ABSTRACT
Vitamin D deficiency has been linked to various inflammatory diseases in lungs, including pneumonia, asthma and chronic obstructive pulmonary disease. However, the mechanisms by which vitamin D and vitamin D receptor reduce inflammation in lung diseases remain poorly understood. In this study, we investigated the expression and cell-specific distribution of tight and adherens junctions in the lungs of vitamin D receptor-deficient (VDR-/-) mice. Our results demonstrated that mRNA and protein levels of claudin-2, claudin-4 and claudin-12 were significantly decreased in the lungs of VDR-/- mice. Other tight and adherens junction proteins, such as ZO-1, occludin, claudin-10, β-catenin, and VE-cadherin, showed significant differences in expression in the lungs of VDR-/- and wild-type mice. These data suggest that altered expression of tight and adherens junction molecules, especially of claudin-2, −4, −10, −12, and −18, after chronic pneumonia caused by VDR deletion could increase lung permeability.Therefore, VDR may play an important role in maintaining pulmonary barrier integrity. Further studies should confirm whether vitamin D/VDR is beneficial for the prevention or treatment of lung diseases.
Introduction
In recent years, vitamin D deficiency has been on the rise in the general population throughout the worldCitation1. Epidemiological studies have suggested that vitamin D deficiency is linked to an accelerated decline in lung function, increased inflammation and reduced immunity in susceptible populations, particularly in patients with asthma and chronic obstructive pulmonary disease (COPD)Citation2. Vitamin D deficiency and low levels of vitamin D receptor (VDR) are highly prevalent in patients with COPD, and the lowest vitamin D levels are associated with the most severe airflow obstructionCitation3. Moreover, VDR-deficient/knockout mice develop a COPD phenotype, and overexpression of VDR exerts anti-inflammatory effects in the lungs, implicating VDR in the pathogenesis of chronic lung diseasesCitation4,Citation5. Hence, understanding the molecular epigenetic mechanism of vitamin D/VDR will provide rationale for dietary vitamin D-mediated intervention in the prevention and management of chronic lung diseases. Vitamin D and VDR appear to regulate permeability barrier formationCitation6. However, the exact mechanisms of VDR underlying the pathogenesis of lung diseases are still largely unknown.
The permeability and integrity of the alveolar wall depend on the junctions between alveolar epithelial cells and capillary endothelial cells. Importantly, injury severity is directly related to the level of lung epithelial barrier dysfunctionCitation7. Adherens junctions (AJs) and tight junctions (TJs) are intercellular junctions that play a crucial role in the formation and maintenance of epithelial and endothelial barriersCitation8. AJs consist of the transmembrane proteins E-cadherin and VE-cadherin and intracellular components such as p120-catenin, β-catenin and α-catenin; these proteins control the formation, maintenance and function of AJs, which are positioned immediately below TJsCitation8,Citation9. TJs are the most apical structure of the apical complex demarcating the border between apical and basolateral membrane domains. TJs control fluid diffusion between cells by creating a barrier that specifically regulates paracellular permeability to ions and small molecules. The permeability of TJs in epithelia is a key factor in several pulmonary diseases. Alveolar barrier function depends critically on the claudins; therefore, small differences in alveolar epithelial barrier properties could have a large impact on disease susceptibility or outcome. Moreover, recent work has focused on the vital role of alterations in claudin expression in lung diseases, such as COPD, asthma, and acute respiratory distress syndrome (ARDS)Citation10.
Mice deficient in 25-hydroxyvitamin D1 α-hydroxylase, which is required for the formation of active vitamin D [1,25 dihydroxyvitamin D(1,25(OH)2D3)], have normal basal permeability barrier function but impaired epidermis barrier repair after acute disruption due to a decrease in lamellar body secretionCitation11. VDR-deficient mice also have defects in permeability barrier homeostasis due to a decrease in the number of lamellar bodiesCitation12. 1,25(OH)2D3 may play a protective role in mucosal barrier homeostasis by maintaining the integrity of junction complexes and in the healing capacity of the colon epitheliumCitation13. The role of VDR in the regulation of pulmonary barrier integrity in the chronic pneumonia observed in VDR-/- mice is unclear. To our knowledge, we have not found report about the expression patterns of tight junctions and adherens junctions in mice with chronic pneumonia induced by VDR deletion.
Here, we investigated alterations in the expression and cell-specific distribution of TJs and AJs in the lungs of VDR-/- mice. Our results demonstrated that the altered expression of TJ and AJ molecules in the absence of VDR could increase the permeability of alveolar walls. VDR may play an important role in maintaining pulmonary barrier integrity.
Materials and methods
Animals
All of the mice, including wild-type (WT) and VDR-deficient (VDR-/-) mice, were on a C57BL/6 background and housed in conventional animal facilities at Rush University. Experiments were performed on 4- to 6-month-old sex-matched (male and female) WT and VDR-/- mice of average body weight (24 ± 1.5 gm) were provided with water ad libitum and maintained on a 12-h dark/light cycle. All experimental protocols described in this study were approved by the animal research committee of Rush University.
Tight and adherens junction proteins detected by western blot
One-third of the left lung was harvested, cut into pieces with surgical scissors, lysed in lysis buffer, and sonicated, and the protein concentration was measured by the Bradford assay (Bio-Rad). The detailed method is described in our previous studyCitation14. All the antibodies were diluted to 1:1000, and primary antibodies () were used to detect VDR; TJ family members, including ZO-1, occludin, claudin-1, claudin-2, claudin-3, claudin-4, claudin-7, claudin-10, claudin-12 and claudin-15; and AJ family members such as α-catenin, β-catenin, E-cadherin and VE-cadherin. Bands were visualized by ECL. Protein quantitative analyses were completed by Quantity One software (Bio-Rad).
