective compounds including certain lipids activate Rac that decreases endothelial permeability and enhances barrier function by inducing the formation of cortical actin, stabilization of cell-cell junctions and inhibition of paracellular gaps formation.Citation13,15,16 A dynamic negative cross talk between Rho and Rac regulates overall endothelial permeability and inflammatory activation. For instance, Rho can inhibit the activation of Rac via Rho-associated kinase (ROCK) and Rac can also suppress Rho activity by activating p190RhoGAP.Citation17,18 In this line of Rho-Rac cross talk in determining EC barrier function, we have shown that activation of Rap1, which can also activate Rac signaling pathway of EC barrier enhancement, mediates agonist-induced EC barrier recovery by down-regulating Rho.Citation19,20
A direct link exists between the endothelial barrier dysfunction and inflammation.Citation21,22 Inflammatory agents increase the expression of endothelial cell-surface adhesion molecules- vascular cell adhesion molecule-1 (VCAM-1) and intercellular adhesion molecule-1 (ICAM-1) which promote increased neutrophil adhesion and transmigration, resulting in lung inflammation. The interactions between neutrophils and endothelium cause cytoskeletal rearrangement resulting in increased cellular gaps that leads to vascular permeability.Citation23–25 However, recent studies have shown that neutrophils extravasation does not necessarily always result in vascular permeability.Citation26,27 In addition to the potent endothelial barrier protective effects discussed below, anti-inflammatory properties of phospholipids underscore their potential importance as prototype molecules for development of future therapeutic interventions.
Barrier protective oxidized phospholipids
In response to tissue injury, various cell types release membrane vesicles containing oxidized phospholipids that can act as both pro- and anti-inflammatory mediators.Citation28–30 Enhanced production of oxidized phospholipids has been described in ARDS and asthma.Citation31,32 The lipid modification also occurs through inflammation-induced reactive nitrogen species.Citation33,34 For example, the non-enzymatic free radical-induced formation of isoprostanes has been detected in various lung diseases including ALI, ARDS, asthma, cystic fibrosis and pulmonary hypertension, and the measurement of isoprostanes levels has been proposed as an index of oxidant stress.Citation35–38 These lipid mediators modulate endothelial barrier function at different levels: by regulating the gene expression, by activating cell surface receptors and signaling pathways, and by modifying cytoskeleton remodeling and binding of immune cells to vascular endothelium.Citation7,39-45
Among various cellular phospholipids, 1-palmitoyl-2-arachidonoyl-sn-glycero-3-phosphorylcholine (PAPC) is one of the major plasma membrane phospholipids which undergoes oxidation to generate a group of compounds with various modified residues at the sn-2 position of the phospholipid. Initial studies suggested that oxidized PAPC (OxPAPC) triggers inflammatory cascades and is present in atherosclerotic lesions.Citation5,30,41,46,47 OxPAPC was also shown to stimulate endothelial cells to bind to monocytes and the levels of these oxidized phospholipids were elevated in atherosclerosis.Citation48 However, other simultaneous studies showed that OxPAPC plays a protective role against lipopolysaccharide (LPS)-induced inflammatory tissue damage.Citation49,50 The inhibitory effects of OxPAPC against inflammation were attributed to its ability to block the interaction of LPS with LPS-binding protein and CD14.Citation49 Likewise, OxPAPC also inhibited LPS or CpG DNA-induced tumor necrosis factor-α (TNF-α) production in mice by blocking the activation of p38 MAPK and the NF-κB pathway.Citation50 In consistence with these anti-inflammatory properties of OxPAPC, several reports demonstrated OxPAPC-induced enhancement of endothelial barrier function, protection from agonist-induced EC hyperpermeability and LPS-caused barrier disruption.Citation51,52 These mechanisms will be discussed in the next section.
OxPAPC, a potent endothelial barrier protective agent
Pioneer studies by our group have described potent and sustained barrier-enhancing responses in human pulmonary artery endothelial cells (HPAECs) to low OxPAPC concentrations (5–20 µg/ml) and attenuation of thrombin-induced EC barrier disruption.Citation51 These barrier protective effects of OxPAPC were mediated by the activation of small GTPases Cdc42 and Rac. Later, our studies also showed that OxPAPC protects against LPS-induced acute lung injury both in vitro and in vivo.Citation52 OxPAPC reduced LPS-induced inflammatory cytokines production, and, also attenuated LPS-caused endothelial barrier dysfunction by actin cytoskeletal and focal adhesion rearrangement.Citation52 OxPAPC also showed beneficial effects in ventilator-induced lung injury in rats and in mechanochemical-stimulated HPAECs.Citation53 Here, OxPAPC significantly reduced high tidal volume-induced protein and inflammatory cell accumulation in bronchoalveolar lavage (BAL) fluid and lung tissues in rats. In HPAECs, OxPAPC attenuated pathologic cyclic stretch (18%)-enhanced and thrombin-induced paracellular gaps by activating Rac.Citation53 With our continued interest in investigating the endothelial barrier protective activities of OxPAPC, we found that OxPAPC also protects against heat-killed Stapylococcus aureus-induced lung injury and endothelial permeability.Citation54 These cumulative findings strongly suggest that specific bioactive compounds generated during PAPC free radical oxidation show a promising therapeutic potential and may be used to mitigate acute lung injury caused by a wide range of agonists (). In addition to oxidation state, the polar head groups (phosphocholine, phosphoserine, phosphoethanolamine) contained in the PLs have been shown to be essential for barrier-protective effects of oxidized phospholipids. Interestingly, even among these structurally different species they showed different levels of protection against EC permeability caused by thrombin and other inflammatory agonists, while non-oxidized phospholipids did not exhibit any barrier protective effects.Citation55 To better establish the therapeutic potential of OxPAPC, we extensively studied the molecular and cellular mechanism(s) of its barrier protective and anti-inflammatory actions which will be described in the following section.
Figure 1. Mechanisms of OxPAPC-induced EC barrier protection. OxPAPC induces the activation of multiple signaling pathways that leads to the activation of Rap1 and Rac. The cytoskeletal remodeling facilitated by the cortical actin formation and assembly of tight junctions and adherens junctions proteins enhances lung endothelial barrier. In addition, EP4 receptor and lipoxin A4 also mediate the barrier protective effects of OxPAPC against LPS/TNFα-induced inflammation and lung injury in vitro and in vivo.
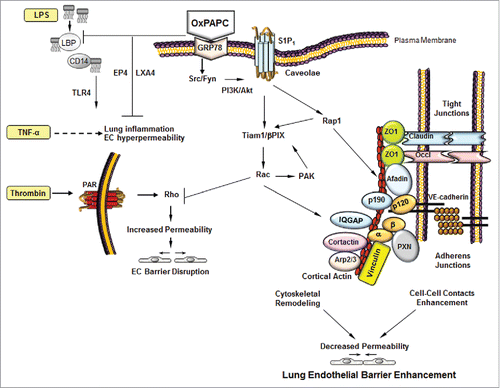
Intracellular signaling pathways involved in OxPAPC-induced barrier protection
OxPAPC activated protein kinase C (PKC), protein kinase A (PKA) and Raf-MEK1, 2-Erk 1, 2 mitogen-activated protein kinase (MAPK) cascade in HPAECs.Citation56 The same study also showed that OxPAPC induced tyrosine phosphorylation in EC and activated focal adhesion regulatory proteins cofillin, paxillin and focal adhesion kinase (FAK). The endothelial barrier enhancing and cytoskeletal remodeling effects of OxPAPC were mediated by the activation of Cdc42 and Rac.Citation51 Further studies revealed that Rac/Cdc42-specific guanine nucleotide exchange factors (GEFs) Tiam1 and betaPIX play a critical role in OxPAPC-induced Rac activation.Citation57 A cross talk between paxillin and Rac is essential for mediating barrier protective effects of OxPAPC which is achieved by p21-activated kinase (PAK1) dependent phosphorylation of paxillin that serves as a positive feedback loop and contributes to sustained barrier protection by OxPAPC.Citation58 On further characterizing the Rac-mediated endothelial barrier protective effects of OxPAPC, we found that inhibition of PKA, PKC, tyrosine phosphorylation of Src, FAK and paxillin as well as inhibition of small GTPase with toxin B abolished the OxPAPC-induced Rac activation and decreased paxillin and FAK phosphorylation.Citation59 The interaction between AJ and FA complexes mediated by the association between β-catenin and paxillin was also critical for OxPAPC-induced EC barrier regulation.Citation60 Similarly, Rap1-mediated association between AJ and TJ proteins plays an important role in OxPAPC-induced EC barrier enhancement.Citation61 Briefly, OxPAPC activates Rap1 and increases the peripheral accumulation of AJ proteins VE-cadherin, p120- and β-catenin as well as TJ proteins ZO-1 and occludin. Furthermore, the barrier protective effects of OxPAPC depend on Rap1-mediated assembly of AJ-TJ protein complexes by promoting the interaction of VE-cadherin with p120-catenin, β-catenin and ZO-1. Afadin, an AJ-associated Rap1 effector, also plays a crucial role in mediating OxPAPC-induced EC barrier protection. OxPAPC enhances the accumulation of afadin in cell periphery in a Rap1-depedent manner and also increases the association of afadin with p120-catenin and ZO-1 which is essential for counterbalancing thrombin-induced EC barrier disruption.Citation62 OxPAPC activates caveolin-enriched microdomains (CEMs) and rapidly recruits shingosine 1-phosphate receptor (S1P1), Akt kinase, Rac and Tiam1 to CEMs.Citation63 The depletion of Akt blocks OxPAPC-induced S1P1 activation and silencing of S1P1 inhibits Tiam recruitment to CEMs and Rac activation, ultimately abolishing OxPAPC-induced cortical cytoskeletal remodeling, assembly of cell junction protein complexes and barrier enhancement. These findings indicate that Akt-dependent transactivation of S1P1 in CEMs is essential for preserving the barrier protective effects of OxPAPC.Citation63 Further investigation revealed that OxPAPC-induced activation of S1P1 requires its binding to plasma membrane localized chaperone protein GRP78.Citation64 Briefly, binding of OxPAPC to GRP78 in the cell membrane induces GRP78 trafficking to CEMs which subsequently activates S1P1, Src and Fyn tyrosine kinases, and Rac1 GTPase that is essential for maintaining barrier protective actions of OxPAPC.Citation64
With our persistent focus to better understand the intracellular signaling pathways involved in mediating OxPAPC effects in EC, we explored molecular basis of OxPAPC-induced downregulation of RhoA signaling. It was found that p190RhoGAP, plays a critical role as a negative regulator of Rho GTPase, and its depletion abolishes the protective effects of OxPAPC against ventilator-induced lung injury.Citation65 The interaction of p190RhoGAP with intracellular protein p120-catenin was necessary for its membrane translocation and to mediate the barrier protective effects of OxPAPC by activating Rac1 and inhibiting Rho.Citation8 Furthermore, afadin-controlled interactions between AJ protein p120-catenin and TJ protein ZO-1 is also crucial for OxPAPC-induced endothelial barrier enhancement.Citation62 Vinculin, a protein involved in transmitting the mechanical forces signaling in EC, also plays a role in mediating barrier protective effects of OxPAPC.Citation66 OxPAPC-induced association of vinculin with VE-cadherin is essential for EC barrier enhancement whereas the association of vinculin with FA protein talin following thrombin treatment causes an increase in EC permeability. Likewise, IQ domain containing GTPase-activating protein (IQGAP1), an effector of Rac1/Cdc42, acts as a molecular transducer of OxPAPC-induced EC barrier enhancement signals by targeting the additional activation of Rac1/Cdc42, thereby providing a positive feedback loop of Rac1/Cdc42 signaling.Citation67 Our studies showed the essential role of IQGAP1 in mediating barrier protective effects of OxPAPC since its knockdown abolishes OxPAPC-induced barrier protection against thrombin due to the reduced Rac1 and Cdc42 activation as well as decreased membrane localization of cortactin, VE-cadherin and p120-catenin.Citation67 In conclusion, OxPAPC appears to activate a wide arrays of signaling cascades that cumulatively result in Rac-mediated cytoskeleton remodeling leading to EC barrier protection (). The signaling pathways involved in OxPAPC-mediated barrier protection in vivo are less known and one of our study has shown that Rap1 plays a critical role in mediating the protective effects of OxPAPC against mechanical ventilation-induced lung injury.Citation61 Since our in vitro studies have established that Rap1 activates Rac via Tiam1, it can be speculated that the same signaling axis may exist in vivo. Moreover, Rac-mediated activation of p190RhoGAP, a negative regulator or Rho, mediated the protective effects of OxPAPC against ventilator-induced lung injury in mice, indicating the direct involvement of Rac pathway. Future studies will determine the definite role of the Rac pathway in mediating OxPAPC-induced EC barrier enhancement in vivo.
Role of receptors in barrier protective effects of OxPAPC
OxPLs exert their biological effects by activating wide varieties of receptors including platelet activating factor (PAF) receptor, prostaglandin receptors E2 and D, scavenger receptor CD36, vascular endothelial growth factor receptor-2 (VEGFR-2), S1P1, toll-like receptor 4, and peroxisome proliferator-activated-receptors (PPARα and PPARγ).Citation63,68-73 In consistent with these reports, our most recent study revealed a novel role of a prostanoid receptor in mediating the sustained barrier enhancing effects of OxPAPC that was associated with the activation of Rac. By employing the specific pharmacological inhibitors and gene silencing with small interfering RNA, we showed that prostaglandin E receptor-4 (EP4) plays a critical role in maintaining the EC barrier protective effects during the sustained phase but has no effect on rapid phase of barrier enhancement by OxPAPC.Citation74 Consistently, EP4 receptor did not play any role in suppressing thrombin-induced EC barrier dysfunction due to its acute nature but was involved in mediating the protective effects of OxPAPC against TNF-α-induced sustained EC barrier dysfunction and inflammation. A definite role of EP4 receptor in OxPAPC-induced anti-inflammatory effects was evident in LPS-induced acute lung injury rodent model where the beneficial properties of OxPAPC observed in wild type mice were abolished in endothelial-specific EP4 knockout mice. These novel finding suggest that OxPAPC also exerts its protective effects via prostaglandin receptor, especially during the advance stage of barrier enhancement, which could be a promising therapeutic target against acute lung injury.
Role of lipoxins in anti-inflammatory effects of OxPAPC
The unique properties of OxPAPC that its single in vivo injection can produce long lasting anti-inflammatory and protective effects in lung prompted us to explore other additional mechanisms involved in this process. Recent study by our group revealed that OxPAPC treatment causes significant accumulation of a bioactive lipid mediator lipoxin A4 (LXA4) in HPAEC cultures as well as in the lungs of OxPAPC-treated mice.Citation75 This lipid mediator is of particular importance, since earlier studies have found that its analogues have anti-inflammatory effects on EC and inhibit VEGF-induced EC permeability and actin stress fiber formation.Citation76,77 We found that the anti-inflammatory effects of OxPAPC were mimicked by LXA4 against TNF-α induced EC permeability in vitro and LPS-induced ALI in vivo. In addition, the anti-inflammatory activities of OxPAPC were markedly suppressed in HPAECs following the pharmacological or genetic inhibition of LXA4 formyl-peptide receptor-2 (FPR2/ALX) and also in FPR2/ALX knock out mice.Citation75 These novel findings warrant a further investigation of such EC barrier and lung protective lipid molecules generated during the oxidation of phospholipids.
Barrier-disruptive phospholipid oxidation products
It is noteworthy to mention that some fragmented oxidation products of phospholipids cause EC barrier disruption () and even OxPAPC at higher concentrations induces hyperpermeability, actin stress fiber formation and myosin light chain phosphorylation in EC which is mediated by the activation of VEGFR-2.Citation78,79 In consistence with the role of VEGFR-2 in mediating higher dose of OxPAPC-induced barrier dysfunction, it was found to be a critical regulator during oxidized phospholipids-induced stimulation of EC via the activation of sterol regulatory element-binding protein (SREBP) and Erk 1/2.Citation68,80 Some studies also reported that oxidized phospholipids-activated VEGFR is responsible for activating transcription factor-4 mediated unfolded protein response in EC.Citation81,82 The dose-dependent differential effects i.e.; barrier protective at lower concentrations (5–20 μg/mL) and barrier disruptive at higher concentrations of OxPAPC (50–100 μg/mL) is due to the activation of different signaling cascades. The higher concentrations of OxPAPC increases the total cell protein tyrosine phosphorylation followed by the activation of Src kinase and phosphorylation of VE-cadherin limiting its ability to form complex with p120-catenin that results in increased EC permeability.Citation83 In addition, the fragmented products of PAPC oxidation such as lysophosphatidyl choline (lyso-PC), and 1-palmitoyl-2-(5-oxovaleroyl)-sn-glycero-phosphatidylchonine (POVPC) induce EC permeability via reactive oxygen species-dependent activation of Src and VE-cadherin phosphorylation but full length oxidation product 1-palmitoyl-2-(5, 6-epoxyisoprstane E2)-sn-glycero-3-phosphatidyl choline (PEIPC) induces EC barrier enhancement and also reverses barrier disruption caused by former fragmented lipid molecules.Citation79,83 The fragmented lipids induced the phosphorylation of VE-cadherin at both tyrosine residues 658 and 731 and phosphorylation of VE-cadherin is known to prevent its binding to p120- and β-catenin causing leukocytes transendothelial migration and EC barrier disruption.Citation84–86 However, a recent in vivo study has demonstrated that only phosphorylation at Tyr685 of VE-cadherin is involved in the induction of vascular permeability.Citation26 A role of oxidized phospholipid in EC barrier disruption was also revealed by a recent study where oxidized cardiolipin, a mitochondrial phospholipid, induced permeability as well as toxicity in cultured bovine pulmonary artery endothelial cells.Citation87 Based on these findings, it appears that wide varieties of structurally different oxidized phospholipids are generated with varying degree of cellular effects, either barrier protective or destructive functions largely determined by the type of signaling pathways activated.Citation88,89
Other lipids in endothelial barrier regulation
Sphingosine-1-phosphate
In addition to oxidized phospholipids, other lipid mediators are also known to enhance endothelial barrier function. Sphingosine-1-phosphate (S1P) represents a major group of biologically active sphingolipids which is produced from the sphingomyelin metabolic pathway.Citation90–92 (). Platelets are the major source of S1P and earlier studies showed marked endothelial barrier protective effects by platelets-released factors.Citation93,94 Later, it was confirmed that platelet-derived S1P is responsible for maintaining EC barrier integrity and also protects EC barrier dysfunction caused by thrombin.Citation95 S1P binds to five G-protein coupled receptor isoforms S1P1 to S1P5 to exert its effects on EC. The differential binding of S1P to its receptors has contrasting downstream physiological consequences.Citation96 For example, S1P at higher concentrations binds to S1P2 and S1P3 coupled to Gα12/13 or Gαq and disrupts EC barrier function through the activation of Rho.Citation16,97 On the other hand, S1P at lower concentrations binds to S1P1 coupled to Gαi and mediates EC barrier protective effects by the activation of Rac.Citation95,98 The varying effects of S1P on EC barrier function was also reported by another study which showed that at physiological concentrations S1P bound to S1P1 and preserved the EC barrier function by activating Rac where as excessive S1P bound to S1P2 and induced EC barrier dysfunction by activating RhoA.Citation99 A number of recent studies have confirmed that S1P1 is involved in strengthening EC barrier, inhibiting the release of inflammatory cytokines and suppressing the expression of EC surface adhesion molecules while S1P2 and S1P3 antagonize these beneficial effects of S1P1.Citation100–102 Animal models of ALI had also shown the protective effects of S1P on lung function.Citation103,104 Furthermore, the role of S1P1 in in vivo EC barrier protection was evident from a study where the barrier protective and anti-inflammatory effects of S1P1 receptor agonist was reduced in LPS-challenged S1P1 knock out mice.Citation105 Interestingly, as discussed above, OxPAPC also activates S1P1 to induce EC barrier enhancement. With these promising protective effects and its reduced levels in sepsis patients, S1P might be considered as a potential therapeutic target as well as biomarker for EC barrier dysfunction and sepsis.Citation106