Table 1. Antibodies used in Western blotting and immunofluorescence staining.
Tight and adherens junction components detected by quantitative real-time PCR
Total RNA was extracted from one-third of the left lung using TRIzol® reagent (Invitrogen, Carlsbad, CA). The detailed method is described in our previous studyCitation15. RNA was reverse-transcribed by the iScript cDNA synthesis kit (Bio-Rad, Hercules, CA) according to the manufacturer’s directions. The RT reaction products (cDNAs) were subjected to quantitative real-time PCR using the MyiQ Single-Color Real-time PCR Detection System (Bio-Rad) and iQ SYBR Green Supermix (Bio-Rad). All expression levels were normalized to β-actin levels in the same sample. Relative expression was calculated as the ratio of the normalized value of each sample to that of the control sample. All real-time PCRs were performed in triplicate. PCR primers for TJ and AJ components, interleukin (IL)-1β and IL-6 were designed using Lasergene software (DNAStar, Madison, WI), and all the primers used are listed in .
Table 2. Real-time PCR primers used in this study.
Immunofluorescence and confocal imaging
Four-micrometer-thick sections were deparaffinized in xylene and rehydrated in a graded ethanol series. Antigen retrieval was performed in EDTA buffer (1 mM EDTA, 0.05% Tween 20, pH 8.0) at 95–98°C for 15 min in a microwave (Panasonic, 1250 W), followed by cooling for 30 min. After being incubated in 2% bovine serum albumin (BSA) (Sigma, St. Louis, MO, USA) and blocked for 1 h at RT, the sections were incubated with antibodies () at 37°C for 1 h and then at 4°C overnight. Sections were then washed three times with TBS-T (0.5% Tween, 0.1 M Tris-base, 0.9% NaCl, and pH 7.6) for 5 min each and incubated at 37°C for 60 min with goat anti-rabbit or anti-mouse IgG (1:100 dilution, Alexa Fluor® 594, Alexa Fluor® 488, Invitrogen, NY, USA) combined with DAPI (1:2000, Invitrogen, NY, USA). Negative control samples were prepared in parallel, but the primary antibody was replaced with TBS. Sections were rinsed three times with TBS-T for 5 min each and finally sealed with antifade reagent in glycerol (Invitrogen, NY, USA). The red or green signal was captured by a Carl Zeiss LSM 710 confocal microscope system. Colocalization of occludin and claudin-10 or claudin-4 was detected in VDR-/- and WT mouse lung tissues according to double immunofluorescence staining.
Histopathologic evaluation
One-third of the left lung was harvested, embedded in paraffin, stained with hematoxylin and eosin (H&E), observed and imaged by light microscopy with an Olympus CCD DP72. Slides were blinded for genotype of the mice and scored by two pathologists using a semiquantitative histology scoring in the range of 0 to 5Citation16. Zero indicated no inflammatory change, while 5 represented severe inflammation with tissue destruction.
Lung wet-to-dry weight ratio
After mice were euthanized, the diaphragmatic lobe of the right lung was excised and rapidly weighed to obtain the wet weight. Samples were dried in an oven (65°C) for 48 h to a stable dry lung weight. The wet-to-dry (W/D) lung weight ratio was calculated to assess lung tissue edema.
Bronchoalveolar lavage fluid collection and protein determination
An increased protein concentration in the BALF can reflect an increase in capillary leakage. Immediately after each mouse died, the left lung and the diaphragmatic lobe of the right lung were ligated, and the other lung lobes were washed by an intratracheal injection of 3 × 0.5 ml PBS, followed by gentle aspiration. The recovered fluid was processed for protein concentration analysis. Cells were removed by centrifugation (7,000 rpm for 10 min), and the cell-free supernatant was assayed for protein by the Bradford assay (Bio-Rad).
Statistical analysis
Data are presented as the mean ± SEM. Statistical analysis was performed using one-way ANOVA followed by Student’s t test. A p-value of 0.05 or less was considered statistically significant. All the data were analyzed using GraphPad Prism 5.
Results
Alterations in the expression of tight and adherens junction components in VDR-/- mice
TJs and AJs provide important adhesive contacts between neighboring epithelial cells. Proteins in TJs and AJs were detected by Western blot in WT and VDR-/- mouse lung tissues. The protein expressions of claudin-1, claudin-3, claudin-7, claudin-15 and α-catenin were normal in VDR-/- mouse lung tissues, whereas the protein expressions of other TJ proteins, ZO-1, occludin, claudin-2, claudin-4, and claudin-12, and AJ molecules, β-catenin and VE-cadherin, were significantly decreased in VDR-/- mouse lung tissues, compared to WT mouse lung tissues. However, claudin-10 protein levels were higher in VDR-/- mouse lung tissues than in WT mouse lung tissues (). Real-time PCR results showed that the mRNA expression of most TJ and AJ molecules was decreased in VDR-/- mouse lung tissues compared to WT mouse lung tissues. There were significant differences in claudin-2, claudin-4, claudin-10, claudin-12 and claudin-15 mRNA levels between VDR-/- and WT mouse lung tissues (). The mRNA levels of ZO-1 occludin were not affected by the VDR deletion. Simultaneously, we confirmed the expression level of VDR in our working system: VDR protein and mRNA were significantly decreased in VDR-/- mice compared to WT mice ( and ).
Figure 1. Alterations in tight and adherens junction proteins detected by Western blot TJ and AJ proteins such as ZO-1, occludin, claudin-2, claudin-4, claudin-12, β-catenin and VE-cadherin were significantly decreased in VDR-/- mice, but claudin-10 showed higher protein levels in VDR-/- mouse lung tissues than in WT mouse lung tissues (n = 3–5; * P < 0.05; ** P < 0.01).
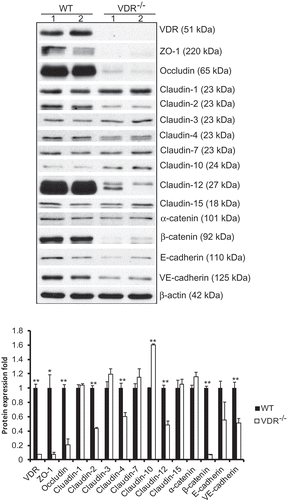
Figure 2. Alterations in tight and adherens junction mRNA expression detected by q-PCR. The mRNA expression of most TJ and AJ components showed a decreasing trend in VDR-/- mouse lung tissues. There were significant differences in claudin-2, claudin-4, claudin-10, claudin-12 and claudin-15 mRNA levels between VDR-/- and WT mouse lung tissues (n = 3–5; * P < 0.05; ** P < 0.01).
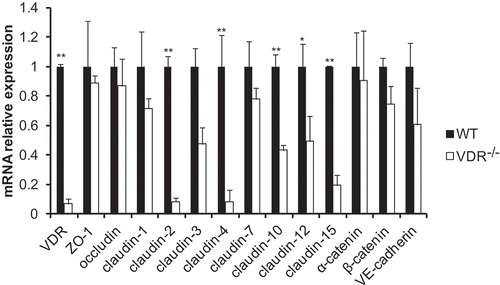
Specific distribution of tight and adherens junctions in mouse lung tissues
We detected VDR protein in the cytoplasm of bronchial epithelial cells only in WT mice; VDR-/- mice were nearly negative for VDR protein expression (). These data are consistent with the previous report that VDR is expressed in bronchial epithelial cells in the human, mouse and rat fetus but is absent in alveolar cells in the lungsCitation17.
Figure 3. Distribution of tight and adherens junction proteins in mouse lung tissues. VDR protein was located in the cytoplasm of bronchial epithelial cells in only WT mice, with nearly no expression in VDR-/- mice. ZO-1 and occludin were located at the cell membrane of alveolar epithelial cells and bronchial epithelium cells. Positive signals for claudin-2 was detected in only the cytoplasm of bronchial epithelium cells, and more positive signals were observed in WT mouse lung tissues. Claudin-3 and claudin-4 could be observed at the cell membrane of bronchial epithelium cells; moreover, claudin-4 levels were significantly decreased in VDR-/- mouse lung tissues. Claudin-10 protein expression was found at the cell membrane and in the cytoplasm of bronchial epithelium cells. Positive signals for claudin-12 were mostly located in the cytoplasm of bronchial epithelium cells, and stronger positive signals could be detected in lung tissues from WT mice than in that from VDR-/- mice. Positive signals for claudin-15 protein were detected in the cell membrane of mouse bronchial epithelium cells. AJs proteins, E-cadherin and β-catenin, had the same expression pattern, with primary localization at the cell membrane of alveolar epithelial cells and bronchial epithelium cells.
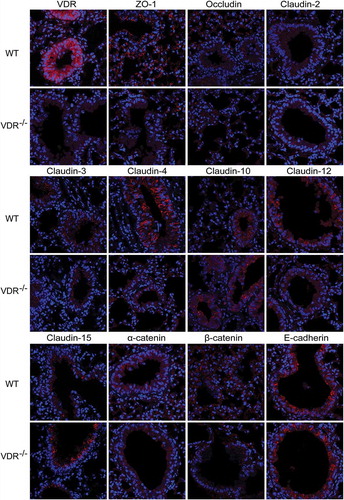
We used immunohistochemistry combined with confocal imaging to detect the specific distribution of TJ and AJ proteins in mouse lung tissues; all the positive signals are red. illustrates that ZO-1 and occludin were located at the cell membrane of alveolar epithelial cells and bronchial epithelium cells. Dot-like positive signals of claudin-2 were detected only in the cytoplasm of bronchial epithelium cells, and more claudin-2-positive cells in lung tissues were observed in WT mice than in VDR-/- mice. Claudin-3, −4 and −7 (data not shown) were observed at the cell membrane of bronchial epithelium cells; moreover, claudin-4 showed low levels of expression in VDR-/- mouse lung tissues. Claudin-10 protein expression was found at the cell membrane and in the cytoplasm of bronchial epithelium cells. Punctate positive signals of claudin-15 protein expression were detected in the cytoplasm and at the cell membrane of bronchial epithelium cells from VDR-/- mice, but were rare in WT mouse lung tissues. Three AJ proteins, E-cadherin, β-catenin and α-catenin, had the same expression pattern, with primary localization at the cell membrane of alveolar epithelial cells and bronchial epithelium cells. In , colocalization of occludin and claudin-10 was detected by double immunofluorescence staining in VDR-/- and WT mouse lung tissues.
Figure 4. Colocalization of occludin and claudin-10 in mouse lung tissues. Positive signals for claudin-10 protein were red, those for occludin protein were green, and colocalization of occludin with a claudin protein was yellow. Claudin-10 protein had higher expression in VDR-/- mouse lung tissues than in WT mouse lung tissues. On the contrary, occludin protein showed low levels of expression in the VDR-/- mouse lung tissues.