The studies have established that activation of Rac and subsequent cytoskeletal remodeling is the major intracellular signaling pathway that mediates the beneficial effects of S1P on pulmonary EC.Citation95,107,108 The pathways activated by S1P upstream of Rac include PI3K, Src, and elevation of intracellular calcium concentration.Citation107,109 A synthetic analogue of sphingosine, FTY720 which acts as a potent agonist for S1P1 and S1P3 after getting phosphorylated inside the cells, has shown promising EC barrier protective effects in vitro and in vivo.Citation110,111 With its therapeutic potential in reducing ALI in rats with necrotizing pancreatitis and also prolonging the survival of rodents by increasing cytokines production in ischemia-reperfusion model, FTY720 has undergone Phase III clinical trial as an immunosuppressant for the prevention of solid organ transplant rejection.Citation103,112-114 Several novel derivatives of FTY720 have been developed in the recent years which exhibit preventive effects against EC barrier dysfunction, lung injury and inflammation.Citation111
Prostaglandins and other cyclooxygenase metabolites
Prostaglandins (PGs) represent another important group of lipid mediators with potent barrier protective effects in the pulmonary endothelium.Citation115,116 PGs are the products of cyclooxygenase (COX)-mediated arachidonic acid metabolic pathway and synthesized by many tissues including vascular endothelial cells (). The role of PGs in the regulation of vascular permeability is complex since their cellular effects is largely determined by the receptor types. For instance, PGs may be involved in causing acute lung injury via vasodilatory effects but also exhibit protective effects in the resolution of inflammation as observed with PGI2 and enhancement of EC barrier function by PGE2, PGI2 and PGD2.Citation20,117,118 Furthermore, stable PGI2 analogs beraprost and iloprost which are widely used for the treatment of pulmonary hypertension, exhibit anti-inflammatory and anti-edemagenic effects in various models of acute lung injury.Citation119,120 A number of studies from our group has shown the barrier protective effects of beraprost and iloprost against various models of ALI including LPS and mechanical ventilation.Citation121–124 Our studies also established a critical role of cAMP-activated PKA and Epac-Rap1 pathway in mediating the barrier protective effects of PGs and iloprost. Rac is activated by both PKA and Epac-Rap1, thus both of these signaling axis may converge into a universal pathway of barrier protection via Rac activation which is mediated by Rac-specific GEFs Tiam1 and Vav2.Citation20,125 In one of our most recent study, we performed a comprehensive analysis of barrier protective and anti-inflammatory activities of various PGs on pulmonary EC in vitro and in vivo and identify PGE2, PGI2 and PGA2 with most potent beneficial effects.Citation126 Furthermore, our studies have also identified a critical role of PGs receptor EP4 in PGA2 and OxPAPC-induced prevention of EC permeability and lung inflammation.Citation74,127
In addition to PGs, arachidonic acid metabolism by COX also generates other products such as thromboxane A2 (TXA2), leukotrienes, and platelet-activating factor (PAF) which mostly act as EC barrier disruptive agents.Citation128 Among these COX metabolites, TXA2 is produced by several cell types including platelets, and EC and it causes platelet aggregation and vasoconstriction.Citation129,130 TXA2 is known to increase EC permeability,Citation131,132 and more importantly, it is present in the lungs of ALI/ARDS patients where it plays an inflammatory role.Citation133–135 In consistent with the role of TXA2 in lung injury, a recent study reported that TXA2 disrupts EC barrier by the activation of Rho and promotes lung edema formation.Citation136 Likewise, another study showed that hyperglycemia-activated TXA2 disrupts the integrity and compromises the function of blood- brain barrier in brain microvascular endothelial cells.Citation137 Besides TXA2, the activation of PAF is also known to impair endothelial barrier function.Citation138,139
The promising EC barrier protective effects of PGs and oxidized phospholipids in vitro and in vivo do not easily translate into therapeutics since PGs have very short half-life in vivo (∼ 40 seconds) and phospholipids are prone to cleavage by intracellular and extracellular phospholipases. To overcome these limitations, we recently developed a novel approach to synthesize a new class of phospholipase cleavage resistant PG-phospholipid compound by incorporating iloprost into phosphatidylcholine (Ilo-PC).Citation140 Ilo-PC showed more potent and long lasting EC barrier protective and anti-inflammatory effects over free iloprost. These superior effects of Ilo-PC were associated with its ability to induce prolonged activation of Rap1 and Rac GTPases.Citation140 The enhanced protective effects of Ilo-PC might have been contributed by various factors including its increased stability, ability to better interact with cell lipid layer to engage the receptors more efficiently and involvement of additional signaling pathways to activate Rap1/Rac. Currently, we are investigating all these possible scenarios and, nevertheless the far superior EC barrier protective and anti-inflammatory effects of Ilo-PC suggests that such stable PGs-phospholipid compounds could be potential therapeutics for restoring EC barrier integrity to treat lung injuries.
Future perspectives of therapeutic potential of oxidized phospholipids
The role of oxidized phospholipids in the regulation of EC barrier function has long been appreciated but the later studies have highlighted their barrier protective and anti-inflammatory properties. Among these, our extensive studies provide a substantial evidence that OxPAPC products could be promising prototype molecules for therapeutic treatment of lung injury, acute vascular endothelial inflammation and barrier dysfunction (). Mechanistically, OxPAPC employs multiple barrier protective pathways that makes it a suitable target against wide varieties of insults and injury in the pulmonary endothelium. It also causes a sustained EC barrier enhancement, indicating the very stable and prolonged effects, thus making it therapeutically viable. Further, the ability to produce long lasting protective effects after a single in vivo injection makes it an ideal candidate for ALI therapies. The involvement of prostaglandin receptor EP4 in OxPAPC-induced EC barrier function enhancement further strengthens its therapeutic potential since we recently demonstrated that EP4- mediated prostaglandin A2 (PGA2) barrier protective and anti-inflammatory effects were very effective in suppressing thrombin and LPS-induced acute lung injury in vitro and in vivo.Citation127 Moreover, our latest findings that OxPAPC treatment generates other important bioactive lipid mediators such as LXA4 with equally effective in anti-inflammatory and barrier protective functions underscores the potential of this compound in therapeutic development against lung injury and inflammation. To further investigate the therapeutic potential of oxidized phospholipids, future studies need to target on identifying more such bioactive, endothelial barrier protective and anti-inflammatory species of phospholipids oxidation products by applying advanced mass spectroscopic analysis and developing antibodies to detect them. In addition, testing the protective efficacy of oxidized phospholipids in vivo against various pulmonary and cardiovascular diseases associated with endothelial barrier dysfunction and inflammation will determine the translation of these molecules into clinics. Given that protective effects of phospholipids is mediated by a cumulative and complex cellular events of activation of receptors, intracellular signaling pathways as well as remodeling of cytoskeletal organization, future studies are required to identify the precise molecular and cellular mechanisms of phospholipids-induced endothelial barrier protection in vivo. Furthermore, new strategies to get rid of EC barrier disruptive truncated phospholipids should be considered. For this purpose, platelet activating factor acetylhydrolases (PAF-AHs) which specifically target the hydrolysis of truncated phospholipids could be employed. These oxidized phospholipid-specific phospholipases are known to reduce inflammation and apoptosis by selectively removing oxidatively truncated phospholipids.Citation141,142 Finally, besides EC barrier dysfunction, emerging evidence suggests that oxidized phospholipids play an important role in pathophysiology of other diseases, thus the knowledge on their structure, function, mechanisms of actions could potentially lead to the development of effective therapeutics against these diseases.Citation143–145 With these diverse effects in multiple diseases, the identification and quantification of various oxidized phospholipids are proposed to be utilized as biomarkers.Citation146
Figure 5. OxPAPC enhances endothelial barrier function and attenuates agonist-induced endothelial barrier dysfunction in vitro and in vivo. (A) HPAECs grown on coverslips were treated with OxPAPC (15 µg/mL, 30 minutes) and dual immunofluorescence staining with Texas-Red phalloidin and VE-cadherin was performed to monitor actin cytoskeleton and cell junction remodeling. The confocal images illustrated the barrier enhancing effects of OxPAPC as evidenced by increased F-actin and VE-Cadherin staining. (B) HPAECs grown on biotinylated gelatin substrate were pre-treated with OxPAPC (15 µg/mL, 30 minutes) followed by thrombin stimulation (0.5 Units/mL, 10 minutes) and cell permeability was visualized using FITC-avidin as a tracer. OxPAPC treatment protected thrombin-induced permeability as demonstrated by reduced green fluorescence that depicts areas permeable for FITC-avidin. (C) Intravenous injection of (1.5 mg/kg) of OxPAPC after 5 hours of LPS instillation (0.7 mg/kg, intratracheal) in mice protects against LPS-induced lung injury as detected by Evans blue staining of lung.
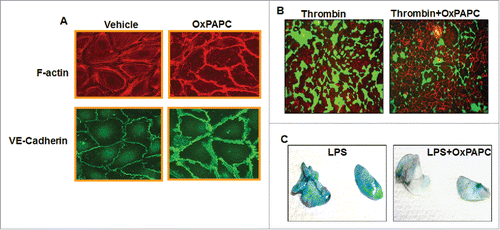
Conclusion
Bioactive lipids are critical regulators of endothelial barrier function. Products of polyunsaturated fatty acid (PUFA) enzymatic conversion (Prostaglandins, leukotriens, thromboxanes, lipoxin, resolvins, etc.) and the phospholipids generated during oxidative or nitrosative stress exhibit both pro- and anti-inflammatory effects on EC. Among such oxidized phospholipids species, full length products of phosphocholine and phosphoserine oxidation represent a class of OxPL products with potent EC barrier protective and anti-inflammatory activities in vitro and in vivo. It appears that cells produce these bioactive lipid molecules in response to injury or inflammation as a recovery or survival mechanism. Since some OxPL products, especially the fragmented oxidation products and also the higher concentrations of full length products, have barrier disruptive and inflammatory effects it is critical to precisely identify the correct species and concentrations to introduce these molecules into clinics. Consideration of employing specific phospholipases such as PAF-AHs which selectively remove truncated oxidized phospholipids might be important for mitigating the deleterious effects caused by these fragmented lipid products. The extensive studies by our and other groups have characterized intracellular signaling pathways and described receptors mediating barrier enhancing functions of OxPAPC. The engagement of multiple EC barrier enhancing and lung injury recovery pathways suggests OxPAPC products as a potential therapeutics of choice, as they show a great potential in suppressing the EC barrier dysfunction and lung injury caused by wide variety of agonists. Moreover, the identification of novel pathways triggered by other lipid mediators and contributing to their anti-inflammatory and barrier-protective effects could lead to the development of effective therapeutics against EC barrier dysfunction.
Abbreviations
ALI | = | acute lung injury |
ARDS | = | acute respiratory distress syndrome |
EP4 | = | prostaglandin E receptor-4 |
HPAECs | = | human pulmonary artery endothelial cells |
LPS | = | lipopolysaccharide |
LXA4 | = | lipoxin A4 |
OxPL | = | oxidized phospholipids |
OxPAPC | = | oxidized 1-palmitoyl-2-arachidonoyl-sn-glycero-3-phosphorylcholine |
PGs | = | prostaglandins |
S1P | = | shingosine 1-phosphate |
TNF-α | = | tumor necrosis factor-α |
Disclosure of potential conflicts of interest
No potential conflicts of interest were disclosed.
Funding
This work was supported by the grants: HL076259, HL087823 from the National Heart, Lung, and Blood Institute, and GM122940 from the National Institute of General Medical Sciences.
References
- Kim JA, Montagnani M, Chandrasekran S, Quon MJ. Role of lipotoxicity in endothelial dysfunction. Heart Fail Clin. 2012;8:589–607. doi:10.1016/j.hfc.2012.06.012. PMID:22999242.
- Berg AH, Scherer PE. Adipose tissue, inflammation, and cardiovascular disease. Circ Res. 2005;96:939–49. doi:10.1161/01.RES.0000163635.62927.34. PMID:15890981.
- Steinberg HO, Chaker H, Leaming R, Johnson A, Brechtel G, Baron AD. Obesity/insulin resistance is associated with endothelial dysfunction. Implications for the syndrome of insulin resistance. J Clin Invest. 1996;97:2601–10. doi:10.1172/JCI118709. PMID:8647954.
- Berliner JA, Gharavi NM. Endothelial cell regulation by phospholipid oxidation products. Free Radic Biol Med. 2008;45:119–23. doi:10.1016/j.freeradbiomed.2008.04.013. PMID:18460347.
- Lee S, Birukov KG, Romanoski CE, Springstead JR, Lusis AJ, Berliner JA. Role of phospholipid oxidation products in atherosclerosis. Circ Res. 2012;111:778–99. doi:10.1161/CIRCRESAHA.111.256859. PMID:22935534.
- Bochkov VN, Oskolkova OV, Birukov KG, Levonen AL, Binder CJ, Stockl J. Generation and biological activities of oxidized phospholipids. Antioxid Redox Signaling. 2010;12:1009–59. doi:10.1089/ars.2009.2597.
- Bochkov V, Gesslbauer B, Mauerhofer C, Philippova M, Erne P, Oskolkova OV. Pleiotropic effects of oxidized phospholipids. Free Radic Biol Med. 2016;111:6–24. doi:10.1016/j.freeradbiomed.2016.12.034. PMID:28027924.
- Zebda N, Tian Y, Tian X, Gawlak G, Higginbotham K, Reynolds AB, Birukova AA, Birukov KG. Interaction of p190RhoGAP with C-terminal domain of p120-catenin modulates endothelial cytoskeleton and permeability. J Biol Chem. 2013;288:18290–9. doi:10.1074/jbc.M112.432757. PMID:23653363.
- Garcia-Ponce A, Citalan-Madrid AF, Velazquez-Avila M, Vargas-Robles H, Schnoor M. The role of actin-binding proteins in the control of endothelial barrier integrity. Thromb Haemost. 2015;113:20–36. doi:10.1160/TH14-04-0298. PMID:25183310.
- Spindler V, Schlegel N, Waschke J. Role of GTPases in control of microvascular permeability. Cardiovasc Res. 2010;87:243–53. doi:10.1093/cvr/cvq086. PMID:20299335.
- Trani M, Dejana E. New insights in the control of vascular permeability: vascular endothelial-cadherin and other players. Curr Opin Hematol. 2015;22:267–72. doi:10.1097/MOH.0000000000000137. PMID:25767951.
- Oldenburg J, de Rooij J. Mechanical control of the endothelial barrier. Cell Tissue Res. 2014;355:545–55. doi:10.1007/s00441-013-1792-6. PMID:24519624.
- Vandenbroucke E, Mehta D, Minshall R, Malik AB. Regulation of endothelial junctional permeability. Ann N Y Acad Sci. 2008;1123:134–45. doi:10.1196/annals.1420.016. PMID:18375586.
- van Nieuw Amerongen GP, van Delft S, Vermeer MA, Collard JG, van Hinsbergh VW. Activation of RhoA by thrombin in endothelial hyperpermeability: role of Rho kinase and protein tyrosine kinases. Circ Res. 2000;87:335–40. doi:10.1161/01.RES.87.4.335. PMID:10948069.
- Kumar P, Shen Q, Pivetti CD, Lee ES, Wu MH, Yuan SY. Molecular mechanisms of endothelial hyperpermeability: implications in inflammation. Expert Rev Mol Med. 2009;11:e19. doi:10.1017/S1462399409001112. PMID:19563700.
- Wang L, Dudek SM. Regulation of vascular permeability by sphingosine 1-phosphate. Microvasc Res. 2009;77:39–45. doi:10.1016/j.mvr.2008.09.005. PMID:18973762.
- Worthylake RA, Burridge K. RhoA and ROCK promote migration by limiting membrane protrusions. J Biol Chem. 2003;278:13578–84. doi:10.1074/jbc.M211584200. PMID:12574166.
- Bustos RI, Forget MA, Settleman JE, Hansen SH. Coordination of Rho and Rac GTPase function via p190B RhoGAP. Curr Biol. 2008;18:1606–11. doi:10.1016/j.cub.2008.09.019. PMID:18948007.
- Birukova AA, Tian X, Tian Y, Higginbotham K, Birukov KG. Rap-afadin axis in control of Rho signaling and endothelial barrier recovery. Mol Biol Cell. 2013;24:2678–88. doi:10.1091/mbc.E13-02-0098. PMID:23864716.
- Birukova AA, Zagranichnaya T, Fu P, Alekseeva E, Chen W, Jacobson JR, Birukov KG. Prostaglandins PGE(2) and PGI(2) promote endothelial barrier enhancement via PKA- and Epac1/Rap1-dependent Rac activation. Exp Cell Res. 2007;313:2504–20. doi:10.1016/j.yexcr.2007.03.036. PMID:17493609.
- Zhang C. The role of inflammatory cytokines in endothelial dysfunction. Basic Res Cardiol. 2008;103:398–406. doi:10.1007/s00395-008-0733-0. PMID:18600364.
- Pober JS, Sessa WC. Evolving functions of endothelial cells in inflammation. Nat Rev Immunol. 2007;7:803–15. doi:10.1038/nri2171. PMID:17893694.
- DiStasi MR, Ley K. Opening the flood-gates: how neutrophil-endothelial interactions regulate permeability. Trends Immunol. 2009;30:547–56. doi:10.1016/j.it.2009.07.012. PMID:19783480.
- Clark PR, Manes TD, Pober JS, Kluger MS. Increased ICAM-1 expression causes endothelial cell leakiness, cytoskeletal reorganization and junctional alterations. J Invest Dermatol. 2007;127:762–74. doi:10.1038/sj.jid.5700670. PMID:17195014.
- Cook-Mills JM, Marchese ME, Abdala-Valencia H. Vascular cell adhesion molecule-1 expression and signaling during disease: regulation by reactive oxygen species and antioxidants. Antioxid Redox Signaling. 2011;15:1607–38. doi:10.1089/ars.2010.3522.
- Wessel F, Winderlich M, Holm M, Frye M, Rivera-Galdos R, Vockel M, Linnepe R, Ipe U, Stadtmann A, Zarbock A, et al. Leukocyte extravasation and vascular permeability are each controlled in vivo by different tyrosine residues of VE-cadherin. Nat Immunol. 2014;15:223–30. doi:10.1038/ni.2824. PMID:24487320.
- Heemskerk N, Schimmel L, Oort C, van Rijssel J, Yin T, Ma B, van Unen J, Pitter B, Huveneers S, Goedhart J, et al. F-actin-rich contractile endothelial pores prevent vascular leakage during leukocyte diapedesis through local RhoA signalling. Nat Commun. 2016;7:10493. doi:10.1038/ncomms10493. PMID:26814335.