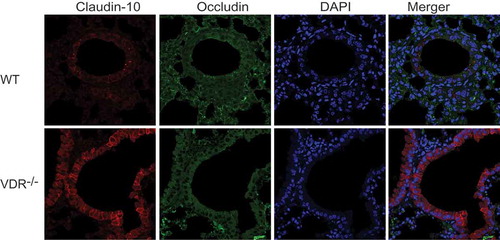
Claudin-18 plays a key role in alveolar barrier function. We further investigated the expression of claudin-18 in lung tissues with or without VDR. As shown in , the claudin-18 expression at the mRNA level tissues was significantly reduced in VDR-/- lung. The claudin-18 protein in lung tissues was detected by Western blot. We found the significant reduction of claudin-18 in the VDR-/- mice, compared to the WT mice. Localization of claudin-18 was detected by immunofluorescence staining in VDR-/- and WT mouse lung tissues. Claudin-18 was observed at the cell membrane of bronchial epithelium cells in mouse lungs. However, Claudin-18 was decreased in VDR-/- mice ().
Figure 5. The expression of claudin-18 in lung tissues with or without VDR. (A) Claudin-18 expression at the mRNA level tissues was significantly reduced in VDR-/- lung, tested by real-time PCR. (B) Claudin-18 protein in lung tissues was detected by Western blots. (C) Claudin-18 was observed at the cell membrane of bronchial epithelium cells in mouse lung tissues by Immunofluorescence staining. Claudin-18 was decreased in VDR-/- mice. (n = 3; * P < 0.05; ** P < 0.01).
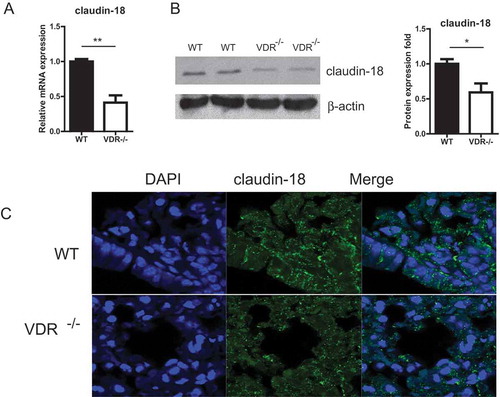
Chronic pneumonia and increased pulmonary permeability in VDR-/- mice
In our previous study, VDR-/- mice showed an emphysema/COPD phenotype, which makes these mice an appropriate in vivo model to study the pathogenesis of chronic lung diseasesCitation4, , shows the same pathological changes, including emphysema because of alveoli wall destruction, chronic inflammatory cell infiltration, lung septa with more than two times broadening, bronchial epithelium detachment, compared to the lungs of wild type mice. The semiquantitative histopathological scores were from two to four in the lungs of VDR-/- mice (). Moreover, the levels of IL−1β and IL−6 were significantly increased in VDR-/- mice compared with WT mice (data not shown). In interstitial lung disease, lung injury increases alveolar-capillary permeability. BALF total protein has been used to evaluate alveolar-capillary permeability in vivoCitation18, , and show the lung W/D ratio and the total protein concentration in the BALF. As expected, the values were significantly higher in VDR-/- mice than in WT mice.
Table 3. Histopathological scores of mice with wild type VDR and VDR-/-.
Figure 6. Histopathological alterations and increased lung permeability in VDR-/- mice. (A) Emphysema (star showed), chronic inflammatory cell infiltration (white arrowhead), lung septa more than two times broadening (green arrow with start and end) and bronchial epithelium detachment (green arrow) in VDR-/- mice lung tissues, compared with the wild type mice (magnification, × 400). (B) The lung wet-to-dry weight ratio. (C) Total protein concentration in the BALF (n = 3–5; * P < 0.05; ** P < 0.01).
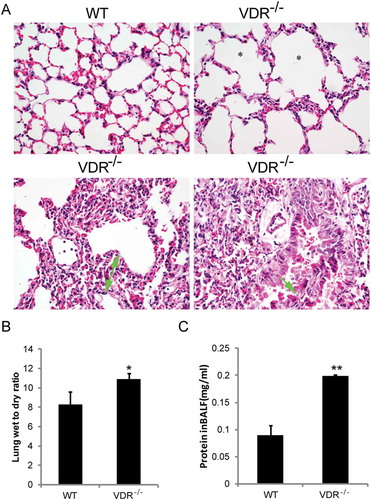
Discussion
In the current study, we reported that claudin-2, −4, 12, and −18 mRNA and protein levels were significantly decreased in the lungs of VDR-/- mice. We also report claudin-12 and claudin-15 protein distribution in mouse lung tissue. We discovered that positive signals for claudin-12 were mostly located in the cytoplasm of bronchial epithelium cells, with more positive cells in WT mouse lung tissues than in those from VDR-/- mice. Our findings indicated that altered expression of TJ and AJ components after chronic pneumonia caused by VDR deletion increases lung permeability. Therefore, VDR may play an important role in maintaining pulmonary barrier integrity.
Our data showed the altered expression of TJ and AJ components in VDR-/- mouse lung tissues and significant decreases in claudin−2, −4, −12, and −18 protein and mRNA levels; the changes in these four claudins closely correlate with airway epithelial barrier destruction in chronic pneumonia because of Vitamin D/VDR deficiency. Claudin−18 plays a key role in alveolar barrier function Citation19,Citation20–Citation22. Claudin-18 deficiency is associated with airway epithelial barrier dysfunction and asthmaCitation20. Our data suggested that claudin-18 was reduced at the mRNA level in the VDR-/- lungs. The regulatory mechanism of VDR on claudin-18 may be through the transcriptional level and lead to the changes of protein expression and distribution, thus affecting the barrier functions. Lower 25-hydroxyvitamin D (25[OH]D) levels are associated with a higher frequency of respiratory infections in healthy adults and more frequent asthma exacerbations in childrenCitation23. Moreover, asthma, COPD, interstitial lung disease and cystic fibrosis show correlations between severity and low vitamin D status, as do the incidences of pulmonary infections and lung cancerCitation24-Citation26. VDR deficiency in the mouse lung can lead to early-onset emphysema/COPD because of chronic inflammation, immune dysregulation, and lung destructionCitation4. Our data indicate that VDR deficiency invokes lung inflammation and alterations in lung function by destroying TJs and AJs. A decrease in claudin-2 protein and mRNA expression was observed in the gut epithelium of VDR-/- mice, compared with WT miceCitation27,Citation28. Previously, we have demonstrated that the claudin-2 gene is a direct target of VDRCitation28 in intestinal epithelial cells. 1,25(OH)2D3 can upregulate the expression of claudin-2 and claudin-12 through VDR in enterocytes in vitro and in vivo Citation29. However, during acute lung injury, the role of VDR regulation of lung claudin-2 is still unknown. Claudin-4 is known to increase, and the loss of claudin-4 decreases alveolar fluid clearance in mice; therefore, claudin-4 may represent a protective response in the alveolar epithelium that promotes alveolar fluid clearanceCitation30,Citation31. The above data suggested that claudin-4 may have different roles in different lung diseases, such as acute lung injury and chronic pneumonia. Simultaneously, ZO-1 and occludin proteins were significantly decreased in lung tissues from VDR-/- mice compared with those from WT mice, corresponding well with previously reported resultsCitation32. VDR knockout can also affect mouse corneal epithelium wound healing and tight junction integrityCitation33.
This report is the first on claudin-12 and claudin-15 protein distribution in mouse lung tissues. Airway TJs form a rate-limiting barrier to the penetration of inhaled pathogens into the interstitial compartment. Epithelia and endothelia display highly diverse tight junction barrier properties, likely indicative of the varied requirements for ion and solute regulation by tissue type. Airway TJs are regulated by claudin-claudin interactions that confer the selectivity of the junctionCitation34. claudin-12 and claudin-15 are both present in total lung at the mRNA levelCitation35, although at low levels in alveolar epithelial cells, and it is possible that they make important contributions to the alveolar epithelial barrierCitation36. We found that positive signals for claudin-12 were mostly located in the cytoplasm of bronchial epithelium cells, different from the other TJs (e.g. claudin-15), which were localized at the cell membrane of bronchial epithelium cells in VDR-/- mice. Claudin-15 is a pore forming claudins, just like claudin-2 Citation37. The studies of claudin-15 are still limited. We found that claudin-15 mRNA levels were significantly decreased in lungs of VDR-/- mice, but total claudin-15 protein levels were not changed. Immunoblotting of claudin-15 protein in total lung tissue may not reflect its changes in certain type of cells in lungs. Cell types in lungs might matter. Further studies are needed in a cell specific way.
The levels of claudin-3 and claudin-7 mRNA and proteins of lung tissues in VDR-/- mice showed no significant alteration, compared to those in wild type mice. Interestingly, decreases of claudin-3, claudin-7 and claudin-15 proteins in the small intestine have been detected in villous epithelial cells corresponding to the severity of histological changes, whereas leaving these claudin subspecies unchanged in crypt cellsCitation38. These results suggested that claudins have different biology function in the different cells or tissues. In our study, other TJ molecules such as ZO-1 and occludin were significantly reduced in VDR-/- mice, suggesting that chronic inflammation induced by VDR deletion may affect the barrier function of TJs and may correlate with the interaction between VDR and histone deacetylase 11 (HDAC11)Citation39.
Interestingly, In VDR-/- mouse lung tissues, claudin-10 was significantly decreased at the mRNA level but increased at the protein level, implying that claudin-10 mRNA and protein were not strictly linear. It may be subjected to different regulatory mechanisms. Claudin-10 exists in alternatively spliced isoforms that exhibit distinct localization and functionCitation40. It is known that two splice variants of claudin-10 (a and b) in the kidney create paracellular pores with different ion selectivities Citation41,Citation42. A recent study has shown that human claudin-10b N48K variant has pathogenic consequences, because it alters paracellular Na+ transport for sweat secretion, claudin oligomerization, TJ formation, and abundance of claudin-10b in the cell membraneCitation43. In the current study, we used claudin-10 polyclonal antibody (Catalog: 38–8400), a synthetic peptide derived from the C-terminus of the human/mouse claudin-10 protein. This antibody could only detect total claudin-10 protein. In addition, claudin-10 has been linked to lung adenocarcinomaCitation44. Claudin-10 is also regulated through IL10 in modulating aggressiveness of melanoma cells by B-1 lymphocytesCitation45. Which claudin-10 isoform regulated by VDR in lungs needs to be further investigated in the inflamed state.
AJ molecules, such as β-catenin and VE-cadherin, were significantly decreased at the protein level in VDR-/- mouse lung tissues compared to WT mouse lung tissues, indicating that airway AJs are also impaired by VDR deficiency. Cigarette smoke disrupts the integrity of airway AJs through the aberrant interaction of p120-catenin with the cytoplasmic tail of MUC1Citation46. In VDR-/- mouse lung tissues, we observed emphysema, chronic inflammatory cell infiltration, lung septa broadening and bronchial epithelium detachment. IL-1β and IL-6 increased significantly in VDR-/- mice compared with WT mice, suggesting the presence of chronic inflammatory lesions. Moreover, the lung W/D ratio and BALF total protein were found to be significantly higher in VDR-/- mice than in WT mice. These data suggested that VDR deficiency could increase lung permeability and disrupt the integrity of airway TJs and AJs, consistent with the above data.