- Bochkov VN, Leitinger N. Anti-inflammatory properties of lipid oxidation products. J Mol Med (Berl). 2003;81:613–26. doi:10.1007/s00109-003-0467-2. PMID:13679995.
- Huber J, Vales A, Mitulovic G, Blumer M, Schmid R, Witztum JL, Binder BR, Leitinger N. Oxidized membrane vesicles and blebs from apoptotic cells contain biologically active oxidized phospholipids that induce monocyte-endothelial interactions. Arterioscler Thromb Vasc Biol. 2002;22:101–7. doi:10.1161/hq0102.101525. PMID:11788468.
- Leitinger N. Oxidized phospholipids as modulators of inflammation in atherosclerosis. Curr Opin Lipidol. 2003;14:421–30. doi:10.1097/00041433-200310000-00002. PMID:14501580.
- Chabot F, Mitchell JA, Gutteridge JM, Evans TW. Reactive oxygen species in acute lung injury. Eur Respir J. 1998;11:745–57. PMID:9596132.
- Wood LG, Gibson PG, Garg ML. Biomarkers of lipid peroxidation, airway inflammation and asthma. Eur Respir J. 2003;21:177–86. doi:10.1183/09031936.03.00017003a. PMID:12570126.
- Kalyanaraman B. Nitrated lipids: a class of cell-signaling molecules. Proc Natl Acad Sci U S A. 2004;101:11527–8. doi:10.1073/pnas.0404309101. PMID:15292510.
- Pennathur S, Bergt C, Shao B, Byun J, Kassim SY, Singh P, Green PS, McDonald TO, Brunzell J, Chait A, et al. Human atherosclerotic intima and blood of patients with established coronary artery disease contain high density lipoprotein damaged by reactive nitrogen species. J Biol Chem. 2004;279:42977–83. doi:10.1074/jbc.M406762200. PMID:15292228.
- Carpenter CT, Price PV, Christman BW. Exhaled breath condensate isoprostanes are elevated in patients with acute lung injury or ARDS. Chest. 1998;114:1653–9. doi:10.1378/chest.114.6.1653. PMID:9872202.
- Cracowski JL, Cracowski C, Bessard G, Pepin JL, Bessard J, Schwebel C, Stanke-Labesque F, Pison C. Increased lipid peroxidation in patients with pulmonary hypertension. Am J Respir Crit Care Med. 2001;164:1038–42. doi:10.1164/ajrccm.164.6.2104033. PMID:11587993.
- Montuschi P, Corradi M, Ciabattoni G, Nightingale J, Kharitonov SA, Barnes PJ. Increased 8-isoprostane, a marker of oxidative stress, in exhaled condensate of asthma patients. Am J Respir Crit Care Med. 1999;160:216–20. doi:10.1164/ajrccm.160.1.9809140. PMID:10390403.
- Montuschi P, Kharitonov SA, Ciabattoni G, Corradi M, van Rensen L, Geddes DM, Hodson ME, Barnes PJ. Exhaled 8-isoprostane as a new non-invasive biomarker of oxidative stress in cystic fibrosis. Thorax. 2000;55:205–9. doi:10.1136/thorax.55.3.205. PMID:10679539.
- Greig FH, Kennedy S, Spickett CM. Physiological effects of oxidized phospholipids and their cellular signaling mechanisms in inflammation. Free Radic Biol Med. 2012;52:266–80. doi:10.1016/j.freeradbiomed.2011.10.481. PMID:22080084.
- Gugiu BG, Mouillesseaux K, Duong V, Herzog T, Hekimian A, Koroniak L, Vondriska TM, Watson AD. Protein targets of oxidized phospholipids in endothelial cells. J Lipid Res. 2008;49:510–20. doi:10.1194/jlr.M700264-JLR200. PMID:18071189.
- Cole AL, Subbanagounder G, Mukhopadhyay S, Berliner JA, Vora DK. Oxidized phospholipid-induced endothelial cell/monocyte interaction is mediated by a cAMP-dependent R-Ras/PI3-kinase pathway. Arterioscler Thromb Vasc Biol. 2003;23:1384–90. doi:10.1161/01.ATV.0000081215.45714.71. PMID:12805072.
- Patricia MK, Kim JA, Harper CM, Shih PT, Berliner JA, Natarajan R, Nadler JL, Hedrick CC. Lipoxygenase products increase monocyte adhesion to human aortic endothelial cells. Arterioscler Thromb Vasc Biol. 1999;19:2615–22. doi:10.1161/01.ATV.19.11.2615. PMID:10559003.
- Shih PT, Elices MJ, Fang ZT, Ugarova TP, Strahl D, Territo MC, Frank JS, Kovach NL, Cabanas C, Berliner JA, et al. Minimally modified low-density lipoprotein induces monocyte adhesion to endothelial connecting segment-1 by activating beta1 integrin. J Clin Invest. 1999;103:613–25. doi:10.1172/JCI5710. PMID:10074478.
- Gargalovic PS, Imura M, Zhang B, Gharavi NM, Clark MJ, Pagnon J, Yang WP, He A, Truong A, Patel S, et al. Identification of inflammatory gene modules based on variations of human endothelial cell responses to oxidized lipids. Proc Natl Acad Sci U S A. 2006;103:12741–6. doi:10.1073/pnas.0605457103. PMID:16912112.
- Yeh M, Gharavi NM, Choi J, Hsieh X, Reed E, Mouillesseaux KP, Cole AL, Reddy ST, Berliner JA. Oxidized phospholipids increase interleukin 8 (IL-8) synthesis by activation of the c-src/signal transducers and activators of transcription (STAT)3 pathway. J Biol Chem. 2004;279:30175–81. doi:10.1074/jbc.M312198200. PMID:15143062.
- Bochkov VN, Mechtcheriakova D, Lucerna M, Huber J, Malli R, Graier WF, Hofer E, Binder BR, Leitinger N. Oxidized phospholipids stimulate tissue factor expression in human endothelial cells via activation of ERK/EGR-1 and Ca(++)/NFAT. Blood. 2002;99:199–206. doi:10.1182/blood.V99.1.199. PMID:11756172.
- Lusis AJ. Atherosclerosis. Nature. 2000;407:233–41. doi:10.1038/35025203. PMID:11001066.
- Watson AD, Leitinger N, Navab M, Faull KF, Horkko S, Witztum JL, Palinski W, Schwenke D, Salomon RG, Sha W, et al. Structural identification by mass spectrometry of oxidized phospholipids in minimally oxidized low density lipoprotein that induce monocyte/endothelial interactions and evidence for their presence in vivo. J Biol Chem. 1997;272:13597–607. doi:10.1074/jbc.272.21.13597. PMID:9153208.
- Bochkov VN, Kadl A, Huber J, Gruber F, Binder BR, Leitinger N. Protective role of phospholipid oxidation products in endotoxin-induced tissue damage. Nature. 2002;419:77–81. doi:10.1038/nature01023. PMID:12214235.
- Ma Z, Li J, Yang L, Mu Y, Xie W, Pitt B, Li S. Inhibition of LPS- and CpG DNA-induced TNF-alpha response by oxidized phospholipids. Am J Physiol Lung Cell Mol Physiol. 2004;286:L808–16. doi:10.1152/ajplung.00220.2003. PMID:14644758.
- Birukov KG, Bochkov VN, Birukova AA, Kawkitinarong K, Rios A, Leitner A, Verin AD, Bokoch GM, Leitinger N, Garcia JG. Epoxycyclopentenone-containing oxidized phospholipids restore endothelial barrier function via Cdc42 and Rac. Circ Res. 2004;95:892–901. doi:10.1161/01.RES.0000147310.18962.06. PMID:15472119.
- Nonas S, Miller I, Kawkitinarong K, Chatchavalvanich S, Gorshkova I, Bochkov VN, Leitinger N, Natarajan V, Garcia JG, Birukov KG. Oxidized phospholipids reduce vascular leak and inflammation in rat model of acute lung injury. Am J Respir Crit Care Med. 2006;173:1130–8. doi:10.1164/rccm.200511-1737OC. PMID:16514111.
- Nonas S, Birukova AA, Fu P, Xing J, Chatchavalvanich S, Bochkov VN, Leitinger N, Garcia JG, Birukov KG. Oxidized phospholipids reduce ventilator-induced vascular leak and inflammation in vivo. Crit Care. 2008;12:R27. doi:10.1186/cc6805. PMID:18304335.
- Meliton AY, Meng F, Tian Y, Sarich N, Mutlu GM, Birukova AA, Birukov KG. Oxidized phospholipids protect against lung injury and endothelial barrier dysfunction caused by heat-inactivated Staphylococcus aureus. Am J Physiol Lung Cell Mol Physiol. 2015;308:L550–62. doi:10.1152/ajplung.00248.2014. PMID:25575515.
- Birukova AA, Fu P, Chatchavalvanich S, Burdette D, Oskolkova O, Bochkov VN, Birukov KG. Polar head groups are important for barrier-protective effects of oxidized phospholipids on pulmonary endothelium. Am J Physiol Lung Cell Mol Physiol. 2007;292:L924–35. doi:10.1152/ajplung.00395.2006. PMID:17158600.
- Birukov KG, Leitinger N, Bochkov VN, Garcia JG. Signal transduction pathways activated in human pulmonary endothelial cells by OxPAPC, a bioactive component of oxidized lipoproteins. Microvasc Res. 2004;67:18–28. doi:10.1016/j.mvr.2003.09.004. PMID:14709399.