The vitamin D pathway is an attractive target for intervention studies, particularly for COPD, because vitamin D deficiency may simultaneously enhance chronic airway and systemic inflammation, reduce bacterial clearance, and increase the risk for infectious exacerbations. VDR deficiency may cause defects in airway epithelial barrier function that contribute to a variety of lung diseases.
In summary, VDR deletion could evoke chronic pneumonia, which is associated with increased lung permeability, increased total protein in BALF, and alterations in the expression of TJ and AJ proteins, especially claudin-2, −4, −10, −12, and 18. These data indicate that VDR plays a vital role in maintaining pulmonary barrier integrity. Claudins play critical roles in health and disease of lungs Citation10,Citation47. Thus, the function and regulation of VDR on TJs and AJs may provide new therapeutic insights into lung diseases, e.g. acute lung injury, asthma, chronic pneumonia. pulmonary fibrosis Citation48 and lung cancer.
Authors’ contributions
JS secured research funding, designed the study, analyzed data, and finalized the manuscript; HLC and RL performed experiments and collected and analyzed data; HLC drafted the article; and YZ provided confocal imaging expertise and analyzed data. All authors were involved in writing the manuscript and reviewed and approved the final version.
Acknowledgments
This project was supported by the NIDDK R01DK105118-01 and R01DK114126 to JS. We thank Drs. Shaoping Wu and Dapeng Jin for their technical support.
Additional information
Funding
References
- Holick MF, Chen TC. Vitamin D deficiency: a worldwide problem with health consequences. Am J Clin Nutr. 2008;87(4):1080S–1086S. doi:10.1093/ajcn/87.4.1080S.
- Sundar IK, Rahman I. Vitamin d and susceptibility of chronic lung diseases: role of epigenetics. Front Pharmacol. 2011;2:50. doi:10.3389/fphar.2011.00050.
- Janssens W, Bouillon R, Claes B, Carremans C, Lehouck A, Buysschaert I, Coolen J, Mathieu C, Decramer M, Lambrechts D. Vitamin D deficiency is highly prevalent in COPD and correlates with variants in the vitamin D-binding gene. Thorax. 2010;65(3):215–220. doi:10.1136/thx.2009.120659.
- Sundar IK, Hwang JW, Wu S, Sun J, Rahman I. Deletion of vitamin D receptor leads to premature emphysema/COPD by increased matrix metalloproteinases and lymphoid aggregates formation. Biochem Biophys Res Commun. 2011;406(1):127–133. doi:10.1016/j.bbrc.2011.02.011.
- Ishii M, Yamaguchi Y, Isumi K, Ogawa S, Akishita M. Transgenic Mice Overexpressing Vitamin D Receptor (VDR) Show Anti-Inflammatory Effects in Lung Tissues. Inflammation. 2017;40(6):2012–2019. doi:10.1007/s10753-017-0641-2.
- Kong J, Zhang Z, Musch MW, Ning G, Sun J, Hart J, Bissonnette M, Li YC. Novel role of the vitamin D receptor in maintaining the integrity of the intestinal mucosal barrier. Am J Physiol Gastrointest Liver Physiol. 2008;294(1):G208–216. doi:10.1152/ajpgi.00398.2007.
- Ware LB, Matthay MA. Alveolar fluid clearance is impaired in the majority of patients with acute lung injury and the acute respiratory distress syndrome. Am J Respir Crit Care Med. 2001;163(6):1376–1383. doi:10.1164/ajrccm.163.6.2004035.
- Niessen CM. Tight junctions/adherens junctions: basic structure and function. J Invest Dermatol. 2007;127(11):2525–2532. doi:10.1038/sj.jid.5700865.
- Hartsock A, Nelson WJ. Adherens and tight junctions: structure, function and connections to the actin cytoskeleton. Biochim Biophys Acta. 2008;1778(3):660–669. doi:10.1016/j.bbamem.2007.07.012.
- Soini Y. Claudins in lung diseases. Respir Res. 2011;12:70. doi:10.1186/1465-9921-12-70.
- Bikle DD, Chang S, Crumrine D, Elalieh H, Man M-Q, Choi EH, Dardenne O, Xie Z, Arnaud RS, Feingold K, et al. 25 Hydroxyvitamin D 1 alpha-hydroxylase is required for optimal epidermal differentiation and permeability barrier homeostasis. J Invest Dermatol. 2004;122(4):984–992. doi:10.1111/j.0022-202X.2004.22424.x.
- Oda Y, Uchida Y, Moradian S, Crumrine D, Elias PM, Bikle DD. Vitamin D receptor and coactivators SRC2 and 3 regulate epidermis-specific sphingolipid production and permeability barrier formation. J Invest Dermatol. 2009;129(6):1367–1378. doi:10.1038/jid.2008.380.
- Zhao H, Zhang H, Wu H, Li H, Liu L, Guo J, Li C, Shih DQ, Zhang X. Protective role of 1,25(OH)2 vitamin D3 in the mucosal injury and epithelial barrier disruption in DSS-induced acute colitis in mice. BMC Gastroenterol. 2012;12:57. doi:10.1186/1471-230X-12-57.
- Liao AP, Petrof EO, Kuppireddi S, Zhao Y, Xia Y, Claud EC, Sun J, Ahmed N. Salmonella type III effector AvrA stabilizes cell tight junctions to inhibit inflammation in intestinal epithelial cells. PLoS One. 2008;3(6):e2369. doi:10.1371/journal.pone.0002369.