- Birukova AA, Malyukova I, Mikaelyan A, Fu P, Birukov KG. Tiam1 and betaPIX mediate Rac-dependent endothelial barrier protective response to oxidized phospholipids. J Cell Physiol. 2007;211:608–17. doi:10.1002/jcp.20966. PMID:17219408.
- Birukova AA, Alekseeva E, Cokic I, Turner CE, Birukov KG. Cross talk between paxillin and Rac is critical for mediation of barrier-protective effects by oxidized phospholipids. Am J Physiol Lung Cell Mol Physiol. 2008;295:L593–602. doi:10.1152/ajplung.90257.2008. PMID:18676874.
- Birukova AA, Chatchavalvanich S, Oskolkova O, Bochkov VN, Birukov KG. Signaling pathways involved in OxPAPC-induced pulmonary endothelial barrier protection. Microvasc Res. 2007;73:173–81. doi:10.1016/j.mvr.2006.12.004. PMID:17292425.
- Birukova AA, Malyukova I, Poroyko V, Birukov KG. Paxillin-beta-catenin interactions are involved in Rac/Cdc42-mediated endothelial barrier-protective response to oxidized phospholipids. Am J Physiol Lung Cell Mol Physiol. 2007;293:L199–211. doi:10.1152/ajplung.00020.2007. PMID:17513457.
- Birukova AA, Zebda N, Fu P, Poroyko V, Cokic I, Birukov KG. Association between adherens junctions and tight junctions via Rap1 promotes barrier protective effects of oxidized phospholipids. J Cell Physiol. 2011;226:2052–62. doi:10.1002/jcp.22543. PMID:21520057.
- Birukova AA, Fu P, Wu T, Dubrovskyi O, Sarich N, Poroyko V, Birukov KG. Afadin controls p120-catenin-ZO-1 interactions leading to endothelial barrier enhancement by oxidized phospholipids. J Cell Physiol. 2012;227:1883–90. doi:10.1002/jcp.22916. PMID:21732359.
- Singleton PA, Chatchavalvanich S, Fu P, Xing J, Birukova AA, Fortune JA, Klibanov AM, Garcia JG, Birukov KG. Akt-mediated transactivation of the S1P1 receptor in caveolin-enriched microdomains regulates endothelial barrier enhancement by oxidized phospholipids. Circ Res. 2009;104:978–86. doi:10.1161/CIRCRESAHA.108.193367. PMID:19286607.
- Birukova AA, Singleton PA, Gawlak G, Tian X, Mirzapoiazova T, Mambetsariev B, Dubrovskyi O, Oskolkova OV, Bochkov VN, Birukov KG. GRP78 is a novel receptor initiating a vascular barrier protective response to oxidized phospholipids. Mol Biol Cell. 2014;25:2006–16. doi:10.1091/mbc.E13-12-0743. PMID:24829380.
- Birukova AA, Zebda N, Cokic I, Fu P, Wu T, Dubrovskyi O, Birukov KG. p190RhoGAP mediates protective effects of oxidized phospholipids in the models of ventilator-induced lung injury. Exp Cell Res. 2011;317:859–72. doi:10.1016/j.yexcr.2010.11.011. PMID:21111731.
- Birukova AA, Shah AS, Tian Y, Moldobaeva N, Birukov KG. Dual role of vinculin in barrier-disruptive and barrier-enhancing endothelial cell responses. Cell Signal. 2016;28:541–51. doi:10.1016/j.cellsig.2016.02.015. PMID:26923917.
- Tian Y, Tian X, Gawlak G, Sarich N, Sacks DB, Birukova AA, Birukov KG. Role of IQGAP1 in endothelial barrier enhancement caused by OxPAPC. Am J Physiol Lung Cell Mol Physiol. 2016;311:L800–L809. doi:10.1152/ajplung.00095.2016. PMID:27566003.
- Zimman A, Mouillesseaux KP, Le T, Gharavi NM, Ryvkin A, Graeber TG, Chen TT, Watson AD, Berliner JA. Vascular endothelial growth factor receptor 2 plays a role in the activation of aortic endothelial cells by oxidized phospholipids. Arterioscler Thromb Vasc Biol. 2007;27:332–8. doi:10.1161/01.ATV.0000252842.57585.df. PMID:17110601.
- Pegorier S, Stengel D, Durand H, Croset M, Ninio E. Oxidized phospholipid: POVPC binds to platelet-activating-factor receptor on human macrophages. Implications in atherosclerosis. Atherosclerosis. 2006;188:433–43. doi:10.1016/j.atherosclerosis.2005.11.015. PMID:16386258.
- Li R, Mouillesseaux KP, Montoya D, Cruz D, Gharavi N, Dun M, Koroniak L, Berliner JA. Identification of prostaglandin E2 receptor subtype 2 as a receptor activated by OxPAPC. Circ Res. 2006;98:642–50. doi:10.1161/01.RES.0000207394.39249.fc. PMID:16456101.
- Podrez EA, Poliakov E, Shen Z, Zhang R, Deng Y, Sun M, Finton PJ, Shan L, Gugiu B, Fox PL, et al. Identification of a novel family of oxidized phospholipids that serve as ligands for the macrophage scavenger receptor CD36. J Biol Chem. 2002;277:38503–16. doi:10.1074/jbc.M203318200 10.1074/jbc.M205924200. PMID:12105195.
- Walton KA, Hsieh X, Gharavi N, Wang S, Wang G, Yeh M, Cole AL, Berliner JA. Receptors involved in the oxidized 1-palmitoyl-2-arachidonoyl-sn-glycero-3-phosphorylcholine-mediated synthesis of interleukin-8. A role for Toll-like receptor 4 and a glycosylphosphatidylinositol-anchored protein. J Biol Chem. 2003;278:29661–6. doi:10.1074/jbc.M300738200. PMID:12777373.
- Lee H, Shi W, Tontonoz P, Wang S, Subbanagounder G, Hedrick CC, Hama S, Borromeo C, Evans RM, Berliner JA, et al. Role for peroxisome proliferator-activated receptor alpha in oxidized phospholipid-induced synthesis of monocyte chemotactic protein-1 and interleukin-8 by endothelial cells. Circ Res. 2000;87:516–21. doi:10.1161/01.RES.87.6.516. PMID:10988245.
- Oskolkova O, Gawlak G, Tian Y, Ke Y, Sarich N, Son S, Andreasson K, Bochkov VN, Birukova AA, Birukov KG. Prostaglandin E receptor-4 receptor mediates endothelial barrier-enhancing and anti-inflammatory effects of oxidized phospholipids. FASEB J. 2017;31(9):4187–202. doi:10.1096/fj.201601232RR. PMID:28572443.
- Ke Y, Zebda N, Oskokova O, Afonyushkin T, Berdyshev E, Tian Y, Meng F, Sarich N, Bochkov VN, Wang JM, et al. Anti-inflammatory effects of OxPAPC involve endothelial cell mediated generation of LXA4. Circ Res. 2017;121(3):244–57. doi:10.1161/CIRCRESAHA.116.310308. PMID:28522438.
- Cezar-de-Mello PF, Nascimento-Silva V, Villela CG, Fierro IM. Aspirin-triggered Lipoxin A4 inhibition of VEGF-induced endothelial cell migration involves actin polymerization and focal adhesion assembly. Oncogene. 2006;25:122–9. PMID:16132039.
- Vieira AM, Neto EH, Figueiredo CC, Barja Fidalgo C, Fierro IM, Morandi V. ATL-1, a synthetic analog of lipoxin, modulates endothelial permeability and interaction with tumor cells through a VEGF-dependent mechanism. Biochem Pharmacol. 2014;90:388–96. doi:10.1016/j.bcp.2014.05.019. PMID:24887589.
- Birukova AA, Lee S, Starosta V, Wu T, Ho T, Kim J, Berliner JA, Birukov KG. A role for VEGFR2 activation in endothelial responses caused by barrier disruptive OxPAPC concentrations. PLoS One. 2012;7:e30957. doi:10.1371/journal.pone.0030957. PMID:22303475.
- Birukova AA, Starosta V, Tian X, Higginbotham K, Koroniak L, Berliner JA, Birukov KG. Fragmented oxidation products define barrier disruptive endothelial cell response to OxPAPC. Transl Res. 2013;161:495–504. doi:10.1016/j.trsl.2012.12.008. PMID:23305708.
- Yeh M, Cole AL, Choi J, Liu Y, Tulchinsky D, Qiao JH, Fishbein MC, Dooley AN, Hovnanian T, Mouilleseaux K, et al. Role for sterol regulatory element-binding protein in activation of endothelial cells by phospholipid oxidation products. Circ Res. 2004;95:780–8. doi:10.1161/01.RES.0000146030.53089.18. PMID:15388640.
- Bochkov VN, Philippova M, Oskolkova O, Kadl A, Furnkranz A, Karabeg E, Afonyushkin T, Gruber F, Breuss J, Minchenko A, et al. Oxidized phospholipids stimulate angiogenesis via autocrine mechanisms, implicating a novel role for lipid oxidation in the evolution of atherosclerotic lesions. Circ Res. 2006;99:900–8. doi:10.1161/01.RES.0000245485.04489.ee. PMID:16973904.
- Oskolkova OV, Afonyushkin T, Leitner A, von Schlieffen E, Gargalovic PS, Lusis AJ, Binder BR, Bochkov VN. ATF4-dependent transcription is a key mechanism in VEGF up-regulation by oxidized phospholipids: critical role of oxidized sn-2 residues in activation of unfolded protein response. Blood. 2008;112:330–9. doi:10.1182/blood-2007-09-112870. PMID:18451308.
- Starosta V, Wu T, Zimman A, Pham D, Tian X, Oskolkova O, Bochkov V, Berliner JA, Birukova AA, Birukov KG. Differential regulation of endothelial cell permeability by high and low doses of oxidized 1-palmitoyl-2-arachidonyl-sn-glycero-3-phosphocholine. Am J Respir Cell Mol Biol. 2012;46:331–41. doi:10.1165/rcmb.2011-0153OC. PMID:21997484.