- Zhang YG, Wu S, Xia Y, Chen D, Petrof EO, Claud EC, Hsu W, Sun J, Heimesaat MM. Axin1 prevents Salmonella invasiveness and inflammatory response in intestinal epithelial cells. PLoS One. 2012;7(4):e34942. doi:10.1371/journal.pone.0034942.
- Davidson DJ, Dorin JR, McLachlan G, Ranaldi V, Lamb D, Doherty C, Govan J, Porteous DJ. Lung disease in the cystic fibrosis mouse exposed to bacterial pathogens. Nat Genet. 1995;9(4):351–357. doi:10.1038/ng0495-351.
- Wang Y, Zhu J, DeLuca HF. Where is the vitamin D receptor?. Arch Biochem Biophys. 2012;523(1):123–133. doi:10.1016/j.abb.2012.04.001.
- Inoue Y, Barker E, Daniloff E, Kohno N, Hiwada K, Newman LS. Pulmonary epithelial cell injury and alveolar-capillary permeability in berylliosis. Am J Respir Crit Care Med. 1997;156(1):109–115. doi:10.1164/ajrccm.156.1.9612043.
- Zhou B, Flodby P, Luo J, Castillo DR, Liu Y, Yu F-X, McConnell A, Varghese B, Li G, Chimge N-O, et al. Claudin-18-mediated YAP activity regulates lung stem and progenitor cell homeostasis and tumorigenesis. J Clin Invest. 2018;128(3):970–984. doi:10.1172/JCI90429.
- Sweerus K, Lachowicz-Scroggins M, Gordon E, LaFemina M, Huang X, Parikh M, Kanegai C, Fahy JV, Frank JA. Claudin-18 deficiency is associated with airway epithelial barrier dysfunction and asthma. J Allergy Clin Immunol. 2017;139(1):72–81. e71. doi:10.1016/j.jaci.2016.02.035.
- Li G, Flodby P, Luo J, Kage H, Sipos A, Gao D, Ji Y, Beard LL, Marconett CN, DeMaio L, et al. Knockout mice reveal key roles for claudin 18 in alveolar barrier properties and fluid homeostasis. Am J Respir Cell Mol Biol. 2014;51(2):210–22. doi:10.1165/rcmb.2013-0353OC .
- LaFemina MJ, Sutherland KM, Bentley T, Gonzales LW, Allen L, Chapin CJ, Rokkam D, Sweerus KA, Dobbs LG, Ballard PL, et al. Claudin-18 deficiency results in alveolar barrier dysfunction and impaired alveologenesis in mice. Am J Respir Cell Mol Biol. 2014;51(4):550–558. doi:10.1165/rcmb.2013-0456OC.
- Brehm JM, Schuemann B, Fuhlbrigge AL, Hollis BW, Strunk RC, Zeiger RS, Weiss ST, Litonjua AA. Serum vitamin D levels and severe asthma exacerbations in the Childhood Asthma Management Program study. J Allergy Clin Immunol. 2010;126(1):52–58. e55. doi:10.1016/j.jaci.2010.03.043.
- Finklea JD, Grossmann RE, Tangpricha V. Vitamin D and chronic lung disease: a review of molecular mechanisms and clinical studies. Adv Nutr. 2011;2(3):244–253. doi:10.3945/an.111.000398.
- Gromowski T, Gapska P, Scott RJ, Kąklewski K, Marciniak W, Durda K, Lener M, Górski B, Cybulski C, Sukiennicki G, et al. Serum 25(OH)D concentration, common variants of the VDR gene and lung cancer occurrence. Int J Cancer. 2017;141(2):336–341. doi:10.1002/ijc.30740.
- Bar-Yoseph R, Bentur L, Goldbart A, Livnat G, Hakim F, Weisman Y, Tiosano D. A mutated vitamin D receptor in hereditary vitamin D-resistant rickets prevents induction of bronchial hyperreactivity and inflammation. J Clin Endocrinol Metab. 2014;99(9):E1610–1616. doi:10.1210/jc.2014-1396.
- Kuhne H, Hause G, Grundmann SM, Schutkowski A, Brandsch C, Stangl GI. Vitamin D receptor knockout mice exhibit elongated intestinal microvilli and increased ezrin expression. Nutr Res. 2016;36(2):184–192. doi:10.1016/j.nutres.2015.10.005.
- Zhang YG, Wu S, Lu R, Zhou D, Zhou J, Carmeliet G, Petrof E, Claud EC, Sun J. Tight junction CLDN2 gene is a direct target of the vitamin D receptor. Sci Rep. 2015;5:10642. doi:10.1038/srep10642.
- Fujita H, Sugimoto K, Inatomi S, Maeda T, Osanai M, Uchiyama Y, Yamamoto Y, Wada T, Kojima T, Yokozaki H, et al. Tight junction proteins claudin-2 and −12 are critical for vitamin D-dependent Ca2+ absorption between enterocytes. Mol Biol Cell. 2008;19(5):1912–1921. doi:10.1091/mbc.e07-09-0973.
- Rokkam D, Lafemina MJ, Lee JW, Matthay MA, Frank JA. Claudin-4 levels are associated with intact alveolar fluid clearance in human lungs. Am J Pathol. 2011;179(3):1081–1087. doi:10.1016/j.ajpath.2011.05.017.
- Wray C, Mao Y, Pan J, Chandrasena A, Piasta F, Frank JA. Claudin-4 augments alveolar epithelial barrier function and is induced in acute lung injury. Am J Physiol Lung Cell Mol Physiol. 2009;297(2):L219–227. doi:10.1152/ajplung.00043.2009.
- Shi YY, Liu TJ, Fu JH, Xu W, Wu -L-L, Hou A-N, Xue X-D. Vitamin D/VDR signaling attenuates lipopolysaccharideinduced acute lung injury by maintaining the integrity of the pulmonary epithelial barrier. Mol Med Rep. 2016;13(2):1186–1194. doi:10.3892/mmr.2015.4685.
- Elizondo RA, Yin Z, Lu X, Watsky MA. Effect of vitamin D receptor knockout on cornea epithelium wound healing and tight junctions. Invest Ophthalmol Vis Sci. 2014;55(8):5245–5251. doi:10.1167/iovs.13-13553.
- Coyne CB, Gambling TM, Boucher RC, Carson JL, Johnson LG, Coyne CB, Gambling TM, Boucher RC, Carson JL, Johnson LG. Role of claudin interactions in airway tight junctional permeability. Am J Physiol Lung Cell Mol Physiol. 2003;285(5):L1166–1178. doi:10.1152/ajplung.00182.2003.
- Ohta H, Chiba S, Ebina M, Furuse M, Nukiwa T. Altered expression of tight junction molecules in alveolar septa in lung injury and fibrosis. Am J Physiol Lung Cell Mol Physiol. 2012;302(2):L193–205. doi:10.1152/ajplung.00349.2010.
- LaFemina MJ, Rokkam D, Chandrasena A, Pan J, Bajaj A, Johnson M, Frank JA. Keratinocyte growth factor enhances barrier function without altering claudin expression in primary alveolar epithelial cells. Am J Physiol Lung Cell Mol Physiol. 2010;299(6):L724–734. doi:10.1152/ajplung.00233.2010.
- Lechuga S, Ivanov AI. Disruption of the epithelial barrier during intestinal inflammation: quest for new molecules and mechanisms. Biochim Biophys Acta. 2017;1864(7):1183–1194. doi:10.1016/j.bbamcr.2017.03.007.
- Abiko Y, Kojima T, Murata M, Tsujiwaki M, Takeuchi M, Sawada N, Mori M. Changes of Tight Junction Protein Claudins in Small Intestine and Kidney Tissues of Mice Fed a DDC Diet. J Toxicol Pathol. 2013;26(4):433–438. doi:10.1293/tox.2013-0009.
- Liu FH, Li SS, Li XX, Wang S, Li MG, Guan L, Luan TG, Liu ZG, Liu ZJ, Yang PC. Vitamin D3 induces vitamin D receptor and HDAC11 binding to relieve the promoter of the tight junction proteins. Oncotarget. 2017;8(35):58781–58789. doi: 10.18632/oncotarget.17692.
- Gunzel D, Stuiver M, Kausalya PJ, Haisch L, Krug SM, Rosenthal R, Meij IC, Hunziker W, Fromm M, Muller D. Claudin-10 exists in six alternatively spliced isoforms that exhibit distinct localization and function. J Cell Sci. 2009;122(Pt 10):1507–1517. doi:10.1242/jcs.040113.
- Milatz S, Piontek J, Hempel C, Meoli L, Grohe C, Fromm A, Lee I-FM, El-Athman R, Günzel D. Tight junction strand formation by claudin-10 isoforms and claudin-10a/-10b chimeras. Ann N Y Acad Sci. 2017;1405(1):102–115. doi:10.1111/nyas.13393.
- Van Itallie CM, Rogan S, Yu A, Vidal LS, Holmes J, Anderson JM. Two splice variants of claudin-10 in the kidney create paracellular pores with different ion selectivities. Am J Physiol Renal Physiol. 2006;291(6):F1288–1299. doi:10.1152/ajprenal.00138.2006.
- Klar J, Piontek J, Milatz S, Tariq M, Jameel M, Breiderhoff T, Schuster J, Fatima A, Asif M, Sher M, et al. Altered paracellular cation permeability due to a rare CLDN10B variant causes anhidrosis and kidney damage. PLoS Genet. 2017;13(7):e1006897. doi:10.1371/journal.pgen.1006897.
- Zhang Z, Wang A, Sun B, Zhan Z, Chen K, Wang C. Expression of CLDN1 and CLDN10 in lung adenocarcinoma in situ and invasive lepidic predominant adenocarcinoma. J Cardiothorac Surg. 2013;8:95. doi:10.1186/1749-8090-8-95.
- Perez EC, Xander P, Laurindo MFL, Novaes E Brito RR, Vivanco BC, Mortara RA, Mariano M, Lopes JD, Keller AC, Facchiano A. The axis IL-10/claudin-10 is implicated in the modulation of aggressiveness of melanoma cells by B-1 lymphocytes. PLoS One. 2017;12(11):e0187333. doi:10.1371/journal.pone.0187333.
- Zhang L, Gallup M, Zlock L, Basbaum C, Finkbeiner WE, McNamara NA. Cigarette smoke disrupts the integrity of airway adherens junctions through the aberrant interaction of p120-catenin with the cytoplasmic tail of MUC1. J Pathol. 2013;229(1):74–86. doi:10.1002/path.2013.229.issue-1.
- Baumholtz AI, Gupta IR, Ryan AK. Claudins in morphogenesis: forming an epithelial tube. Tissue Barriers. 2017;5(4):e1361899. doi:10.1080/21688370.2017.1361899.
- Frank JA. Claudins and alveolar epithelial barrier function in the lung. Ann N Y Acad Sci. 2012;1257:175–183. doi:10.1111/j.1749-6632.2012.06533.x.