- Alcaide P, Newton G, Auerbach S, Sehrawat S, Mayadas TN, Golan DE, Yacono P, Vincent P, Kowalczyk A, Luscinskas FW. p120-Catenin regulates leukocyte transmigration through an effect on VE-cadherin phosphorylation. Blood. 2008;112:2770–9. doi:10.1182/blood-2008-03-147181. PMID:18641366.
- Potter MD, Barbero S, Cheresh DA. Tyrosine phosphorylation of VE-cadherin prevents binding of p120- and beta-catenin and maintains the cellular mesenchymal state. J Biol Chem. 2005;280:31906–12. doi:10.1074/jbc.M505568200. PMID:16027153.
- Allingham MJ, van Buul JD, Burridge K. ICAM-1-mediated, Src- and Pyk2-dependent vascular endothelial cadherin tyrosine phosphorylation is required for leukocyte transendothelial migration. J Immunol. 2007;179:4053–64. doi:10.4049/jimmunol.179.6.4053. PMID:17785844.
- Buland JR, Wasserloos KJ, Tyurin VA, Tyurina YY, Amoscato AA, Mallampalli RK, Chen BB, Zhao J, Zhao Y, Ofori-Acquah S, et al. Biosynthesis of oxidized lipid mediators via lipoprotein-associated phospholipase A2 hydrolysis of extracellular cardiolipin induces endothelial toxicity. Am J Physiol Lung Cell Mol Physiol. 2016;311:L303–16. PMID:27233995.
- Birukov KG. Oxidized lipids: the two faces of vascular inflammation. Curr Atheroscler Rep. 2006;8:223–31. doi:10.1007/s11883-006-0077-x. PMID:16640959.
- Heffern CT, Pocivavsek L, Birukova AA, Moldobaeva N, Bochkov VN, Lee KY, Birukov KG. Thermodynamic and kinetic investigations of the release of oxidized phospholipids from lipid membranes and its effect on vascular integrity. Chem Phys Lipids. 2013;175–176:9–19. doi:10.1016/j.chemphyslip.2013.07.003. PMID:23911706.
- Ozaki H, Hla T, Lee MJ. Sphingosine-1-phosphate signaling in endothelial activation. J Atheroscler Thromb. 2003;10:125–31. doi:10.5551/jat.10.125. PMID:14564080.
- Hla T. Signaling and biological actions of sphingosine 1-phosphate. Pharmacol Res. 2003;47:401–7. doi:10.1016/S1043-6618(03)00046-X. PMID:12676514.
- Abbasi T, Garcia JG. Sphingolipids in lung endothelial biology and regulation of vascular integrity. Handb Exp Pharmacol. 2013;216:201–26. doi:10.1007/978-3-7091-1511-4_10. PMID:23563658.
- Lo SK, Burhop KE, Kaplan JE, Malik AB. Role of platelets in maintenance of pulmonary vascular permeability to protein. Am J Physiol. 1988;254:H763–71. PMID:3354701.
- Haselton FR, Alexander JS. Platelets and a platelet-released factor enhance endothelial barrier. Am J Physiol. 1992;263:L670–8. PMID:1476205.
- Garcia JG, Liu F, Verin AD, Birukova A, Dechert MA, Gerthoffer WT, Bamberg JR, English D. Sphingosine 1-phosphate promotes endothelial cell barrier integrity by Edg-dependent cytoskeletal rearrangement. J Clin Invest. 2001;108:689–701. doi:10.1172/JCI12450. PMID:11544274.
- Takuwa Y. Subtype-specific differential regulation of Rho family G proteins and cell migration by the Edg family sphingosine-1-phosphate receptors. Biochimica Et Biophysica Acta. 2002;1582:112–20. doi:10.1016/S1388-1981(02)00145-2. PMID:12069818.
- McVerry BJ, Garcia JG. In vitro and in vivo modulation of vascular barrier integrity by sphingosine 1-phosphate: mechanistic insights. Cell Signal. 2005;17:131–9. doi:10.1016/j.cellsig.2004.08.006. PMID:15494205.
- Shikata Y, Birukov KG, Garcia JG. S1P induces FA remodeling in human pulmonary endothelial cells: role of Rac, GIT1, FAK, and paxillin. J Appl Physiol (1985). 2003;94:1193–203. doi:10.1152/japplphysiol.00690.2002. PMID:12482769.
- Li Q, Chen B, Zeng C, Fan A, Yuan Y, Guo X, Huang X, Huang Q. Differential activation of receptors and signal pathways upon stimulation by different doses of sphingosine-1-phosphate in endothelial cells. Exp Physiol. 2015;100:95–107. doi:10.1113/expphysiol.2014.082149. PMID:25557733.
- Galvani S, Sanson M, Blaho VA, Swendeman SL, Obinata H, Conger H, Dahlbäck B, Kono M, Proia RL, Smith JD, et al. HDL-bound sphingosine 1-phosphate acts as a biased agonist for the endothelial cell receptor S1P1 to limit vascular inflammation. Sci Signal. 2015;8:ra79. doi:10.1126/scisignal.aaa2581. PMID:26268607.
- Zhang G, Yang L, Kim GS, Ryan K, Lu S, O'Donnell RK, Spokes K, Shapiro N, Aird WC, Kluk MJ, et al. Critical role of sphingosine-1-phosphate receptor 2 (S1PR2) in acute vascular inflammation. Blood. 2013;122:443–55. doi:10.1182/blood-2012-11-467191. PMID:23723450.
- Nussbaum C, Bannenberg S, Keul P, Graler MH, Goncalves-de-Albuquerque CF, Korhonen H, von Wnuck Lipinski K, Heusch G, de Castro Faria Neto HC, Rohwedder I, et al. Sphingosine-1-phosphate receptor 3 promotes leukocyte rolling by mobilizing endothelial P-selectin. Nat Commun. 2015;6:6416. doi:10.1038/ncomms7416. PMID:25832730.
- Peng X, Hassoun PM, Sammani S, McVerry BJ, Burne MJ, Rabb H, Pearse D, Tuder RM, Garcia JG. Protective effects of sphingosine 1-phosphate in murine endotoxin-induced inflammatory lung injury. Am J Respir Crit Care Med. 2004;169:1245–51. doi:10.1164/rccm.200309-1258OC. PMID:15020292.
- McVerry BJ, Peng X, Hassoun PM, Sammani S, Simon BA, Garcia JG. Sphingosine 1-phosphate reduces vascular leak in murine and canine models of acute lung injury. Am J Respir Crit Care Med. 2004;170:987–93. doi:10.1164/rccm.200405-684OC. PMID:15282202.
- Sammani S, Moreno-Vinasco L, Mirzapoiazova T, Singleton PA, Chiang ET, Evenoski CL, Wang T, Mathew B, Husain A, Moitra J, et al. Differential effects of sphingosine 1-phosphate receptors on airway and vascular barrier function in the murine lung. Am J Respir Cell Mol Biol. 2010;43:394–402. doi:10.1165/rcmb.2009-0223OC. PMID:19749179.
- Winkler MS, Nierhaus A, Poppe A, Greiwe G, Graler MH, Daum G. Sphingosine-1-Phosphate: A potential biomarker and therapeutic target for endothelial dysfunction and sepsis? Shock (Augusta, Ga). 2017;47:666–72. doi:10.1097/SHK.0000000000000814. PMID:27922551.
- Dudek SM, Jacobson JR, Chiang ET, Birukov KG, Wang P, Zhan X, Garcia JG. Pulmonary endothelial cell barrier enhancement by sphingosine 1-phosphate: roles for cortactin and myosin light chain kinase. J Biol Chem. 2004;279:24692–700. doi:10.1074/jbc.M313969200. PMID:15056655.
- Birukov KG, Zebda N, Birukova AA. Barrier enhancing signals in pulmonary edema. Compr Physiol. 2013;3:429–84. PMID:23720293.
- Mehta D, Konstantoulaki M, Ahmmed GU, Malik AB. Sphingosine 1-phosphate-induced mobilization of intracellular Ca2+ mediates rac activation and adherens junction assembly in endothelial cells. J Biol Chem. 2005;280:17320–8. doi:10.1074/jbc.M411674200. PMID:15728185.
- Mandala S, Hajdu R, Bergstrom J, Quackenbush E, Xie J, Milligan J, Thornton R, Shei GJ, Card D, Keohane C, et al. Alteration of lymphocyte trafficking by sphingosine-1-phosphate receptor agonists. Science. 2002;296:346–9. doi:10.1126/science.1070238. PMID:11923495.
- Natarajan V, Dudek SM, Jacobson JR, Moreno-Vinasco L, Huang LS, Abassi T, Mathew B, Zhao Y, Wang L, Bittman R, et al. Sphingosine-1-phosphate, FTY720, and sphingosine-1-phosphate receptors in the pathobiology of acute lung injury. Am J Respir Cell Mol Biol. 2013;49:6–17. doi:10.1165/rcmb.2012-0411TR. PMID:23449739.
- Dudek SM, Camp SM, Chiang ET, Singleton PA, Usatyuk PV, Zhao Y, Natarajan V, Garcia JG. Pulmonary endothelial cell barrier enhancement by FTY720 does not require the S1P1 receptor. Cell Signal. 2007;19:1754–64. doi:10.1016/j.cellsig.2007.03.011. PMID:17475445.
- Frink M, Kaudel CP, Hildebrand F, Pape HC, Klempnauer J, Winkler M, Krettek C, van Griensven M. FTY720 improves survival after transient ischemia and reperfusion of the hind limbs. J Trauma. 2007;63:263–7. doi:10.1097/TA.0b013e3180d0a6fc. PMID:17693822.
- Liu HB, Cui NQ, Wang Q, Li DH, Xue XP. Sphingosine-1-phosphate and its analogue FTY720 diminish acute pulmonary injury in rats with acute necrotizing pancreatitis. Pancreas. 2008;36:e10–5. doi:10.1097/MPA.0b013e31815f3905. PMID:18362832.
- Farmer PJ, Bernier SG, Lepage A, Guillemette G, Regoli D, Sirois P. Permeability of endothelial monolayers to albumin is increased by bradykinin and inhibited by prostaglandins. Am J Physiol Lung Cell Mol Physiol. 2001;280:L732–8. PMID:11238014.
- Fukuhara S, Sakurai A, Sano H, Yamagishi A, Somekawa S, Takakura N, Saito Y, Kangawa K, Mochizuki N. Cyclic AMP potentiates vascular endothelial cadherin-mediated cell-cell contact to enhance endothelial barrier function through an Epac-Rap1 signaling pathway. Mol Cell Biol. 2005;25:136–46. doi:10.1128/MCB.25.1.136-146.2005. PMID:15601837.
- Park GY, Christman JW. Involvement of cyclooxygenase-2 and prostaglandins in the molecular pathogenesis of inflammatory lung diseases. Am J Physiol Lung Cell Mol Physiol. 2006;290:L797–805. doi:10.1152/ajplung.00513.2005. PMID:16603593.
- Kobayashi K, Tsubosaka Y, Hori M, Narumiya S, Ozaki H, Murata T. Prostaglandin D2-DP signaling promotes endothelial barrier function via the cAMP/PKA/Tiam1/Rac1 pathway. Arterioscler Thromb Vasc Biol. 2013;33:565–71. doi:10.1161/ATVBAHA.112.300993. PMID:23307871.
- Howard LS, Morrell NW. New therapeutic agents for pulmonary vascular disease. Paediatr Respir Rev. 2005;6:285–91. doi:10.1016/j.prrv.2005.09.006. PMID:16298312.
- Ueno Y, Koike H, Annoh S, Nishio S. Anti-inflammatory effects of beraprost sodium, a stable analogue of PGI2, and its mechanisms. Prostaglandins. 1997;53:279–89. doi:10.1016/S0090-6980(97)89601-3. PMID:9167214.
- Birukova AA, Fu P, Xing J, Birukov KG. Rap1 mediates protective effects of iloprost against ventilator-induced lung injury. J Appl Physiol (Bethesda, Md: 1985). 2009;107:1900–10. doi:10.1152/japplphysiol.00462.2009.
- Birukova AA, Fu P, Xing J, Cokic I, Birukov KG. Lung endothelial barrier protection by iloprost in the 2-hit models of ventilator-induced lung injury (VILI) involves inhibition of Rho signaling. Transl Res. 2010;155:44–54. doi:10.1016/j.trsl.2009.09.002. PMID:20004361.
- Birukova AA, Wu T, Tian Y, Meliton A, Sarich N, Tian X, Leff A, Birukov KG. Iloprost improves endothelial barrier function in lipopolysaccharide-induced lung injury. Eur Respir J. 2013;41:165–76. doi:10.1183/09031936.00148311. PMID:22790920.
- Langeler EG, van Hinsbergh VW. Norepinephrine and iloprost improve barrier function of human endothelial cell monolayers: role of cAMP. Am J Physiol. 1991;260:C1052–9. PMID:1709785.
- Birukova AA, Meng F, Tian Y, Meliton A, Sarich N, Quilliam LA, Birukov KG. Prostacyclin post-treatment improves LPS-induced acute lung injury and endothelial barrier recovery via Rap1. Biochimica Et Biophysica Acta. 2015;1852:778–91. doi:10.1016/j.bbadis.2014.12.016. PMID:25545047.
- Ke Y, Oskolkova O, Sarich N, Tian Y, Sitikov A, Tulapurkar M, Son S, Birukova AA, Birukov KG. Effects of prostaglandin lipid mediators on agonist-induced lung endothelial permeability and inflammation. Am J Physiol Lung Cell Mol Physiol. 2017;313(4):L710–L721. doi:10.1152/ajplung.00519.2016. PMID:28663336.
- Ohmura T, Tian Y, Sarich N, Ke Y, Meliton A, Shah AS, Andreasson K, Birukov KG, Birukova AA. Regulation of lung endothelial permeability and inflammatory responses by prostaglandin A2: role of EP4 receptor. Mol Biol Cell. 2017;28(12):1622–35. doi:10.1091/mbc.E16-09-0639. PMID:28428256.
- Moloney ED, Evans TW. Pathophysiology and pharmacological treatment of pulmonary hypertension in acute respiratory distress syndrome. Eur Respir J. 2003;21:720–7. doi:10.1183/09031936.03.00120102. PMID:12762363.
- Ali AE, Barrett JC, Eling TE. Prostaglandin and thromboxane production by fibroblasts and vascular endothelial cells. Prostaglandins. 1980;20:667–88. doi:10.1016/0090-6980(80)90107-0. PMID:7008090.
- Huang JS, Ramamurthy SK, Lin X, Le Breton GC. Cell signalling through thromboxane A2 receptors. Cell Signal. 2004;16:521–33. doi:10.1016/j.cellsig.2003.10.008. PMID:14751539.
- Klausner JM, Abu-Abid S, Alexander JS, Hanshke-Mineau R, Goldman G, Morel N, Valeri CR, Shepro D, Hechtman HB. Thromboxane modulates endothelial permeability. Mediators Inflamm. 1994;3:149–53. doi:10.1155/S0962935194000190. PMID:18472934.
- Kim SR, Bae SK, Park HJ, Kim MK, Kim K, Park SY, Jang HO, Yun I, Kim YJ, Yoo MA, et al. Thromboxane A(2) increases endothelial permeability through upregulation of interleukin-8. Biochem Biophys Res Commun. 2010;397:413–9. doi:10.1016/j.bbrc.2010.05.106. PMID:20510674.
- Deby-Dupont G, Braun M, Lamy M, Deby C, Pincemail J, Faymonville ME, Damas P, Bodson L, Lecart MP, Goutier R. Thromboxane and prostacyclin release in adult respiratory distress syndrome. Intensive Care Med. 1987;13:167–74. doi:10.1007/BF00254700. PMID:3584647.
- Goff CD, Corbin RS, Theiss SD, Frierson HF Jr, Cephas GA, Tribble CG, Kron IL, Young JS. Postinjury thromboxane receptor blockade ameliorates acute lung injury. Ann Thorac Surg. 1997;64:826–9. doi:10.1016/S0003-4975(97)00490-6. PMID:9307481.
- Zarbock A, Singbartl K, Ley K. Complete reversal of acid-induced acute lung injury by blocking of platelet-neutrophil aggregation. J Clin Invest. 2006;116:3211–9. doi:10.1172/JCI29499. PMID:17143330.
- Kobayashi K, Horikami D, Omori K, Nakamura T, Yamazaki A, Maeda S, Murata T. Thromboxane A2 exacerbates acute lung injury via promoting edema formation. Sci Rep. 2016;6:32109. doi:10.1038/srep32109. PMID:27562142.
- Zhao Z, Hu J, Gao X, Liang H, Yu H, Liu S, Liu Z. Hyperglycemia via activation of thromboxane A2 receptor impairs the integrity and function of blood-brain barrier in microvascular endothelial cells. Oncotarget. 2017;8:30030–8. PMID:28415790.
- Knezevic II, Predescu SA, Neamu RF, Gorovoy MS, Knezevic NM, Easington C, Malik AB, Predescu DN. Tiam1 and Rac1 are required for platelet-activating factor-induced endothelial junctional disassembly and increase in vascular permeability. J Biol Chem. 2009;284:5381–94. doi:10.1074/jbc.M808958200. PMID:19095647.
- Fang W, Geng X, Deng Y, Li Y, Shang E, Cen J, Lv P. Platelet activating factor induces blood brain barrier permeability alteration in vitro. J Neuroimmunol. 2011;230:42–7. doi:10.1016/j.jneuroim.2010.08.015. PMID:20870297.
- Meng F OT, Yang MM, Birukova A, Birukov K,. Esterification of iloprost enhances its barrier protective effects on pulmonary endothelium. Am J Respir Crit Care Med. 2017;195:A4779.
- McIntyre TM, Prescott SM, Stafforini DM. The emerging roles of PAF acetylhydrolase. J Lipid Res. 2009;50(Suppl):S255–9. doi:10.1194/jlr.R800024-JLR200. PMID:18838739.
- Latchoumycandane C, Marathe GK, Zhang R, McIntyre TM. Oxidatively truncated phospholipids are required agents of tumor necrosis factor alpha (TNFalpha)-induced apoptosis. J Biol Chem. 2012;287:17693–705. doi:10.1074/jbc.M111.300012. PMID:22433871.
- Deigner HP, Hermetter A. Oxidized phospholipids: emerging lipid mediators in pathophysiology. Curr Opin Lipidol. 2008;19:289–94. doi:10.1097/MOL.0b013e3282fe1d0e. PMID:18460921.
- Florens N, Calzada C, Lyasko E, Juillard L, Soulage CO. Modified lipids and lipoproteins in chronic kidney disease: A new class of uremic toxins. Toxins (Basel). 2016;8:E376. doi:10.3390/toxins8120376. PMID:27999257.
- Sharma S, Ruffenach G, Umar S, Motayagheni N, Reddy ST, Eghbali M. Role of oxidized lipids in pulmonary arterial hypertension. Pulm Circ. 2016;6:261–73. doi:10.1086/687293. PMID:27683603.
- Philippova M, Resink T, Erne P, Bochkov V. Oxidised phospholipids as biomarkers in human disease. Swiss Med Wkly. 2014;144:w14037. PMID:25539162.